Bone structure is maintained by a dynamic balance between osteoblast-mediated bone formation and osteoclast-mediated bone resorption( Reference Seeman and Delmas 1 , Reference Chen, Lin and Chou 2 ). In particular, osteoblasts, differentiated from mesenchymal stem cells, play critical roles in bone formation( Reference Takeda and Karsenty 3 ). During osteogenesis, stem and primitive osteoprogenitors that are differentiated from stromal stem cells can replace osteoblasts, which promote bone turnover and fracture healing( Reference Engin and Lee 4 ). Additionally, sequential differentiation of these precursor cells leads to osteoblast maturation and mineralisation( Reference Takeda and Karsenty 3 , Reference Engin and Lee 4 ). A complicated network of various proteins is involved in the regulation of osteogenesis( Reference Vandenput and Ohlsson 5 , Reference Hung, Chen and Liao 6 ). For example, endogenous bone morphogenetic proteins (BMP) are multifunctional growth factors that participate in matrix differentiation and bone formation( Reference Kugimiya, Kawaguchi and Kamekura 7 , Reference Trzeciakiewicz, Habauzit and Mercier 8 ). Osteocalcin (OCN) can act as an early osteoblast marker that controls osteoblast function and bone extracellular matrix mineralisation( Reference van Leeuwen, van Driel and van den Bemd 9 ). In addition, collagen type I (Col I), an extracellular matrix protein, stimulates osteoblast adhesion and differentiation( Reference Mizuno and Kuboki 10 , Reference Mathews, Bhonde and Gupta 11 ). The expressions of all these differentiation-related genes can be regulated by several local and systemic factors, including oestrogen, growth factors and cytokines( Reference Hung, Chen and Liao 6 , Reference Fiorelli and Brandi 12 , Reference Hsu, Liao and Tai 13 ).
Oestrogen acts as the main regulator of skeletal growth and maintenance by adjusting osteoblast differentiation and metabolism( Reference Fiorelli and Brandi 12 ). The levels of serum oestrogen are significantly diminished with ageing and concurrently bring about an imbalance of osteoblastogenesis and osteoclastogenesis( Reference Fiorelli and Brandi 12 , Reference Krum 14 ). As a result, reduced serum oestrogen concentrations may cause systemic diseases such as osteoporosis, leading to increased risks of bone fracture( Reference Manolagas 15 , Reference Eastell and Hannon 16 ). In the clinic, oestrogen replacement therapy has been applied to treat osteoporosis, but many side effects, including a certain cancer incidence, have been reported( Reference Modugno, Laskey and Smith 17 ). As an alternative, phyto-oestrogens are plant-derived compounds that exhibit effects similar to those of mammalian estrogens; they have been widely studied to explore their potential to treat osteoporosis( Reference Usui 18 ). Genistein is a non-steroidal phyto-oestrogen found in a number of plants, including lupin, fava beans and soyabeans( Reference Coward, Barnes and Setchell 19 ). Because of a structural similarity to 17β-oestradiol, genistein has been proposed as a potential therapeutic agent for preventing postmenopausal bone loss( Reference Poulsen and Kruger 20 ). The major mechanism of genistein's action is the control of osteoblast metabolism through oestrogen receptor (ER)-dependent pathways( Reference Wang, Sathyamoorthy and Phang 21 ).
ER are a family of intracellular receptors, including ERα and ERβ( Reference Dahlman-Wright, Cavailles and Fuqua 22 ). ERα has been reported to be the major regulator mediating the effects of oestrogen on bone metabolism( Reference Sims, Clément-Lacroix and Minet 23 ). According to the classical mechanism, oestrogen binds to ERα to form a receptor dimer, and then this dimer is translocated from the cytoplasm to the nucleus where it associates with co-regulatory proteins( Reference Levy, Zhao and Tang 24 ). After binding to its specific oestrogen-response elements, this ER–oestrogen complex can initiate certain gene expressions and control osteoblast differentiation( Reference Gruber, Gruber and Gruber 25 ). Genistein may have a noteworthy impact on the prevention and therapy of postmenopausal bone loss( Reference Poulsen and Kruger 20 ). One of the mechanisms of genistein's action is the stimulation of osteoblast maturation via an ER-dependent induction of cell proliferation-related gene expressions( Reference Pan, Quarles and Song 26 ). Other mechanisms may be the promotion of osteogenesis and repression of adipogenic differentiation of human primary bone marrow stromal cells( Reference Heim, Frank and Kampmann 27 ). In addition, genistein can induce ERα mRNA expression in bone marrow cells( Reference Liao, Xiao and Qin 28 ). ERα plays an important role in the mediation of genistein-involved regulation of osteoblast differentiation and mineralisation. However, the effects of genistein on the regulation of ERα gene expression in osteoblasts are still not very clear. Therefore, the present study attempted to evaluate the mechanisms of genistein-induced ERα gene expression and the effects of genistein on the regulation of osteoblast differentiation and mineralisation using MC3T3-E1 cells and primary rat calvarial osteoblasts as the experimental models.
