Introduction
The unique jalca landscape in Peru’s northern Andes is a high-altitude transition ecosystem between the páramo of the northern Andes and the puna of the central and southern Andes (Sánchez-Vega & Dillon Reference Sánchez-Vega, Dillon, Moraes, Øllgaard, Kvist, Borchsenius and Balslev2006, Britto Reference Britto2017). Here, the Andes are interrupted by the Huancabamba Depression, an area of lower altitude. Jalca landscapes are found at altitudes of 3100–4200 m (Sánchez-Vega & Dillon Reference Sánchez-Vega, Dillon, Moraes, Øllgaard, Kvist, Borchsenius and Balslev2006, Britto Reference Britto2017) and extend across five of Peru’s northern political departments: Amazonas, Lambayeque, Piura, Cajamarca and San Martín, covering c. 1 340 320.57 ha (MINAM 2019). Despite being part of the Tropical Andes Biodiversity Hotspot (Myers et al. Reference Myers, Mittermeier, Mittermeier, Da Fonseca and Kent2000), jalca remain largely understudied compared to their páramo and puna counterparts (Sánchez-Vega & Dillon Reference Sánchez-Vega, Dillon, Moraes, Øllgaard, Kvist, Borchsenius and Balslev2006, Ochoa-Tocachi et al. Reference Ochoa-Tocachi, Buytaert, De Bièvre, Célleri, Crespo and Villacís2016, Britto Reference Britto2017, Mosquera et al. Reference Mosquera, Marín, Stern, Bonnesoeur and Ochoa-Tocachi2022).
Jalca landscapes are herbaceous areas dominated by grasslands (pajonales), interspersed with shrubs and wetlands (Sánchez-Vega & Dillon Reference Sánchez-Vega, Dillon, Moraes, Øllgaard, Kvist, Borchsenius and Balslev2006, Cooper et al. Reference Cooper, Wolf, Colson, Vering, Granda and Meyer2010). Plant diversity is considered equal to or greater than that of the páramo and greater than the more southern puna (Sánchez-Vega & Dillon Reference Sánchez-Vega, Dillon, Moraes, Øllgaard, Kvist, Borchsenius and Balslev2006). Jalca and páramo grasslands have the richest tropical mountain flora in the world (Bremer et al. Reference Bremer, Farley, DeMaagd, Suárez, Cárate Tandalla, Vasco Tapia and Mena Vásconez2019). In the absence of glaciers in this area, these montane landscapes are considered the most important water sources in northern Peru (Buytaert et al. Reference Buytaert, Célleri, De Bièvre, Cisneros, Wyseure, Deckers and Hofstede2006, Mosquera et al. Reference Mosquera, Lazo, Célleri, Wilcox and Crespo2015). However, the hydrological functions of jalca remain understudied (Mosquera et al. Reference Mosquera, Lazo, Célleri, Wilcox and Crespo2015).
Since its international discovery in 2006 (BBC Mundo Reference BBC2006), the 771–m tall, two-tiered Gocta waterfall has helped transform Peru’s remote northern department of Amazonas into one of the country’s most important tourist destinations. In 2019, prior to the COVID-19 pandemic, the Gocta waterfall attracted over 59 000 tourists (MINCETUR/VMT/DGIETA – DIAITA 2020). Community members in nearby San Pablo de Valera and Cocachimba believe that the wetlands within the jalca landscape play an important role in ensuring year-round water flow to the waterfall. In páramo ecosystems in Ecuador, catchments with a higher cover of wetlands have higher water yields, and histosols (peat soils) are responsible for maintaining sustained flows throughout the year (Mosquera et al. Reference Mosquera, Lazo, Célleri, Wilcox and Crespo2015, Reference Mosquera, Segura, Vaché, Windhorst, Breuer and Crespo2016). Commonly referred to as bofedales in the Andes, these include a range of wetland types such as peatlands and wet meadows (Sánchez-Vega & Dillon Reference Sánchez-Vega, Dillon, Moraes, Øllgaard, Kvist, Borchsenius and Balslev2006, Cooper et al. Reference Cooper, Wolf, Colson, Vering, Granda and Meyer2010, Maldonado Fonkén Reference Maldonado Fonkén2014, Chimner et al. Reference Chimner, Bourgeau-Chavez, Grelik, Hribljan, Clarke and Polk2019) found between 3000 and 5500 m altitude (INAIGEM 2023).
