Neurodevelopmental disorders are disturbances of the central nervous system's architecture, processes, and maturation that affect a person's behavior (e.g., social-communication and interaction skills, language, attention, emotions, motor performance, learning, memory) and significantly reduce functional adaptive skills in everyday life. The DSM-5 (APA, 2013) acknowledges the significance of aberrant neurodevelopment for psychiatry by introducing the umbrella concept of neurodevelopmental disorders for the first time (Regier et al., Reference Regier, Narrow, Kuhl and Kupfer2009). No good prevalence estimates are available for neurodevelopmental disorders in total, but preliminary survey data from the United States indicates a frequency of 12% in the general population (Pastor & Reuben, Reference Pastor and Reuben2009). Currently, increasingly accepted estimates for two of the most prominent neurodevelopmental disorders, autism spectrum disorder (ASD) and attention-deficit hyperactivity disorder (ADHD), in school-aged children are ~1–2% (Idring et al., Reference Idring, Rai, Dal, Dalman, Sturm, Zander and Magnusson2012; Kim et al., Reference Kim, Leventhal, Koh, Fombonne, Laska, Lim and Grinker2011) and 5% (Polanczyk et al., Reference Polanczyk, de Lima, Horta, Biederman and Rohde2007), respectively. Males are affected 2–4 times more often than females. ASD and ADHD have been increasingly diagnosed in the last decade (Froehlich et al., Reference Froehlich, Lamphear, Epstein, Barbaresi, Katusic and Kahn2007; Rutter, Reference Rutter2005). Although still debated, improved knowledge, increased awareness, and better services, as well as reduced stigmatization, changes in and a more sensitive interpretation of diagnostic criteria probably account for a significant proportion of these time trends (Wazana et al., Reference Wazana, Bresnahan and Kline2007). Comorbidity is high in ASD and ADHD individuals. For example, the overlap of ASD and intellectual developmental disorders is in the range of 50% (Fombonne, Reference Fombonne2009). Simonoff et al. (Reference Simonoff, Pickles, Charman, Chandler, Loucas and Baird2008) found that ~28% of school-aged children with ASD also fulfill DSM-IV criteria for ADHD and 9% for chronic tic disorder. A meta-analysis of ADHD yielded a 10.7-fold risk for conduct disorder, 5.5-fold for depression, and 3.0-fold for anxiety disorder (Angold et al., Reference Angold, Costello and Erkanli1999).
ASD and ADHD have many causes, but most evidence suggest that they are highly heritable and show modest non-shared environmental influence, that is, environmental influences that make children growing up in the same family different (Chang et al., Reference Chang, Lichtenstein, Asherson and Larsson2013; Lichtenstein et al., Reference Lichtenstein, Carlström, Råstam, Gillberg and Anckarsäter2010). Aside from clinically relevant phenotypes qualifying for a categorical diagnosis, data increasingly support the notion of broader/extended phenotypes and traits of ASD and ADHD that are continuously distributed in the general population (Constantino & Todd, Reference Constantino and Todd2003; Normand et al., Reference Normand, Flora, Toplak and Tannock2012), with their variation determined by factors overlapping with clinical phenotypes (Robinson et al., Reference Robinson, Koenen, McCormick, Munir, Hallett, Happé, Plomin and Ronald2011; Larsson et al., Reference Larsson, Anckarsäter, Råstam, Chang and Lichtenstein2012; Lundström et al., Reference Lundström, Chang, Råstam, Gillberg, Larsson, Anckarsäter and Lichtenstein2012). Heritability estimates, predominantly from twin research, indicate that genetic factors account for ~80% of symptom variation in ASD and ADHD (Ronald & Hoekstra, Reference Ronald and Hoekstra2011; Taylor et al., Reference Taylor, Charman, Robinson, Plomin, Happe, Asherson and Ronald2013; Wadsworth et al., Reference Wadsworth, DeFries, Olson and Willcut2007; Wood & Neale, Reference Wood and Neale2010), as well as ASD-like and ADHD-like traits (e.g., Constantino et al., Reference Constantino and Todd2003). In addition, behavior and molecular studies indicate partly overlapping genetic components for both disorders (e.g., Heiser et al., Reference Heiser, Friedel, Dempfle, Konrad, Smidt, Grabarkiewicz and Hebebrand2004; Moreno-De-Luca et al., Reference Moreno-De-Luca, Myers, Challman, Moreno-De-Luca, Evans and Ledbetter2013; Reiersen et al., Reference Reiersen, Constantino, Grimmer, Martin and Todd2008; Ronald et al., Reference Ronald, Simonoff, Kuntsi, Asherson and Plomin2008; Taylor et al., Reference Taylor, Charman, Robinson, Plomin, Happe, Asherson and Ronald2013).
The genetics of ASD and ADHD are complex. In 10% of individuals with ASD an identifiable single-gene disorder, such as fragile X, tuberous sclerosis or neurofibromatosis exists, which may account for the phenotype. In addition, 5–10% have known chromosomal rearrangements such as maternally inherited duplications of 15q11-q13, inherited or non-inherited (de novo) copy number variations (CNVs), predominantly in genes with synaptic functions (e.g., NLGN3, NLGN4, NEUREXIN1, SHANK3, and CNTNAP2; Persico & Napolioni, Reference Persico and Napolioni2013). These findings, coupled with genome sequencing data, suggest the existence of hundreds of ASD risk genes (Devlin & Scherer, Reference Devlin and Scherer2012). To date, ADHD studies have reported substantial evidence implicating several genes, including those in the dopaminergic (DRD4, DAT1, DRD5, COMT), noradrenergic (DBH, ADRA2A), serotonergic (5-HTT, HTR1B, HTR2A), cholinergic (CHRNA4), and central nervous system development (SNAP25, BDNF) pathways (Caylak, Reference Caylak2012).