Materials and methods
Cell culture and drug treatment
Mouse MC3T3-E1 cells were purchased from American Type Culture Collection. Primary rat osteoblasts were prepared from neonatal rat calvaria following a sequential enzymatic digestion method described previously( Reference Hung, Chen and Liao 6 ). All procedures were performed according to the National Institutes of Health Guidelines for the Use of Laboratory Animals and approved by the Institutional Animal Care and Use Committee of Taipei Medical University (Taipei, Taiwan). Briefly, after anaesthesia, the neonatal rats were killed, and the calvarias were collected, washed and cut into pieces. The calvarial pieces were incubated in a digestion solution (0·25 % trypsin, 2 g/l collagenases and 0·1 % EDTA) at 37°C. After centrifugation, the primary osteoblasts were washed and cultured. MC3T3-E1 cells and primary rat osteoblasts were seeded in Dulbecco's modified Eagle's medium (Gibco-BRL) supplemented with 10 % fetal bovine serum, l-glutamine, penicillin (65 mg/l) and streptomycin (100 μg/ml) in 75 cm2 flasks at 37°C in a humidified atmosphere of 5 % CO2. Genistein purchased from Sigma was freshly dissolved in dimethyl sulphoxide. These two types of bone cells were exposed to various concentrations of genistein for different time intervals. Control cells were exposed to only dimethyl sulphoxide.
Assay of cell viability
The cytotoxicity of genistein towards osteoblasts was evaluated by analyses of cell viability and morphologies( Reference Hsu, Liao and Tai 13 ). Cell viability was determined by a colorimetric 3-(4,5-dimethylthiazol-2-yl)-2,5-diphenyltetrazolium bromide (MTT) assay. Briefly, 104 cells were seeded in ninety-six-well tissue culture plates overnight. After genistein treatment, the osteoblasts were cultured with a new medium containing 0·5 mg/ml of MTT for a further 3 h. Blue formazan products in the osteoblasts were dissolved in dimethyl sulphoxide and spectrophotometrically measured at a wavelength of 550 nm. Cell morphologies were observed and photographed using an inverted phase-contrast microscope (Nikon).
Immunodetection of phosphorylated extracellular signal-regulated kinase 1/2, p38 and c-Jun N-terminal kinase and β-actin proteins
After genistein treatment, cell lysates were prepared in an ice-cold radioimmunoprecipitation assay buffer (25 mm-Tris–HCl (pH 7·2), 0·1 % SDS, 1 % Triton X-100, 1 % sodium deoxycholate, 0·15 m-NaCl and 1 mm-EDTA) as described previously( Reference Chang, Chen and Tai 29 ). Protein concentrations were quantified using a bicinchoninic acid protein assay kit (Pierce). Proteins (100 μg per well) were subjected to SDS–PAGE and transferred onto nitrocellulose membranes. After blocking, phosphorylated extracellular signal-regulated kinase (ERK) 1/2, p38 and c-Jun N-terminal kinase (JNK; Cell Signaling Technology) were immunodetected. β-Actin was detected using a mouse monoclonal antibody (Sigma) as the internal control. The bands of these proteins were quantified using a digital imaging system (UVtec).
Extraction of nuclear proteins and immunodetection
Nuclear components were extracted, and immunodetection was carried out following the method of Wu et al. ( Reference Wu, Chen and Ueng 30 ). After genistein treatment, the nuclear extracts of osteoblasts were prepared. Protein concentrations were quantified using a bicinchoninic acid protein assay kit (Pierce). Nuclear proteins (50 μg/well) were subjected to SDS–PAGE and transferred onto nitrocellulose membranes. After blocking, nuclear ERα, NF-κB, c-Jun and c-Fos were immunodetected using specific antibodies (Santa Cruz Biotechnology). Proliferating cell nuclear antigen was immunodetected (Santa Cruz Biotechnology) as the internal standard. The intensities of the immunoreactive bands were determined using a digital imaging system (UVtec).