Chimner et al. (Reference Chimner, Resh, Hribljan, Battaglia, Bourgeau-Chavez, Bowser and Lilleskov2023) compared carbon stocks in wetlands in the central Peruvian Andes, differentiating between peatlands and wet meadows. They found that peatlands store much more carbon (mean 1092 MgC ha–1) than wet meadows (mean 30 MgC ha–1), concluding that efforts to calculate carbon stocks in wetlands in the Andes should focus on peatlands rather than other wetland types. In the Peruvian lowland Amazon, peatlands store nearly 5.4 PgC, almost as much as the entire aboveground carbon stock of Peru, while only covering 5% of the country’s area (Hastie et al. Reference Hastie, Honorio Coronado, Reyna, Mitchard, Akesson and Baker2022). While peat only covers c. 3% of the world’s surface, it stores about a third of global soil carbon (Harenda et al. Reference Harendra, Lamentowicz, Samson, Chojnicki, Zielinski, Sagan and Surosz2018).
Anthropogenic effects on jalca ecosystems are complex; much of the information on this matter is derived from research on páramos and puna. López Gonzales et al. (Reference López Gonzales, Hergoualc’h, Angulo Núñez, Baker, Chimner and Del Águila Pasquel2020) list overgrazing, drainage and peat extraction as the main threats to peatland degradation in the Peruvian Andes. Andean páramos have undergone a historically widespread use of fire to encourage new grass growth for cattle (Buytaert et al. Reference Buytaert, Célleri, De Bièvre, Cisneros, Wyseure, Deckers and Hofstede2006, Borrelli et al. Reference Borrelli, Armenteras, Panagos, Modugno and Schütt2015, White-Nockleby et al. Reference White-Nockleby, Prieto, Yager and Meneses2021). The interaction between the deliberate burning of jalca and climate change is critical: although the hydrological ecosystem services of jalca wetlands are not greatly threatened by infrequent fire due to their high water content, climate change could lead to their gradual drying, thus increasing potential fire damage and threatening the wetlands’ ecosystem services (Buytaert et al. Reference Buytaert, Cuesta-Camacho and Tobón2011).
The effects of livestock grazing on the hydrological ecosystem services of jalca still need to be fully understood. The main type of domestic livestock in the jalca ecoregion is cattle (INEI 2012). Ochoa-Tocachi et al. (Reference Ochoa-Tocachi, Buytaert, De Bièvre, Célleri, Crespo and Villacís2016) showed that livestock overgrazing in jalca has a concerning impact on a catchment’s hydrological regulation capacity, while low-intensity grazing seems to have little impact.
Andean wetlands in general, including peatlands, have only been recently studied and mapped (Otto et al. Reference Otto, Scherer and Richters2011, Ochoa-Tocachi et al. Reference Ochoa-Tocachi, Buytaert, De Bièvre, Célleri, Crespo and Villacís2016, Otto & Gibbons Reference Otto and Gibbons2017, Chimner et al. Reference Chimner, Bourgeau-Chavez, Grelik, Hribljan, Clarke and Polk2019, Reference Chimner, Resh, Hribljan, Battaglia, Bourgeau-Chavez, Bowser and Lilleskov2023, Ross et al. Reference Ross, Mendoza, Drenkhan, Montoya, Baiker and Mackay2023), and they are clearly absent from the global wetland and peatland databases, such as PEATMAP and PEAT-ML (Xu et al. Reference Xu, Morris, Liu and Holden2018, Melton et al. Reference Melton, Chan, Millard, Fortier, Winton and Martin-Lopez2022), the CIFOR Global Wetlands Database (Chimner et al. Reference Chimner, Bourgeau-Chavez, Grelik, Hribljan, Clarke and Polk2019) or the global peatland map of Leifeld and Menichetti (Reference Leifeld and Menichetti2018). Only the Global Peatland Map 2.0 (Global Peatlands Initiative 2022) included a few peatland areas in the Andes, but none were from the jalca ecoregion. Complex topography, remoteness, high levels of cloud and the patchy distribution of Andean peatlands are challenges regarding their detection (Curatola Fernández et al. Reference Curatola Fernández, Obermeier, Gerique, López Sandoval, Lehnert and Thies2015, Chimner et al. Reference Chimner, Bourgeau-Chavez, Grelik, Hribljan, Clarke and Polk2019).