Even though ASD and ADHD are highly genetic, the causes are multifactorial, and environmental influences also play an important role in susceptibility, with non-shared environment consistently reported in twin studies of ASD, ADHD, and their related traits (Ronald & Hoekstra, Reference Ronald and Hoekstra2011). Environmental factors may act via epigenetic mechanisms, that is, modifications of gene expression controlled by something other than the DNA sequence that are potentially reversible. These factors are influenced by the maturational stage, tissue type, and environment. Evidence suggests that dysregulation of epigenetic markers or mechanisms, such as DNA methylation, plays an important role in ASD and ADHD and integrates genetic and environmental influences to dysregulate neurodevelopmental processes (Grafodatskaya et al., Reference Grafodatskaya, Chung, Szatmari and Weksberg2010; Smith et al., Reference Smith, Mick and Faraone2009). Environmental factors may play a role in the inception and lifelong modulation of ASD (Herbert, Reference Herbert2010). Some gene variants in ASD confer altered vulnerability to environmental stressors and exposures (Levy et al., Reference Levy, Mandell and Schultz2009). Random de novo mutations increasing with advanced parental age are stochastic risk factors for ASD, suggesting a role of the environment (Parner et al., Reference Parner, Baron-Cohen, Lauritsen, Jorgensen, Schieve, Yeargin-Allsopp and Obel2012). The systemic and central nervous system pathophysiology in ASD, including oxidative stress, neuroinflammation, and mitochondrial dysfunction, can be consistent with environmental influence (e.g., air pollution, organophosphates, or heavy metals; Chauhan & Chauhan, Reference Chauhan and Chauhan2006; Jung et al., Reference Jung, Lin and Hwang2013) and some of the underlying biochemical disturbances (e.g., abnormalities in glutathione, a critical antioxidant and detoxifier, might be reversed by targeted nutritional interventions; Geraghty et al., Reference Geraghty, Bates-Wall, Ratliff-Schaub and Lane2010). Dietary factors and food contaminants may contribute to the risk. Similarly, the environmental risk factors for ADHD include prenatal exposure to substances, exposure to heavy metals and chemicals, nutritional factors, and lifestyle/psychosocial factors (Froehlich et al., Reference Froehlich, Anixt, Loe, Chirdkiatgumchai, Kuan and Gilman2011).
As indicated by the designation ‘neurodevelopmental disorder’, genetic, epigenetic, and environmental factors detrimentally affect neurobiological and neurocognitive development and maturation. In ASD, macrocephaly is noted by age 12 to 24 months in a substantial proportion of children (Courchesne et al., Reference Courchesne, Campbell and Solso2011). Structural neuroimaging studies demonstrate alterations in both cortical and white matter regions, together with abnormal patterns of growth mainly localized in the frontal lobe, temporal lobes, and limbic structures, such as the amygdala. Diffusion tensor imaging has demonstrated the disruption of white matter fiber bundle connecting brain regions associated with various high-level cognitive functions (e.g., social cognition, language). Functional neuroimaging studies have found alterations in activation and synchrony across cortical networks, with reduced functional connectivity relating to a variety of cognitive functions, including language, working memory, social cognition or perception, and problem solving (Vissers et al., Reference Vissers, Cohen and Geurts2012). Neurochemical investigations with animal models and empirical drug studies remain inconclusive. Genetic differences in serotonin transport seem to have the most empirical evidence for a role in ASD, whereas data lending support to a role of the dopaminergic and glutaminergic systems are presently less robust, but evolving (Geschwind & Levitt, Reference Geschwind and Lewitt2007).
Taken together, the results thus far indicate that ASD is characterized by a neuronal-cortical organization impacting on developmental trajectory of social cognition (including social attention and social orientation; Senju, Reference Senju2013), executive function, and problem-solving mindset (Levy et al., Reference Levy, Mandell and Schultz2009), and top-down versus bottom-up information processing with the integration of information into meaningful gestalt (Bölte et al., Reference Bölte, Holtmann, Poustka, Scheurich and Schmidt2007; Dziobek & Bölte, Reference Dziobek and Bölte2011). These findings tie in with behavior genetic research that suggests that the triad of impairments that characterize ASD may have partly different causal pathways (e.g., Happé & Ronald, Reference Happé and Ronald2008; Ronald et al., Reference Ronald, Larsson, Anckarsäter and Lichtenstein2011). Neurobiological alterations are widespread in ADHD, with the most significant and consistent structural imaging findings pointing to smaller total brain volumes and reduced volumes in the right frontal lobe, right parietal cortex, caudate nucleus, cerebellar hemispheres, and posterior-inferior lobules of the cerebellar vermis (Tripp & Wickens, Reference Tripp and Wickens2009). Neurochemically, ADHD involves hypofunctioning of catecholaminergic circuits, particularly those that project to the prefrontal cortex (Durston, Reference Durston2008). On the cognitive level, a triple pathway model of ADHD is favored, with impairment in executive control/inhibition, motivation (delay-aversion), and timing as dissociable neuropsychological components (Sonuga-Barke et al., Reference Sonuga-Barke, Bitsakou and Thompson2010).
As described above, the understanding of the potential causes of neurodevelopmental disorders, most notably ASD and ADHD, has significantly increased. Still, knowledge regarding their etiology and pathophysiology is far from complete. The genetic, epigenetic, and environmental mechanisms driving neurodevelopmental phenotypes remain poorly understood. Outcome variability, evidence of a treatment response to a variety of interventions, and the possibility of improvement in ASD and ADHD suggest that the brain circuitry is amenable to epigenetic/environmental modulation. Thus, targets need to be sought for early biopsychosocial prevention and treatment. However, ASD and ADHD research so far has several limitations. First, most studies are based on cases versus typical controls only, which does not allow conclusions about causality. Second, many studies are one-dimensional, focusing on the associations between narrow aspects of psychopathology and (epi-)genetics, environment, neurobiology, or neuropsychology. Third, studies have mostly looked at behavioral ASD and ADHD phenotypes categorically (as clinical diagnoses), rather than dimensionally (as traits), and almost never jointly. Fourth, DSM-IV and ICD-10 include mutual diagnostic exclusion criteria for ASD and ADHD, which hampered appropriate research on conditions with high comorbidity.