RT-PCR and real-time PCR assays
For the RT-PCR analyses of ERα, Col I, BMP-6, OCN and β-actin mRNA, mRNA from MC3T3-E1 cells and primary rat osteoblasts were prepared according to a previously described method( Reference Lin, Chen and Hong 31 ). Oligonucleotide primers were designed and synthesised by Clontech Laboratories. The oligonucleotide sequences of the respective upstream and downstream primers for analyses of these mRNA were as follows: 5′-TCCTTCTAGACCCTTCAGTGAAGCC-3′ and 5′-ACATGTCAAAGATCTCCACCATGCC-3′ for ERα; 5′-AAAGCCAAGAGAAACGGTGGGCAT-3′ and 5′-GCCAATCATGTGCACCAGTTCCTT-3′ for ERβ; 5′-CTTGTCCTCATGGCTGTGAAAC-3′ and 5′-TATTGCTGGTGCTCCTGGCTTC-3′ for Col I; 5′-AGGATGGGGTGTCAGAGGGAGA-3′ and 5′-GTTGTGCTGCGGTGTCACCA-3′ for BMP-6; 5′-ATGAGGACCCTCTCTCTGCTC-3′ and 5′-GTGGTGCCATAATGCGCTTG-3′ for OCN and 5′-GTGGGCCGCTCTAGGCACCAA-3′ and 5′-CTCTTTGATGTCACGCACGATTTC-3′ for rat β-actin. The PCR products were loaded onto a 1·8 % agarose gel containing 0·1 μg/ml ethidium bromide and electrophoretically separated. DNA bands were visualised and photographed under UV-light exposure. The intensities of the DNA bands in the agarose gel were quantified with the aid of a digital imaging system (Uvtec). Real-time PCR analyses were carried out using the iQSYBR Green Supermix (Bio-Rad) and the MyiQ Single-Color Real-Time PCR Detection System (Bio-Rad).
Assays of osteoblast mineralisation
Osteoblast maturation was determined by evaluating cell mineralisation using the Alizarin red S dye-staining protocol( Reference Hess, Angel and Schorpp-Kistner 32 ). Primary rat osteoblasts were treated with genistein, a differentiation reagent (DR; 10 nm-dexamethasone, 100 μg/ml ascorbic acid and 10 mm-β-glycerophosphate) and a combination of genistein and the DR for 21 d. After genistein treatment, rat osteoblasts were washed with ice-cold PBS (0·14 m-NaCl, 2·6 mm-KCl, 8 mm-Na2HPO4 and 1·5 mm-KH2PO4) and then fixed in ice-cold 10 % formalin for 20 min. The fixed osteoblasts were thoroughly rinsed and then incubated in 1 % alcian blue (pH 2·5; Fisher Scientific) for 12 h. The sections were then incubated in Alizarin red S (Fisher Scientific) for 8 min, briefly dehydrated in xylene and covered with a coverslip in Permount (Fisher Scientific). Mineralised nodules were visualised and counted under an inverted microscope. Each experiment was performed in duplicate wells and repeated three times.
Statistical analysis
Statistical differences between the control and drug-treated groups were considered significant when the P value of Duncan's multiple-range test was < 0·05. Statistical analysis between the drug-treated groups was carried out using a two-way ANOVA.
Results
The cytotoxicity of genistein towards MC3T3-E1 cells was determined (Fig. 1). Exposure of MC3T3-E1 cells to 0·01, 0·1, 1, 10 and 100 μm-genistein for 24 h did not influence cell viability (Fig. 1(a)). Additionally, after treatment of MC3T3-E1 cells with 1, 5 and 10 μm-genistein for 24 h, the cell morphologies did not change (Fig. 1(b)). Exposure of MC3T3-E1 cells to 10 μm-genistein for 0·5, 1, 3, 6 and 24 h did not affect the cell morphologies (Fig. 1(c)). After exposure to 0·01, 0·1, 1, 10 and 100 μm-genistein for 24 h, the viability of primary rat calvarial osteoblasts was not influenced (data not shown).