Current conservation strategies around Gocta aim to protect Gocta’s water flow to preserve this natural monument and also to secure alternative income for the nearby communities, which could finance local conservation efforts to protect priority biodiversity sites, including jalca and montane cloud forests. However, knowledge of the socio-ecological system upstream of the waterfall is needed to inform conservation planning. The current study sought to: (1) identify the drainage area that sustains the waterfall’s flow; and (2) identify land tenure and peatlands within the jalca-dominated drainage area. At the regional/global level, this study contributes to mapping and conserving peatlands in the Andean jalca and in other tropical mountain areas of the world.
Methods
Study area
The Gocta waterfall is found on the eastern escarpments of the north Peruvian Andes (Fig. 1) at 2500 m above sea level (top of the waterfall) in the Amazonas department. It is formed by the Cocahuayco River, an affluent of the Uctubamba River. The geology is represented by the Pucará group (Goyllarisquizga and Chonta formations), with a geomorphology of high Mesozoic calcareous mountains (Oliva et al. Reference Oliva, Pérez, Salas Lopez, Gamarra, Levia, Collazos and Maicelo2017). The climate is semi-humid and warm temperate (Yalta Meza et al. Reference Yalta Meza, Salas Lopez, Pérez Torres and Quispe Chacón2014). There are two main seasons: the wet season (approximately November–April, with higher rainfall from January to March) and the dry season (approximately May–October, with lower rainfall from June to August; Rascón et al. Reference Rascón, Coroto, Tafur and Torres2021, Leiva-Tafur et al. Reference Leiva-Tafur, Goñas, Culqui, Santa Cruz, Rascón and Oliva-Cruz2022).

Figure 1. Study area in the northern Peruvian Andes. The map depicts the geographical domain of the satellite scenes used for the peatland classification. Within this area, the vegetation classes of the National Map of Peruvian Ecosystems (MINAM 2019) are shown. Yunga is the local name given to the eastern escarpments of the Peruvian Andes between 600 and 3200 m altitude. The class ‘secondary vegetation’ denotes deforested areas that are either grasslands or fallow.
The waterfall and its catchment are dominated by two ecosystems: montane cloud forests and highland jalca above the waterfall (>2800 m altitude; Fig. 2).