With this project, we seek to overcome many of these shortcomings in order to generate a launch pad for pioneering, novel evidence in understanding the complexity of ASD and ADHD. For this purpose, the largest cohort ever of monozygotic (MZ) twins discordant or concordant for carefully characterized clinical and subthreshold ASD and ADHD phenotypes, as well as typically developed controls, will be investigated in concert with multidimensional, simultaneous assessments and combined analytical approaches, comprising medical history, comorbidity, neuropsychology, dysmorphology, collection of neuroepithelial stem (NES) cells and specimens for (epi-)genetic, neurotoxic, gut bacteria, protein-/monoamine, electron microscopy analyses, and multimodal neuroimaging (e.g., structural, functional, and molecular investigations). We hypothesize subtle, but specific differences within discordant monozygotic ASD and ADHD pairs on multiple biological and behavioral levels of assessment that will be informative for deciphering the complexity of genotype-environment-phenotype correlations, especially those related to non-shared environment.
Materials and Methods
Design
Roots of Autism and ADHD Twin Study in Sweden (RATSS) uses the co-twin control or twin-differences design (Martin et al., Reference Martin, Boomsma and Machin1997). Only a moderate number of studies have specifically addressed discordant MZ twin pairs for ASD (e.g., Hu et al., Reference Hu, Frank, Heine, Lee and Quackenbush2006; Kates et al., Reference Kates, Mostofsky, Zimmerman, Mazzocco, Landa, Warsofsky and Reiss1998, Reference Kates, Burnette, Eliez, Strunge, Kaplan, Landa and Pearlson2004, Reference Kates, Ikuta and Burnette2009; Mitchell et al., Reference Mitchell, Reiss, Tatusko, Ikuta, Kazmerski, Botti and Kates2009; Wong et al., in press), ADHD (e.g., Castellanos et al., Reference Castellanos, Sharp, Gottesman, Greenstein, Giedd and Rapoport2003; Lehn et al., Reference Lehn, Derks, Hudziak, Heutink, van Beijsterveldt and Boomsma2007; Pearsall-Jones et al., Reference Pearsall-Jones, Piek, Martin, Rigoli, Levy and Hay2008, Reference Pearsall-Jones, Piek, Rigoli, Martin and Levy2009; Sharp et al., Reference Sharp, Gottesman, Greenstein, Ebens, Rapoport and Castellanos2003; van't Ent et al., Reference Van't Ent, van Beijsterveldt, Derks, Hudziak, Veltman, Todd and De Geus2009) or traits of both disorders (e.g., Groen-Blokhuis et al., Reference Groen-Blokhuis, Middeldorp, van Beijsterveldt and Boomsma2011; Ronald et al., Reference Ronald, Happé, Dworzynski, Bolton and Plomin2010). The findings have been promising, indicating medical history (e.g., ADHD: low birth weight and delayed physical growth/motor development in cases), neuromorphological differences (e.g., ASD: reduced caudate, amygdala, hippocampus, and cerebellar volumes, differences in cortical folding within the partial lobe; ADHD: reduced caudate nucleus and prefrontal lobe volumes in cases), and differential gene expression patterns on DNA microarrays (e.g., ASD: ASS, NAGLU, FLAP, and ROBO I) in the affected twin. The current project will elaborate upon these works using an integrated, comprehensive assessment approach in a significantly larger cohort, providing the option of cross-disciplinary analyses.
In RATSS, we predict that the affected co-twins, compared to the unaffected co-twins, in MZ pairs discordant for ASD and ADHD will exhibit de novo mutations, such as single nucleotide variations (SNVs) or CNVs in genes crucial for neurodevelopment, differences in methylation at selected genes or overall epigenome, and environmental factors as a possible source of epigenetic and phenotypic variation. In addition, we expect these differences to be associated with functional and structural neurobiological characteristics, particularly over- and under-connectivity in ASD, and eventually in ADHD (Konrad & Eickhoff, Reference Konrad and Eickhoff2010), as well as with molecular brain characteristics (e.g., GABA (gamma-aminobutyric acid), glutamate dysregulation). We also hypothesize that these mechanisms correlate with cognitive phenotypes (e.g., ASD: social cognition, attention to detail, flexibility, planning; ADHD: working memory, inhibition, timing, reward dependence), with categorically and dimensionally defined ASD/ADHD and ASD/ADHD symptoms, and with functional impairment. Finally, we predict that these findings will emerge as specific compared to typical controls, and partly specific to ASD or ADHD.
Participants
The long-term objective of the study is to collect 50 MZ pairs qualitatively or markedly quantitatively discordant for ASD or broader autism phenotype/autism traits, as well as 50 MZ pairs qualitatively or markedly quantitatively discordant for either ADHD or broader ADHD phenotype/symptoms, all aged 8 to 50 years. In addition, the studies aim to include 30 MZ pairs concordant for each ASD and ADHD, and 40 MZ typically developed control pairs, and 40 MZ pairs discordant for other neurodevelopmental disorders. Twin zygosity is determined on a panel of 48 single nucleotide polymorphisms (SNPs; Hannelius et al., Reference Hannelius, Gherman, Makela, Lindstedt, Zucchelli, Lagerberg and Lindgren2007). A more balanced gender distribution than in the general population is aimed to be accomplished in order to allow gender-specific research. Exclusion criteria for all participants are profound intellectual disability (IQ < 35), serious psychiatric (e.g., paranoid schizophrenia) or neurological (intractable epilepsy) conditions, or any well-defined genetic syndrome (e.g., fragile X). Additional exclusion criteria will apply for certain assessments, such as metallic implants, brain surgery, or claustrophobia for magnetic resonance (MR) neuroimaging. Inclusion criteria for ASD will be an ICD-10-defined diagnosis of autism (F84.0), Asperger syndrome (F84.5), or atypical autism/PDD-NOS (F84.1, F84.8, F84.9), or a DSM-5 diagnosis of ASD. For ADHD, the inclusion criteria will be any ICD-10-defined diagnosis of hyperkinetic disorder (F90.0-F90.9) or DSM-5 diagnosis of ADHD (combined, inattentive, or hyperactive-impulsive subtype). Qualitative discordancy is defined as a main diagnosis of ASD or ADHD in twin 1, and either no diagnosis (typical developmental) or a different main diagnosis in the co-twin. Marked quantitative discordancy is defined by: (1) both twins having a diagnosis in the same diagnostic group but the diagnoses differ in severity (e.g., autism vs. PDD NOS [ICD-10]; ASD with different severity specifiers [DSM-5]); (2) twins scoring with ≥1 SD difference on dimensional ASD (e.g., SRS and/or AQ) or ADHD (Conners-3 and/or CBCL) scales; (3) in addition, not stressing clinically striking phenotypic differences, the concept of discordancy will also be applied more flexibly and exploratory using smaller differences of ASD-like, ADHD-like, and other neurodevelopmental traits.