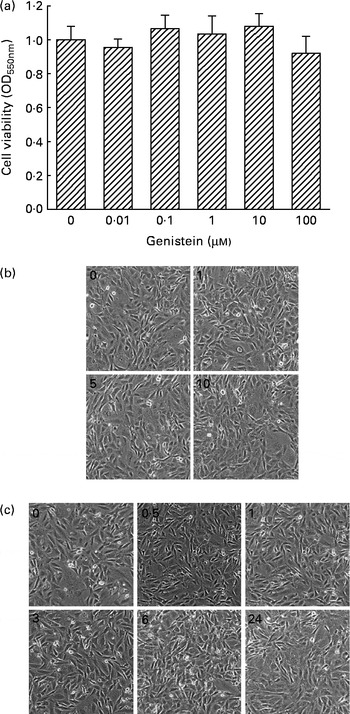
Fig. 1 Cytotoxicity of genistein towards MC3T3-E1 cells. (a) MC3T3-E1 cells were exposed to 0·01, 0·1, 1, 10 and 100 μm-genistein for 24 h, and cell viability was determined using a colorimetric method. The morphologies of MC3T3-E1 cells were observed after exposure to (b) 1, 5 and 10 μm-genistein for 24 h or to (c) 10 μm-genistein for 0·5, 1, 3, 6 and 24 h. Values are means (n 6), with their standard errors represented by vertical bars. OD550 nm, optical density at 550 nm.
The effects of genistein on the phosphorylation of mitogen-activated protein kinase (MAPK) and the translocation of NF-κB and activator protein-1 (AP-1) were immunodetected (Fig. 2). In the untreated MC3T3-E1 cells, low levels of phosphorylated ERK1/2, p38 MAPK and JNK1/2 were detected (Fig. 2(a), top three panels, lane 1). After exposure to 10 μm-genistein for 0·5, 1, 3 and 6 h, the levels of phosphorylated ERK1/2 were increased in a time-dependent manner (Fig. 2(a), top panel, lanes 2–5). The phosphorylation of p38 MAPK in MC3T3-E1 cells was also time dependently enhanced following exposure to 10 μm-genistein for 0·5, 1, 3 and 6 h (lanes 2–5). When MC3T3-E1 cells were treated with 10 μm-genistein for 6 h, the amounts of phosphorylated JNK1/2 increased (lane 5). β-Actin was immunodetected as the internal control (Fig. 2(a)). Exposure of MC3T3-E1 cells to 10 μm-genistein for 0·5 h increased the levels of nuclear NF-κB (Fig. 2(b), top panel, lane 2). After exposure for 1 and 3 h, genistein enhanced the increases in the amounts of nuclear NF-κB (lanes 3 and 4). The levels of c-Jun and c-Fos in the nuclei were immunodetected (Fig. 2(b)). Treatment of MC3T3-E1 cells with genistein for 0·5, 1 and 3 h led to significant increases in the levels of nuclear c-Jun and c-Fos (lanes 2 and 3). Nuclear proliferating cell nuclear antigen was analysed as the internal control (Fig. 2(b)).
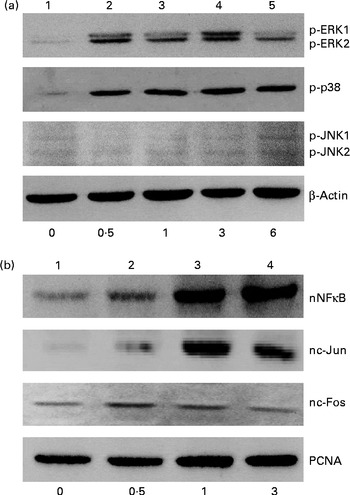
Fig. 2 Effects of genistein on the phosphorylation (p) of extracellular signal-regulated kinase (ERK) 1/2, p38 mitogen-activated protein kinase (MAPK) and c-Jun N-terminal kinase (JNK) 1/2 and the translocation of NF-κB, c-Jun and c-Fos. MC3T3-E1 cells were exposed to 10 μm-genistein for 0·5, 1, 3 and 6 h. (a) p-ERK1/2, p-p38 MAPK and p-JNK1/2 were immunodetected. The amounts of β-actin were analysed as internal controls. (b) The levels of nuclear (n) NF-κB, nc-Jun and nc-Fos were determined. The amounts of nuclear proliferating cell nuclear antigen (PCNA) were analysed as internal controls (bottom).
Analyses of RNA were performed to determine the effects of genistein on ERα and ERβ mRNA expressions (Fig. 3). Exposure of MC3T3-E1 cells to 1 μm-genistein for 6 h did not affect ERα mRNA expression (Fig. 3(a), top panel, lane 2). When the concentration reached 5 μm, genistein slightly induced ERα mRNA synthesis (lane 3). In contrast, 10 μm-genistein induced a strong ERα mRNA expression (lane 4). Treatment of MC3T3-E1 cells with 10 μm-genistein for 1 and 3 h did not influence ERα mRNA expression (Fig. 3(b), top panel, lanes 2 and 3). However, exposure for 6 and 24 h significantly induced ERα mRNA expression (lanes 4 and 5). The amounts of β-actin mRNA were analysed as internal controls (Fig. 3(a) and (b)). Real-time PCR analyses showed that genistein significantly induced ERα and ERβ mRNA expressions by 230 and 190 %, respectively (Fig. 3(c)).