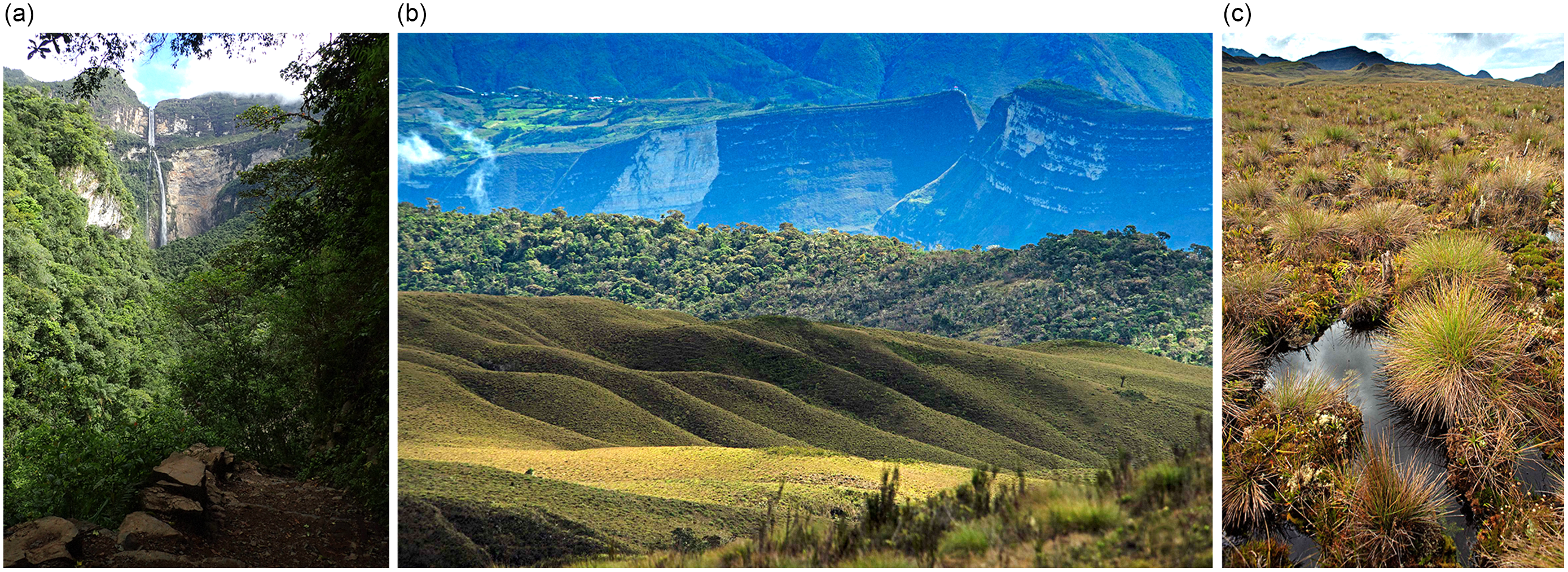
Figure 2. Images of the study area. (a) Gocta waterfall surrounded by montane cloud forests (photo by GF Curatola Fernández). (b) Landscape of the drainage area of the Gocta waterfall composed of jalca vegetation and montane cloud forests (photo by WH Wust). (c) Peatlands in the Gocta waterfall drainage area (photo by WH Wust).
The jalca landscape that dominates the plateau above the waterfall is characterized by bunch grass species, including the genera Calamagrostis, Poa, Festuca, rosette plants of Puya, woody species of Senecio and Asteraceae (Cooper et al. Reference Cooper, Wolf, Colson, Vering, Granda and Meyer2010). Large mammals include Andean bear Tremarctos ornatus and white-tailed deer Odocoileus virginianus (Gobierno Regional de Amazonas 2013, Meza Mori et al. Reference Meza Mori, Barboza Castillo, Torres Guzmán, Cotrina Sánchez, Guzman Valqui and Oliva2020).
Various types of montane forests exist at 1800–2900 m altitude, and these are home to the largest diversity of fauna in the area (Gobierno Regional de Amazonas 2013, Cervera Reference Cervera2020), such as the critically endangered yellow-tailed woolly monkey Lagothrix flavicauda (Shanee et al. Reference Shanee, Cornejo, Aquino, Mittermeier and Vermeer2019) and the endangered marvelous spatuletail hummingbird Loddigesia mirabilis (Gobierno Regional de Amazonas 2013, BirdLife International 2016). Thirty-six endemic species are present, including 12 amphibians, 6 reptiles, 12 birds and 6 mammals (Torres Guzmán et al. Reference Torres Guzmán, García-Bravo and Chuquizuta2022). Between 1800 and 2200 m, there is also ample secondary growth forest and shrublands (Oliva et al. Reference Oliva, Pérez, Salas Lopez, Gamarra, Levia, Collazos and Maicelo2017), interspersed with pine plantations, urban areas and shrubs (vegetation succession after agricultural land abandonment). The most intact forests are those close to the waterfall, but deforestation is increasing due to poorly regulated urban development and agriculture.