Twins are currently being recruited from four sources: (1) the primary resource is the Child and Adolescent Twin Study in Sweden (CATSS; Anckarsäter et al., Reference Anckarsäter, Lundström, Kollberg, Kerekes, Palm, Carlström and Lichtenstein2011) targeting all twins born in Sweden since 1994; the response rate for CATSS is approximately 80%, with >25,000 twins born between 1993 and 2004; (2) the patient registry of the Swedish Board of Health and Welfare; (3) the clinical registries of the Division of Child and Adolescent Psychiatry, the Habilitation and Health centers, and pediatric units in Stockholm County; (4) summons in the journals of the National Society for neurodevelopmental disorders (Riksförbundet Attention; 13,000 members), the National Society for Autism and Asperger syndrome (Autism och Aspergerföreningen; 12,000 members), and the national twin organization (Tvillingklubben), exhibitions at their (bi-)annual meetings, and other forms of media (e.g., reports on the project in newspapers and on television).
Procedure and Measures
Assessments within RATSS are comprehensive, lasting up to 3 days and with participants recruited from all over Sweden. Participants are continuously accompanied by an experienced research nurse from the first contact to the end of the assessments. In case of identification of yet undetected psychiatric or medical problems or the emergence of adverse effects connected to the assessments, the project team can adequately and swiftly provide or refer to services. Assessments are carried out in Stockholm, Sweden, by experienced clinical staff (nurses, psychologists, and physicians) at the Astrid Lindgren Children's Hospital, the Center of Neurodevelopmental Disorders at Karolinska Institutet, the Karolinska Institutet Neuroimaging Center, and St. Görans Hospital. Questionnaires are collected by mail before face-to-face assessments in order to limit the burden of the study. Biosamples are primarily collected from participants during the visits, but also via express service (e.g., saliva, feces).
Diagnosis, general psychopathology
MZ twins with suspicion of a previous clinically confirmed diagnosis of ASD or ADHD are followed up to corroborate the suspicion/diagnosis and create comparable clinical data. For ASD, the Swedish forms of Autism Diagnostic Interview – Revised (ADI-R) and the Autism Diagnostic Observation Schedule (ADOS-2) are used (note that the first author is a certified ADOS and ADI-R trainer). A diagnosis of ADHD is established using the Kiddie Schedule for Affective Disorders and Schizophrenia (K-SADS) or the Diagnostic Interview for ADHD in adults (DIVA). The K-SADS is also used to examine psychiatric comorbidity in both ASD and ADHD. In adults, the Structured Clinical Interview for DSM-IV (SCID, axis I) is collected to assess comorbidity. Additional ASD measures collected in all twins are the (parent report) Social Responsiveness Scale (SRS; or adult version, SRS-A) and the Autism Spectrum Quotient (AQ; or AQ-children). The parent report Conners 3 and the Adult ADHD Self Report Scale are used to quantify ADHD. To assess and quantify general psychopathology, age-appropriate versions of the Achenbach Scales (e.g., Child Behavior Checklist, Young Adult Behavior Checklist) are collected. The Children's Communication Checklist (or Communication Checklist for Adults), Sensory Profile for children or adults, and Adaptive Behavior Assessment Scale-II are obtained for additional psychopathological and functional phenotypic assessment.
Cognition
Cognitive testing comprises different versions of the children's or adult Wechsler IQ (WISC-IV, WAIS-IV) or Leiter scales, and the Peabody Picture Vocabulary Test (in the case of low/borderline intellectual functioning) for general and verbal abilities in all participants. The Reading Mind in the Eyes Test and Movie for the Assessment of Social Cognition are collected for social cognition in ASD and ADHD. The Fragmented Picture Test and (Children’s) Embedded Figures Test are administered for attention to detail/central coherence, and the Tower Test (in D-KEFS) and Wisconsin Card Sorting Test for executive problems in terms of foresighted planning and cognitive flexibility in ASD. The Corsi Block Test for visuospatial working memory (also Digit Span of the Wechsler will be available for verbal working memory), the Conner's Continuous Performance Test for sustained and selective attention and inhibition, a modified go/no-go task for timing, and the choice delay task for delay aversion are used to test executive problems in ADHD.
Medical history
Comprehensive anamnesis and socio-demographic information are collected, including details on familial burden, pregnancy, mother's health and habits, obstetric complications, early nutrition/breastfeeding, viral infections, allergies, food intolerances, medication, parents’ socio-economic status and education, school problems, and diagnostic and therapeutic procedures. Existing clinical records are also reviewed.
Dysmorphology, specimens
Signs of dysmorphology are examined thoroughly by two experienced clinical geneticists, and photographs of the face, hands, feet, and habitus are taken by a professional clinical photographer for quantitative dysmorphology analyses. Samples of blood, saliva, skin, cerebrospinal fluid (CSF), urine, baby teeth (if available, for toxicity; e.g., metals analysis; not described in detail in this proposal), and stool are collected.