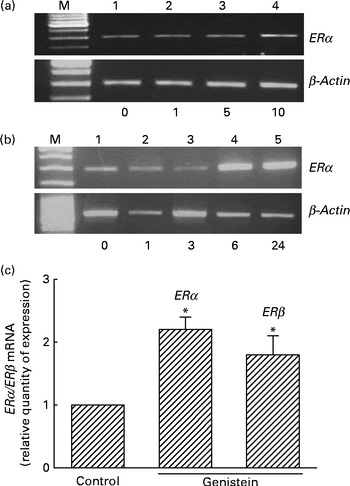
Fig. 3 Effects of genistein on the regulation of the expressions of oestrogen receptor (ER) α and ERβ mRNA. MC3T3-E1 cells were exposed to (a) 1, 5 and 10 μm-genistein for 6 h or to (b) 10 μm-genistein for 1, 3, 6 and 24 h. (a, b) Analysis of ERα mRNA was conducted using a RT-PCR. β-Actin mRNA was analysed as an internal control. (c) Real-time PCR analyses were conducted to confirm the effects of genistein on the regulation of ERα and ERβ mRNA expressions. Values are means (n 4), with their standard errors represented by vertical bars. * Mean value was significantly different from that of the control (P< 0·05). M, marker of 100 bp DNA ladder.
Immunoblotting analyses were performed to determine the effects of genistein on ERα protein synthesis (Fig. 4). Exposure of MC3T3-E1 cells to 1 μm-genistein for 24 h did not affect the levels of ERα (Fig. 4(a), top panel, lane 2). In contrast, when the concentrations reached 5 and 10 μm, genistein increased ERα synthesis (lanes 3 and 4). Exposure of MC3T3-E1 cells to 10 μm-genistein for 0·5, 1, 3, 6 and 24 h time dependently enhanced the levels of ERα (Fig. 4(b)). The amounts of β-actin were immunodetected as internal controls (Fig. 4(a) and (b)).
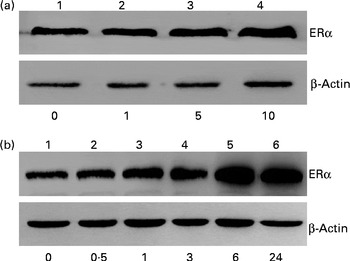
Fig. 4 Effects of genistein on the synthesis of oestrogen receptor (ER) α protein. MC3T3-E1 cells were exposed to (a) 1, 5 and 10 μm-genistein for 24 h or to (b) 10 μm-genistein for 0·5, 1, 3, 6 and 24 h. (a, b) ERα was immunodetected. The amounts of β-actin were analysed as internal controls.
Nuclear proteins were isolated and analysed to determine the effects of genistein on the translocation of ERα from the cytoplasm to the nucleus (Fig. 5). Exposure of MC3T3-E1 cells to 1 μm-genistein for 24 h increased the levels of nuclear ERα (Fig. 5(a), top panel, lane 2). After treatment with 5 and 10 μm-genistein, the amounts of ERα in the nuclei were significantly increased (lanes 3 and 4). Exposure of MC3T3-E1 cells to 10 μm-genistein for 0·5, 1, 3, 6, and 24 h enhanced the nuclear ERα levels in a time-dependent manner (Fig. 5(b), top panel, lanes 2–6). The amounts of β-actin were immunodetected as internal controls (Fig. 5(a) and (b)).
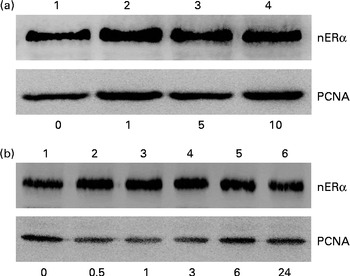
Fig. 5 Effects of genistein on the translocation of oestrogen receptor (ER) α from the cytoplasm to the nucleus (n). MC3T3-E1 cells were exposed to (a) 1, 5 and 10 μm-genistein for 24 h or to (b) 10 μm-genistein for 0·5, 1, 3, 6 and 24 h. The levels of nERα were immunodetected. The amounts of nuclear proliferating cell nuclear antigen (PCNA) were analysed as internal controls.