The waterfall is accessible from Cocachimba and San Pablo de Valera (Fig. 1). Since 2006, Cocachimba in particular has relied heavily on tourism compared to traditional sources of income from agriculture and livestock, and this has resulted in rapid urban development, with local people selling land parcels to earn money and finance the development of tourism-related businesses. In San Pablo de Valera, the land around the waterfall, including part of the waterfall drainage area, is owned communally by the campesino (rural farmer) community, which makes collective decisions on land use. Very little land has been sold to private developers, resulting in slower tourism growth and allowing the community to create the 2603.57–ha San Pablo–Catarata Gocta Privately Protected Area (PPA) in 2019.
The main human disturbances in the Gocta drainage area are forest clearing in the montane cloud forest areas to create pastures for horses or agricultural land and, in the higher jalca landscape, burning of pajonales to encourage new growth for cattle grazing. There has been some afforestation with non-native pine trees in the highland area. These threats have also been identified in more extensive studies of Andean grasslands (Buytaert et al. Reference Buytaert, Célleri, De Bièvre, Cisneros, Wyseure, Deckers and Hofstede2006, Ochoa-Tocachi et al. Reference Ochoa-Tocachi, Buytaert, De Bièvre, Célleri, Crespo and Villacís2016).
Remote sensing and spatial data
We used satellite data subsets covering an area of 7132.86 ha spanning from c. 6.0° to 5.9°S and from 77.9° to 77.8°W (Fig. 1). Two freely accessible digital elevation models (DEMs) were acquired (Table 1). The ASTER GDEM v2 images were pre-processed using QGIS 3.16.9 and later used for catchment delimitation. A topographical position index (TPI) was calculated using the ‘TPI Based Landform Classification’ tool relying on the ALOS PALSAR DEM. The TPI classifies the shape of the land according to its degree of concavity or convexity. These terrain morphologies are determinants of the formation of peatlands.
Table 1. Characteristics of the satellite imagery used in this study.

DEM = digital elevation model; L1 GRD IW = level-1 ground range detected interferometric wide swath; IR = infrared; NIR = near-infrared; SAR = synthetic-aperture radar; SWIR = short-wave infrared; T1 = tier 1; TIR = thermal infrared.
We used a Landsat 8 image (Table 1), which was chosen due to it being cloud-free and captured close to the first date of field data collection (August 2019). To pre-process the Landsat data, we followed Chimner et al.’s (Reference Chimner, Bourgeau-Chavez, Grelik, Hribljan, Clarke and Polk2019) methodology. The Normalized Difference Vegetation Index (NDVI), which provides information on the state of greenness and biomass of the vegetation, was calculated using the red and near-infrared bands according to Rouse et al. (Reference Rouse, Haas, Schell and Deering1974).
We used a radar image from the Sentinel 1B satellite, which we pre-processed using SNAP 8.0 software (Leskovec & Sosic Reference Leskovec and Sosic2016), partially following the methodology of Filipponi (Reference Filipponi2019). We applied the orbit file to improve the information on the position and speed of the satellite, followed by thermal noise removal and scene calibration. Next, we applied a ‘multilook’ correction, lowering the resolution from 10 to 30 m (Landsat and DEM image resolution). To correct the radiometric variability associated with the topography, we performed a bilinear interpolation. We then applied a dot filter to improve image quality and reduce granular noise caused by the interference of reflected waves from various elemental scatterers. Finally, we used the ‘Range Doppler Terrain Correction Operator’ for topographical correction.
For visual support, we used a cloud-free high-resolution image from PeruSat-1 (Table 1). To improve the visual quality of the PeruSat-1 image, we performed an intensity–hue–saturation pan-sharpening.
We also collected the following vector data: (1) water network for the Cocahuayco river basin (Yalta Meza et al., Reference Yalta Meza, Salas Lopez, Pérez Torres and Quispe Chacón2014); (2) boundaries of the San Pablo de Valera PPA (SERNANP 2023); (3) boundaries of the properties of the town of Cocachimba (requested from the National Superintendence of Public Registries; SUNARP); (4) areas of campesino communities (COFOPRI 2023); and (5) human population centres (MED 2023).