Neuroimaging
If the preliminary safety questionnaire reveals no exclusion criterion, an ~50-minute session in a 3 Tesla MR750 GE scanner at the Karolinska Institutet MR center is conducted. A 32-channel coil array is used and the participants’ head position fixed with foam pads to minimize movement. This MR session includes a T1-weighted Inversion Recovery Fast Spoiled Gradient Echo (IR-FSPGR) 3D-volume, two 60-direction Diffusion Weighted Imaging (DWI) sequences (b-value of 1,000 and 3,000 s/mm2), and a resting-state session including 200 T2-weighted Echo Planar Image (EPIs) volumes. In addition, a T2-weighted Fluid Attenuated Inversion Recovery (FLAIR) volume is acquired and checked by an experienced neuroradiologist. With regard to metabolic imaging (MR Spectroscopy; MRS), we will soon add a second session using the same 3T GE scanner with an 8-channel coil array. A Mescher-Garwood point-resolved spectroscopy (MEGA-PRESS) sequence will be used. Volumes of interest (VOI) of 8 to 15 mL will be accurately positioned on two-to-three brain major network nodes using a T1-weighted IR-FSPGR volume (see above for details).
Analyses
We predict differences between affected MZ twins with ASD or ADHD (and pairs discordant for lesser phenotypes) and their unaffected, less affected, or differently affected co-twins with regard to medical history, signs of dysmorphology, psychiatric comorbidity, and neurocognition. Those characteristics are not present in the concordant MZ pairs and other typically developing MZ pairs, hence indicating non-shared risks. In addition, we hypothesize additional differences with regard to these parameters between concordant ASD and ADHD MZ pairs and MZ typically developed pairs, in turn (indicating shared risks for neurodevelopmental disorders). Results from these analyses will help us understand the genetic and epigenetic, CSF, microbiota, and neurobiological findings (see below), as well as generate more phenotype-based, hypotheses-driven studies.
The primary objective of the brain imaging part of RATSS is to examine the ‘brain connectome’ in ASD and ADHD. This model allows us to map and understand in vivo the structural and functional neural connections forming the brain at the network level (Sporns et al., Reference Sporns, Tononi and Kötter2005). As for the ASD affected individuals, overall, we expect either a mixed pattern of local over-connectivity and long-distance under-connectivity (of the frontal lobe mainly; Courchesne & Pierce, Reference Courchesne and Pierce2005), or a differently organized network topology. For ADHD, a hypotheses-free exploratory approach will be applied. The secondary aim of the neurobiological investigations is to complete the brain connectome approach with morphometric and further functional (e.g., MRS) investigations. With regard to morphometry, RATSS will analyze, on a whole-brain basis, gray/white matter volumetry, cortical folding (gyrification) patterns, cortical thickness, and sulcal morphometry indices to provide a characterization of the ASD and ADHD cerebral patterns. These can be used as biomarkers in individuals with neurodevelopmental disorders (e.g., Shokouhi et al, Reference Shokouhi, Williams, Waiter and Condon2012). 1H-MRS is a promising tool for identifying metabolic biomarkers in neurodevelopmental disorders. Several MRS voxels will be positioned on major brain nodes, either suspected to be associated to abnormal neuronal processing (e.g. under- or over-functional or structural connectivity) or to demonstrate significant anatomical differences. Among the major hallmarks of 1H-MRS spectra in human brain, the glutamate, glutamine (Glu, Gln, often Glu + Gln = Glx), and GABA are the most relevant for RATSS as they reflect the equilibrium between the excitatory and inhibitory neurotransmission systems, which in turn can be specific to certain network nodes. In addition, these metabolites are involved not only in signal transduction, but also brain maturation and cortical organization, especially neuronal migration and positioning, which is of high relevance for neurodevelopmental disorders. Accordingly, GABA and glutamate have been found to be involved in the pathophysiology of neurodevelopmental disorder, particularly ASD (e.g., DeVito et al., Reference DeVito, Drost, Neufeld, Rajakumar, Pavlovsky, Williamson and Nicolson2007; Ecker et al., Reference Ecker, Spooren and Murphy2013; Pardo & Eberhart, Reference Pardo and Eberhart2007).
CSF is more likely than blood to contain potential markers of disorders in the central nervous system because the chemical properties of CSF more closely reflect the biochemistry of the brain. CSF markers have the potential to act as diagnostics tools but can also function as predictors for treatment response, side effects, and/or disease progression (Perlis, Reference Perlis2011). CSF-based neurochemical diagnostics have been successfully developed for other neurological disorders (e.g., Alzheimer's disease), but not ASD or ADHD. In RATSS, established biomarkers of neurodegeneration, brain injury, synaptic function, inflammation, and monoamines will be assessed in CSF. The limitation with this candidate marker approach is, however, that we are limited to a set of tools that we already know are of some importance. We will therefore complement these analyses with an approach that is better suited to detect novel markers that may advance the field: hypothesis-free combined proteomics and peptidomics for the global analysis of proteins and endogenous peptides in CSF. New mass spectrometry-based proteomic techniques are now allowing researchers to detect and identify hundreds of biomolecules in a single analysis of minute biological samples. These techniques enable a more unprejudiced research approach that may lead to the discovery of novel molecules involved in disease, as well as disease mechanisms that involve co-action of several molecules. Several research articles in the last few years indicate the feasibility to employ these techniques in the discovery of biomarkers in CSF. By subtracting the protein/peptide pattern of the typical co-twins from that of the affected ASD/ADHD twin, we hope to identify proteins that are only present in a clinical/subclinical neurodevelopmental disorder phenotype (see Hölttä et al., Reference Hölttä, Zetterberg, Mirgorodskaya, Mattsson, Blennow and Gobom2012; Kroksveen et al., Reference Kroksveen, Opsahl, Aye, Ulvik and Berven2011).