Rat calvarial osteoblasts were prepared to evaluate the effects of genistein on the expressions of ERα and cell differentiation-related genes (Fig. 6). Exposure of rat osteoblasts to 10 μm-genistein for 6 h induced ERα mRNA expression (Fig. 6(a), top panel, lane 2). The levels of nuclear ERα in rat osteoblasts were significantly increased following treatment with 10 μm-genistein for 24 h (Fig. 6(b), top panel, lane 2). The amounts of β-actin mRNA and protein were analysed as internal controls (Fig. 6(a) and (b)). A bioinformatic approach was performed, and the results indicated that 9, 10 and 10 ERα-specific DNA-binding elements, respectively, existed in the 5′-promoter regions of the BMP-6, Col I and OCN genes (Fig. 6(c)). After exposure to 10 μm-genistein for 6 h, the expressions of BMP-6, Col I and OCN mRNA were significantly induced (Fig. 6(d), top three panels, lane 2). β-Actin mRNA was analysed as the internal control.
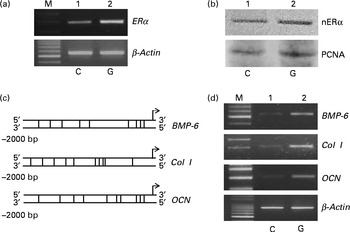
Fig. 6 Effects of genistein on the regulation of the expressions of the oestrogen receptor (ER) α, bone morphogenetic protein (BMP) 6, collagen type I (Col I) and osteocalcin (OCN) genes in primary rat osteoblasts. Rat osteoblasts prepared from rat calvaria were exposed to 10 μm-genistein for (a, d) 6 h and (b) 24 h. (a, d) The levels of ERα, BMP-6, Col I and OCN mRNA were determined using a RT-PCR. (a, d) β-Actin mRNA was analysed as an internal control. (b) The amounts of nuclear ERα (nERα) were immunodetected. Nuclear proliferating cell nuclear antigen (PCNA) was analysed as an internal control. A bioinformatic approach was used to search the ERα-specific DNA-binding elements in the 5′-promoter regions of the BMP-6, Col I and OCN genes. M, marker of 100 bp DNA ladder.
After exposure to a combined mixture of dexamethasone, ascorbic acid, and β-glycerophosphate or genistein for 21 d, osteoblast mineralisation was determined (Fig. 7). Treatment of primary rat calvarial osteoblasts with genistein alone did not affect cell mineralisation (Fig. 7(a)). However, when the osteoblasts were exposed to a mixture of dexamethasone, ascorbic acid and β-glycerophosphate for 21 d, mineralised nodules were obviously increased (Fig. 7(a)). In contrast, co-treatment with the DR and genistein synergistically increased osteoblast mineralisation (Fig. 7(a)). These mineralised nodules were quantified and analysed (Fig. 7(b)). In the control and genistein-treated rat osteoblasts, mineralised nodules were not observed. After exposure to the DR, the nodules were significantly produced. Compared with the DR-treated group, co-treatment with genistein and the DR resulted in a significant twofold increase in osteoblast mineralisation (Fig. 7(b)).
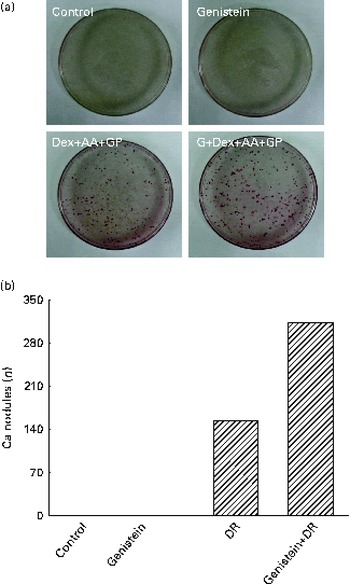
Fig. 7 Effects of genistein on the mineralisation of primary rat osteoblasts. Rat osteoblasts prepared from rat calvaria were exposed to 10 μm-genistein (G), a differentiation reagent (DR, including 10 nm-dexamethasone (Dex), 100 μg ascorbic acid (AA)/ml and 10 mm-β-glycerophosphate (GP)), and a combination of genistein and the DR for 21 d. The drugs were renewed every 2 d. (a) Osteoblast mineralisation was determined using the Alizarin red S dye-staining protocol. (b) The amounts of mineralised nodules were quantified and analysed.