Field data
Two field expeditions were carried out in August 2019 and 2021. Cartographic data were gathered through participatory mapping to delimit land-use areas of communities/owners. Soil cores were sampled to identify the presence/absence of peat in the study site.
Prior to field expeditions, we presented the project to representatives of the campesino community of San Pablo de Valera and the community of Yurumarca. Both communities gave permission to collect soil samples within their territories and chose representatives to participate in the expedition. Printed A1 maps were used in the field and shown to community representatives. These maps included a background image, contour lines, water network, populated centres, limits of campesino communities and private properties. Through visual inspection of a PeruSat ‘pansharpen’ image and the contour lines derived from ASTER GDEM v2, potential peat areas were identified and uploaded onto a GPS to guide soil sample collection. During the expedition, we recorded the GPS coordinates of the land-tenure and land-use boundaries recognized by representatives of the community authorities.
Thirty-three soil cores of 40–cm depth were collected in places with homogeneous vegetation cover in a minimal radius of 30 m from the collection point. Each sample was stored in a numbered zip-lock bag. At each site, we also recorded the GPS coordinates and took photos in the four cardinal directions using the EpiCollect 5 smartphone app (Aanensen et al. Reference Aanensen, Huntley, Feil, Al-Own and Spratt2009). The soil samples were analysed at the Soil and Water Research Laboratory (LABISAG) of the Universidad Nacional Toribio Rodríguez de Mendoza (UNTRM) in Amazonas. Refrigeration was unnecessary because samples were processed the day after the field expeditions. To calculate the percentage of organic matter, which determines the presence or absence of peat in the sampling points, we followed the methodology of Chambers et al. (Reference Chambers, Beilman and Yu2011). The samples were dried at 40°C for 1 week, then 0.5–g samples were extracted, homogenized and incinerated at 500°C for 4 h. All of the samples had 40 cm of continuous organic material. However, those with at least 24% of organic matter (>12% organic carbon) were considered peat, following the definition by the United States Soil Taxonomy Staff (2014) that was used by Chimner et al. (Reference Chimner, Bourgeau-Chavez, Grelik, Hribljan, Clarke and Polk2019) for classifying peatlands in the central Peruvian Andes.
Waterfall drainage area delimitation and peatland detection
We delimited the Cocahuayco river basin and then the Gocta waterfall drainage area in QGIS, relying on the pre-processed ASTER GDEM v2. Validation was done by visual inspection using the contour lines derived from the DEM and the water network. Some corrections were necessary, which we manually digitized. Due to the karstic nature of the area, the morphological delimitation of the waterfall drainage area may differ slightly from the hydrological basin.
We classified peatlands using the random forest classifier in the R software’s (v.3.6.3) ‘caret’ and ‘randomForest’ packages. Spectral data for thematic class definition were derived from Landsat 8, Sentinel 1 and ALOS PALSAR satellite imagery. Random forest was executed using 500 decision trees (ntree) and three variables randomly sampled at each split (mtry) because we found these to be the parameter values delivering the most accurate results through the algorithm’s parameterization. We classified areas in two steps: first, those covered by low vegetation (peatlands, wet meadows and grasslands) were separated from other land-cover types, such as forest, water and bare soil (overall accuracy = 99.57%; Kappa hat = 0.99); and second, based on the results from the first step, we applied a spatial mask to the spectral input layers before running a second classification, so that peatland and non-peatland pixels were exclusively classified in the area covered by low vegetation.
For the peatland/non-peatland classification, we created training sites based on information from ground truth data (field observation and laboratory analysis of soils) and visual interpretation of satellite imagery. First, 60–m × 60–m training polygons centred at the GPS points taken during the field expeditions were generated; these dimensions correspond to twice the spatial resolution of the satellite data. Second, given the large area, the unevenness of the relief and the limited time available in the field, not all areas in the satellite scenes used in the study were covered during field sampling; to fill this gap, we digitized additional training sites through visual interpretation supported by field observations, to include underrepresented locations of the study area. Finally, all training areas were parcelled into 30–m × 30–m square polygons (1049 in total). Approximately 20% (210 polygons) of the training data were left out for validation. For each class, we calculated user, producer and overall accuracy. We also calculated the 95% confidence interval for the overall accuracy rate and estimated the peatland area error at the 95% confidence interval using the confusion matrix, with the predicted area as an uncertainty measure, following Oloffson et al. (Reference Olofsson, Foody, Stehman and Woodcock2013).