Induced pluripotent stem (iPS) cells and iPS-derived NES cells from patients are ideal building blocks for the development of disease-specific cellular models (Falk et al., Reference Falk, Koch, Kesavan, Takashima, Ladewig, Alexander and Brüstle2012). Cellular models built on patient-specific iPS cells have successfully shown disease phenotypes, also when modeling psychiatric disorders (Christian et al., Reference Christian, Song and Ming2012; Park et al., Reference Park, Arora, Huo, Maherali, Ahfeldt, Shimamura and Daley2008). The few reprogramming studies of cells from patients with psychiatric disorders have had encouraging results, proving the principle, as well as presenting possible molecular mechanisms. For example, calcium Ca(v)1.2 has been suggested to regulate the differentiation of cortical neurons in humans and offer new insights into the causes of autism in individuals with Timothy syndrome (Pasca et al., Reference Pasca, Portmann, Voineagu, Yazawa, Shcheglovitov, Pasca and Dolmetsch2011). We will use the skin biopsies from MZ pairs discordant for ASD or ADHD, to derive iPS and NES cells that are further differentiated into disease-relevant neurons. The advantage of using NES cells instead of iPS cells as a starting cell line for directed differentiation is that NES cells only differentiate into neural cell types (neurons and glia) and no cells from other germ layers will disturb differentiation. In addition, the differentiation protocol will span two weeks instead of 5–6 weeks. Protocols will be developed for deriving neuronal subtypes known to be affected in neurodevelopmental disorders, such as cortical neurons and GABAergic interneurons. The literature provides good insight into signaling molecules important for these fates (Jagatha et al., Reference Jagatha, Divya, Sanalkumar, Indulekha, Vidyanand, Divya and James2009). To investigate the mechanisms causing neurodevelopmental disorders, mutated patient NES cells are studied side by side with healthy NES cells using assays reporting proliferation, differentiation, migration, maturation, and survival of neural cells.
Our primary hypothesis in RATSS is that the symptoms in the affected twin are caused by epigenetic and genetic differences between the twins. To identify the putative differences, a broad range of genetic analyses, including high-density DNA microarrays and whole exome and genome sequencing (WES and WGS, respectively) will be performed. First, the discordant twin pairs will be screened for their CNV status using high-resolution DNA microarrays. To identify putative pathogenic variants, the CNVs from twins with ASD or ADHD will be compared to control twins and other available controls. Then, WES and/or WGS of the twins and their parents will be used to pinpoint rare and/or de novo mutations, including mosaic mutations. To identify any epigenetic differences between the twins, a microarray-based global methylation analysis will be performed using the collected blood samples. Any identified differentially methylated regions will be confirmed by pyrosequencing. Furthermore, we can correlate the methylation status to gene expression in blood-derived ribonucleic acid (RNA) using quantitative Reverse Transcriptase Polymerase Chain Reaction (qRT-PCR) (selected genes) or RNA sequencing (global gene expression). For some of the twins, we will be able to measure the methylation status at birth from dried blood spots and detect persistent methylation differences or changes in methylation status during childhood that could contribute to symptoms seen in the affected twin.
In addition to the clinical manifestations typically associated with central nervous system (CNS) dysfunctions, ASD (and to a lesser extent ADHD) has been frequently linked to various gastrointestinal (GI) symptoms such as abdominal pain, constipation, diarrhea, enterocolitis, and abnormal intestinal permeability (Kral et al., Reference Kral, Eriksen, Souders and Pinto-Martin2013). GI functions are critically dependent on the integrity and diversity of the gut microbiota, that is, the collection of commensal microorganisms that colonize the GI tract. It has therefore been suggested that at least parts of the GI symptoms in ASD may stem from alterations in gut microbiota, and such microbial changes may be amenable to therapeutic interventions that could improve the general life qualities of affected individuals (De Angelis et al., Reference De Angelis, Piccolo, Vannini, Siragusa, De Giacomo, Serrazzenetti and Francavilla2013). The gut microbiota has further emerged as an important functional node within the gut-brain axis, which in turn functionally interconnects the viscera, including the GI tract, with the CNS (Mayer, Reference Mayer2012). One of the burning questions in this context is whether GI- and CNS-associated symptoms in neurodevelopmental disorders such as ASD are two separate pathological entities, or whether they are causally related. Owing to the constant bidirectional information flow between the gut and the brain, it is indeed feasible to assume that GI disturbances may causally contribute to at least some brain dysfunctions (Reichelt & Knivsberg, Reference Reichelt and Knivsberg2009). In RATSS, we test the hypothesis that primary alterations in the gut microbiota not only facilitates the emergence of GI dysfunction, but also impacts brain and behavioral functions via changes in afferent vagal signaling, gut immunity, and/or altered secretion of neuroactive substances. In the current project, the nature of microbiotic changes in fecal samples obtained from twins affected by ASD and ADHD are compared to the microflora of their unaffected co-twins. Potential differences in the microbiota between twins and co-twins are related to differences in psychopathology, cognition, and functional and structural neuroimaging findings, as a well as CSF biomarkers, genetics, and epigenetics.
Results
The RATSS data collection began in mid-2011. As of October 2013, N = 109 individuals (68 males, 41 females) from 54 twin-pairs and one set of triplets (Figure 1) have been assessed using the RATSS protocol, and an additional eight pairs are scheduled for 2013. Eighty-two are MZ, 20 dizygotic (DZ), and zygosity is pending in eight individuals. In three twin pairs, twin-to-twin transfusion syndrome was reported. The average age is 15.0 years (SD = 3.2, range = 9–23); the average IQ is 93.3 (SD = 18.9, range = 39–138). Sixty-six percent of the sample has been recruited via the CATSS study, 28% via advertisements, 4% from clinical unit (e.g., child psychiatry), and 2% from the Swedish patient registry.