Discussion
Genistein can induce ERα gene expression. The present study showed that after exposure to genistein the levels of ERα in MC3T3-E1 cells were increased in concentration- and time-dependent manners. At the same time, ERα mRNA expression was significantly increased by genistein. Thus, genistein-induced ERα gene expression occurred at least by a pre-translational mechanism. The genistein-induced ERα mRNA expression was also confirmed in primary osteoblasts isolated from rat calvaria. In addition, we demonstrated that genistein under the present administered conditions did not cause cytotoxicity in osteoblasts. Liao et al. ( Reference Liao, Xiao and Qin 28 ) reported that genistein can stimulate ERα mRNA expression in bone marrow cells. In the present study, we further showed that genistein can induce ERα mRNA and protein expressions in MC3T3-E1 cells and primary rat calvarial osteoblasts. We also demonstrated that ERβ mRNA expression can be induced by genistein. ERα and ERβ play vital roles in the mediation of oestrogen-involved regulation of skeletal growth and maintenance( Reference Sims, Clément-Lacroix and Minet 23 ). However, the levels of human serum oestrogen decrease with ageing( Reference Fiorelli and Brandi 12 , Reference Krum 14 ). Genistein, an isoflavone-type phyto-oestrogen, has been reported to have the potential to prevent and treat osteoporosis( Reference Usui 18 , Reference Coward, Barnes and Setchell 19 ). Osteoblasts contribute to bone formation( Reference Seeman and Delmas 1 , Reference Chen, Lin and Chou 2 ). Therefore, the present study further showed that genistein can induce ERα gene expression and possibly participate in the regulation of osteoblast activities and bone metabolism.
The translocation of NF-κB and AP-1 is involved in genistein-induced ERα gene expression. The amounts of nuclear NF-κB and c-Jun in the osteoblasts were time dependently up-regulated following genistein administration. c-Jun and c-Fos can bind to each other to form the heterodimeric AP-1, a transcription factor( Reference Hess, Angel and Schorpp-Kistner 32 ). The results of our bioinformatic search revealed that both NF-κB- and AP-1-specific DNA-binding elements exist in the 5′-promoter region of the ERα gene. Thus, genistein-induced ERα gene expression was due to the improved translocation of NF-κB and AP-1 from the cytoplasm to the nucleus. The increased translocation of these two transcription factors simultaneously increases their transactivation activities and induces ERα gene expression. NF-κB and AP-1 are two typical transcription factors that participate in the regulation of osteoblast differentiation and mineralisation( Reference Kim, Lee and Kim 33 , Reference Tan, Huang and Chang 34 ). Targeting NF-κB- and AP-1-transduced signals has been reported to be a promising strategy for treating bone diseases( Reference Wu, Li and Yang 35 ). Genistein-induced translocation of NF-κB and AP-1 can concurrently explain the induction of the expression of the ERα gene and its effects on the promotion of osteoblast activities and bone metabolism.
Genistein can trigger the phosphorylation of MAPK and enhance the translocation of NF-κB and AP-1. The present results revealed that exposure of osteoblasts to genistein caused time-dependent increases in the phosphorylation of ERK1/2, JNK1/2 and p38 MAPK. MAPK are serine/threonine-specific protein kinases containing three major enzymes, namely ERK1/2, JNK1/2 and p38 MAPK( Reference Manning, Whyte and Martinez 36 ). After phosphorylation, ERK1/2 and p38 MAPK can activate NF-κB and trigger the translocation of this transcription factor into nuclei, which subsequently induces the expressions of certain genes( Reference Karin 37 ). In contrast, the activated JNK1/2 can phosphorylate c-Jun and induce the transactivation activity of AP-1( Reference Shaulian 38 ). Accordingly, one of the major reasons explaining the translocation of NF-κB and AP-1 stimulated by genistein is the phosphorylation of ERK1/2, p38 MAPK and JNK1/2 by this phyto-oestrogen. In oestrogen-involved signalling, the activated ERK1/2 promotes ERα-mediated intracellular signal-transducing events( Reference Driggers and Segars 39 ). In addition, the phosphorylation of MAPK contributes to the regulation of bone remodelling( Reference Wu, Li and Yang 35 ). Therefore, genistein-induced phosphorylation of ERK1/2, JNK1/2 and p38 MAPK and subsequent activation of the transcription factors NF-κB and AP-1 could have multiple functions in the regulation of ERα gene expression and protein activation and improvement of osteoblast maturation.