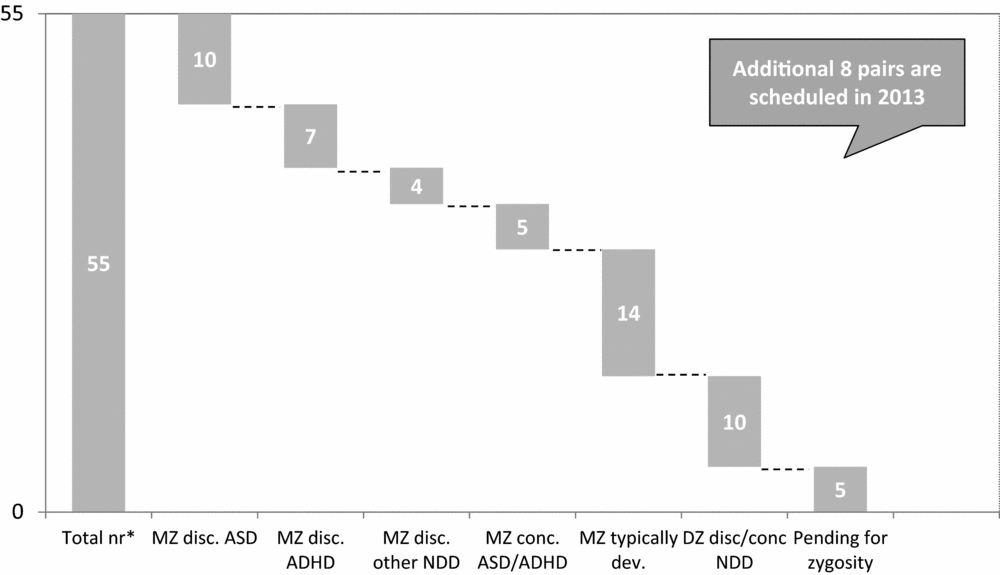
FIGURE 1 Number and type of twin-pairs collected in RATSS on October 9, 2013. Note: *Including one trio of triplets, counted as two pairs. ASD = autism spectrum disorder; ADHD = attention-deficit hyperactivity disorder; DZ = dizygotic; MZ = monozygotic; NDD = neurodevelopmental disorders; TD = typically developed.
Ten MZ twin-pairs are qualitatively or markedly quantitatively discordant for ASD and seven for ADHD. Please note that where one twin was diagnosed with ASD and the co-twin with ADHD, twin pairs were assigned to the ASD discordant pairs count. One additional pair pending for zygosity testing results, at the time of the writing of this article, was highly likely to be MZ and discordant for ASD (Table 1). Moreover, the sample includes four MZ pairs discordant for other neurodevelopmental disorders (intellectual disability, tic disorder, speech sound disorder), and four MZ pairs concordant for ASD or ADHD.
TABLE 1 List of monozygotic twin pairs discordant for autism spectrum disorder, ADHD, and other neurodevelopmental disorders (DSM-5/ICD-10 diagnoses) in RATSS
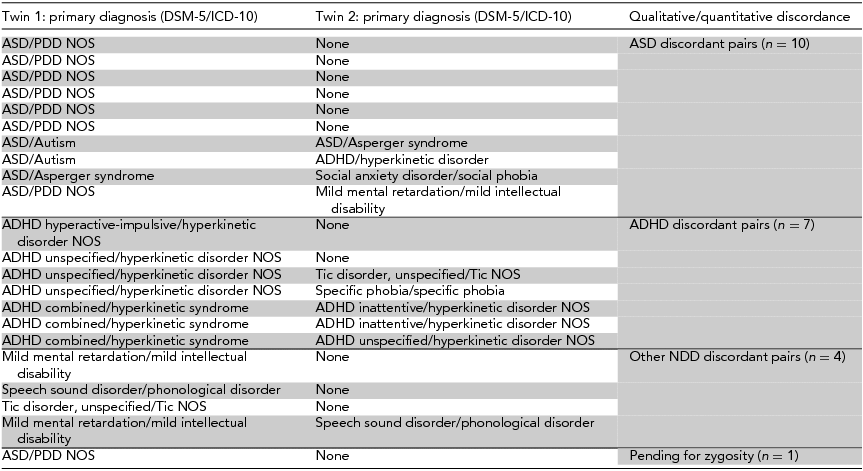
Note: DSM = Diagnostic and Statistical Manual of Mental Disorders; ICD = International Classification of Diseases; ASD = autism spectrum disorder; ADHD = attention-deficit hyperactivity disorder; PDD NOS = pervasive developmental disorder not otherwise specified; NOS = not otherwise specified; NDD = neurodevelopmental disorders.
Overall, the completeness and usability of the collected data and specimens is adequate (Figure 2). Data for psychiatric and psychological assessments are almost complete, and so are samples of blood, saliva, hair, nails, and urine (>99%). Coverage is somewhat lower for dysmorphology examination, extensive medical history taking (93%), and magnetic resonance imaging (MRI; 88%). Coverage is lowest for CSF (63%) and skin biopsies (55%). However, the collection of skin samples was only introduced 1 year ago. With regard to parental data and specimens, RATSS has almost complete questionnaire data on mothers and fathers (89%), as well as a good coverage for mothers’ (93%) and fathers’ blood (59%) and saliva (98% and 83%; Figure 3).
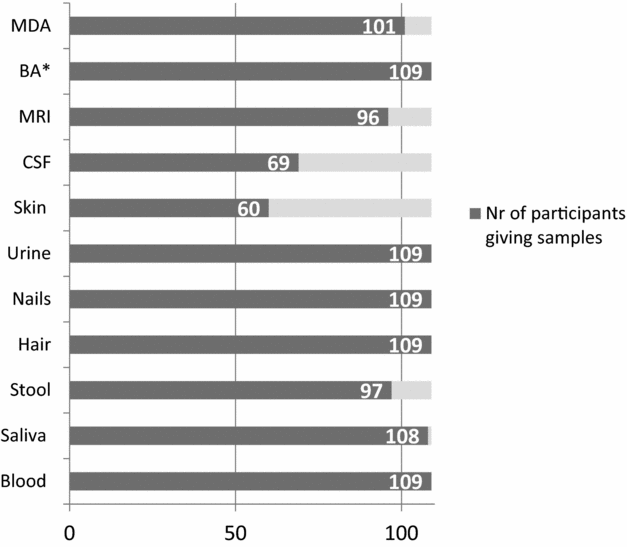
FIGURE 2 Completeness of twin pair assessments and specimen collections in RATSS. Note: *Minimum assessment = verbal and nonverbal IQ + Autism Diagnostic Observation Scale (ADOS) + parent report. MDA = medical and dysmorphologic assessment; BA = behavioral assessment; MRI = magnetic resonance imaging; CSF = cerebrospinal fluid.

FIGURE 3 Completeness of parents’ assessments and specimen collections in RATSS. Note: *Questionnaires = Autism Quotient & Adult Self Report.
Current ongoing analyses focus on differences in executive functioning in MZ twins discordant for ADHD traits, including the role of age and gender effects in 27 pairs; a detailed descriptive comparison analysis of the nine MZ pairs discordant for ASD. Brain imaging data analyses focus on the structural connectome (mapping whole-brain white matter bundles), observed in the most discordant ASD and ADHD MZ twin pairs, as well as morphometric investigations; for example, cortical surface reconstructions, analyses of cortical thickness, cortical folding and gray/white matter contrast (a proxy of myelination), volumetry of subcortical structures at the sample level. CSF analyses focus on the inflammatory markers and markers of brain injury. All the collected twins will be genotyped using Infinium HumanCoreExome chip with additional 50K SNPs relevant for psychiatric disorders (PsychChip). This platform will allow detection of CNVs larger than 30 kb in addition to 387 CNVs found to be associated with different psychiatric conditions and enable us to explore the association of SNPs and different behavioral, cognitive, and neurobiological phenotypes of the twins. Exome sequencing is planned for the most clinically discordant pairs for ASD, ADHD, and other neurodevelopmental disorders together with their parents.
Conclusions
RATSS is the largest multilevel clinical study of ASD and ADHD and their broader phenotypes and traits, using MZ twins. The project has already generated a rich collection of data to be used in a variety of analyses described in this article. The project will shortly have access to new options of recruitment via clinically based registries in Stockholm County (Division of Child and Adolescent Psychiatry, Habilitation and Health Centers, Pediatrics) to markedly increase the number and ‘clinicalness’ of the twin pair collection. Overall, there is good likelihood that the project will be successful in doubling or tripling the amount of twins and material in the next 2–3 years. For 2015, it is planned to start following up on the collected pairs, beginning with the most informative (discordant clinical ASD and ADHD) pairs, in order to establish the longitudinal arm of RATSS.
Recently, parts of RATSS joined EU-AIMS: European Autism Interventions — a multicenter study for developing new medication. The involvement in EU-AIMS will create synergies, as well as higher research output and visibility of RATSS. The engagement in EU-AIMS also means that additional assessments are integrated in RATSS, such as eye-tracking, questionnaires, cognitive tests, and brain imaging (molecular positron emission tomography — PET). As RATSS combines several strengths, particularly access to nation-wide registry data on twins screened for neurodevelopmental disorder traits, multilevel assessments, and a multidisciplinary environment, there is a good chance for novel leads regarding pathogenic pathways, informative biomarkers, and options for treatment and prevention in ASD and ADHD. There are several techniques developed by collaborators in this project that have never been used in ASD or ADHD or in twins discordant for these phenotypes, such as iPS and NES cells, mass spectrometry-based proteomic techniques analyzing the CSF, and brain connectome approaches.
Despite all of the advantages of twin studies, there are some potential limitations, making cross-validation of twin findings in singletons desirable for endorsing generalizability to the total population. Twins are often born premature and are lighter at birth than singletons, which could compromise the ability of twins to represent the general population. Some authors have suggested twinning to be a risk factor for some neurodevelopmental disorders (e.g., ASD), but this has not been confirmed in large population-based studies (e.g., Hallmayer et al., Reference Hallmayer, Glasson, Bower, Petterson, Croen, Grether and Risch2002); in twin family studies including siblings that controlled for confounds (e.g., parental education, socio-economic status), sibs and twins did not differ (e.g., for IQ, see Hoekstra et al., Reference Hoekstra, Bartels, Verweij and Boomsma2007).
In conclusion, RATSS provides some unique opportunities to examine altered development in ASD and ADHD, hazardous and protective environmental and genetic factors and their interplay, as well as their effects on neurobiology, and cognition. Finally, while RATSS focuses on ASD, ADHD, and other neurodevelopmental disorders, the twin collection offers many opportunities for spin-off research through collaboration with additional medical disciplines, as quite a few of the MZ twin-pairs show discordance for other medical conditions, such as epilepsy, deafness, or asthma.
Acknowledgments
We greatly acknowledge Kerstin Andersson, Élodie Cauvet, Ann-Charlotte Engström, Anna Lange Nilsson, Martin Hammar, and Anna Råde for data collection in RATSS. Furthermore, we sincerely thank all twins and their relatives who participate in RATSS. The RATSS project is supported by the Swedish Research Council; the Swedish Research Council in partnership with FAS, FORMAS, and VINNOVA (a cross-disciplinary research program concerning children's and youth's mental health, grant no. 259-2012-24); Innovative Medicines Initiative Joint Undertaking (under grant agreement no. 115300), resources of which are composed of financial contribution from the European Union's Seventh Framework Programme (grant no. FP7/2007–2013) and EFPIA companies’ in kind contribution; Stockholm County Council, Sällskapet Barnavård, The Swedish Order of Freemasons Grand Loge Stockholm, Stiftelsen Sunnerdahls Handikappfond, Hjärnfonden, Autism and Asperger Society Stockholm, Tore Nilssons Stiftelsen, Jeanssons Stiftelse, Magnus Bergvalls Stiftelse, and PRIMA Barn- och Vuxenpsykiatri.