Genistein stimulates the expressions of the BMP-6, Col I and OCN genes through the activation of ERα in primary rat osteoblasts. MC3T3-E1 is an osteoblast-like cell line. To confirm the effects of genistein on primary cells, osteoblasts were isolated from neonatal rat calvaria. After exposure to genistein, ERα mRNA and protein expressions were significantly induced. Thus, genistein can also induce ERα gene expression in primary osteoblasts as that observed in osteoblast-like MC3T3-E1 cells. ERα can act as a transcription factor in the regulation of osteoblast differentiation-related genes, including BMP-6, Col I and OCN ( Reference Dahlman-Wright, Cavailles and Fuqua 22 ). The present study showed that in parallel to the increase in the translocation of ERα into the nuclei, the expressions of BMP-6, Col I and OCN mRNA in primary rat osteoblasts were simultaneously induced. We also showed that several ERα-specific DNA-binding elements are present in the 5′-promoter regions of the BMP-6, Col I and OCN genes. Therefore, in primary osteoblasts, genistein can induce ERα mRNA expression and protein activation and stimulate the expressions of the BMP-6, Col I and OCN genes.
Genistein has synergistic effects on the stimulation of mineralisation of primary rat osteoblasts. The present study demonstrated that although genistein alone cannot induce the mineralisation of primary rat osteoblasts, co-treatment with a traditional DR synergistically promotes osteoblast maturation. Osteoblast differentiation and maturation are regulated by a complicated network of various proteins, including BMP, Col I and OCN( Reference Vandenput and Ohlsson 5 ). In osteogenesis, BMP-6 and Col I participate in bone extracellular matrix differentiation and mineralisation( Reference Trzeciakiewicz, Habauzit and Mercier 8 , Reference van Leeuwen, van Driel and van den Bemd 9 ). Col I contributes to osteoblast adhesion and differentiation( Reference Mathews, Bhonde and Gupta 11 ). Increases in the syntheses of these proteins will trigger osteoblast differentiation and mineralisation( Reference Vandenput and Ohlsson 5 , Reference Kugimiya, Kawaguchi and Kamekura 7 , Reference Mizuno and Kuboki 10 ). In the present study, genistein was shown to induce the expressions of the BMP-6, Col I and OCN genes. Therefore, genistein can promote osteoblast differentiation and bone metabolism by inducing the expressions of these cell differentiation-related genes.
In summary, the present study showed that exposure of MC3T3-E1 cells and primary rat osteoblasts to genistein did not cause cytotoxicity. In contrast, genistein induced ERα mRNA and protein expressions in MC3T3-E1 cells in concentration- and time-dependent manners. As regards the mechanisms, treatment with genistein time dependently increased the phosphorylation of ERK1/2, p38 MAPK and JNK1/2. Sequentially, the levels of nuclear NF-κB and c-Jun were significantly enhanced after genistein administration. In primary neonatal rat calvarial osteoblasts, the effects of genistein on ERα mRNA and protein expressions were also confirmed. Genistein can trigger the translocation of ERα from the cytoplasm to the nucleus. The results of a bioinformatic approach revealed that several ERα-specific DNA-binding elements exist in the 5′-promoter regions of the osteoblast differentiation-related BMP-6, Col I and OCN genes. In parallel, genistein induced the expressions of BMP-6, Col I and OCN mRNA in primary rat osteoblasts. Consequently, exposure to genistein synergistically improved the traditional ER-induced osteoblast mineralisation. In summary, the present study showed that genistein can induce ERα gene expression via the activation of MAPK/NF-κB/AP-1 and stimulate osteoblast differentiation and maturation through the ERα-dependent induction of the expressions of the BMP-6, Col I and OCN genes. The molecular mechanisms of genistein-involved regulation of the expressions of osteoblast differentiation-related genes, including BMP-6, Col I and OCN, are being studied in our laboratory. The effects of genistein on bone healing using an animal model of bone defects will be our next research focus.
Acknowledgements
The present study was supported by Wan-Fang Hospital (100-wf-eva-10) and the National Science Council (NSC101-2314-B-038-003-MY3), Taipei, Taiwan. All authors participated in the design of the present study. M.-H. L. and P.-I. L. carried out the cell culture and drug treatment. M.-H. L., Y.-T. T. and Y.-A. C. conducted the assays of cell viability and immunodetection. M.-H. L., Y.-T. T. and Y.-G. C. contributed to the RT-PCR analyses and osteoblast maturation. S.-H. L. and R.-M. C. participated in the data analyses. M.-H. L. and R.-M. C. wrote the initial manuscript draft. R.-M. C. assembled the final version. All authors read and approved the final manuscript. None of the authors has any conflict of interest to declare.