Based on the validated peatland map at 30–m spatial resolution, we calculated the area of peatlands contained within the territories of each landowner and campesino community by overlaying the mapped peatlands and the land-tenure boundary GIS layer.
Results
The drainage area of the Gocta micro-basin was estimated to be 3152 ha, of which peatland covered 398 ha (c. 18% of the overall drainage area). We found peatland at altitudes between 2800 and 3200 m. We delimited informal land tenure of the Yurumarca community within the Gocta sub-basin (see Fig. 3).

Figure 3. Peatlands and land-tenure/land-use map of the Gocta waterfall drainage area. Peatlands (dark green) were detected using the random forest algorithm, trained with multispectral, radar and digital elevation model-derived spectral data. Land tenure/land use was delineated by combining information from public databases and participatory mapping during fieldwork.
Land tenure in the study area is complex (see Fig. 3). The western part of the drainage area belongs to the San Pablo de Valera campesino community and includes their communal PPA. A smaller portion on the plateau’s southern edge is owned by a family from Cocachimba. The eastern section is used as pasture by the Yurumarca community without formal land tenure. In the north and north-east of the Gocta drainage area, small areas are owned by the Cuispes and San Carlos campesino communities.
The San Pablo de Valera PPA covers 34% of the Gocta drainage area and contains 46% of the peatlands; the property of the family from Cocachimba occupies 10% of the area but contains 23% of the total peatlands. A small area in the north-west of the sub-basin contains 3% of the drainage area and 6% of the peatlands and belongs to the San Carlos campesino community. The property of the Cuispes campesino community contains 2% of the area and 0.5% of the peatlands. The remaining 49% has no formal owner, and participatory mapping processes showed that it is used mainly by the community of Yurumarca (47% of the drainage area and c. 24% of the peatland). The remaining 4% of the drainage area consists of a strip of land without property or known informal use on a slope between the PPA and the property of the Quintana family, containing 0.5% of the peatland.
The overall accuracy was 97.14% (95% confidence interval = 93.89–98.94%), with producer and user accuracies of 95.75% and 97.27% for the ‘peatland’ class and 99.36% and 99.00% for the ‘non-peatland’ class, respectively. The total classified peatland area was 859.14 ha; however, the error-adjusted estimate of the peatland area (±95% confidence interval) according to the method of Oloffson et al. (Reference Olofsson, Foody, Stehman and Woodcock2013) was 872.6 ± 78.8 ha.
Discussion
To our knowledge, this is the first map of peatlands within the jalca ecoregion obtained via remote sensing. The methodological approach initially developed by Chimner et al. (Reference Chimner, Bourgeau-Chavez, Grelik, Hribljan, Clarke and Polk2019), but using only one scene of Sentinel data, proved to be accurate and easily replicable for mapping these peatlands. Our results have three key implications for conservation strategies in the Gocta drainage area, offering a globally relevant use of remote sensing to inform community-based conservation in a complex land-tenure setting.
First, a decade ago, the Tourism Association of Cocachimba attempted a payment for ecosystem services (PES) scheme with the community of Yurumarca (Daniel Quintana, personal communication, 19 October 2021). The agreement failed due to a lack of precise parameters, such as the area to be protected and monitoring processes (Daniel Quintana, personal communication, 19 October 2021). Indeed, in a global review of PES, problems with conditionality, and hence noncompliance, were identified by Wunder et al. (Reference Wunder, Börner, Ezzine-de-Blas, Feder and Pagiola2020) as some of the main obstacles to successful PES schemes. Our results suggest that a more effective conservation strategy would be an agreement between the Tourism Association of Cocachimba, which receives most of the waterfall tourism income but does not own land in the drainage area, and the campesino community of San Pablo de Valera to support the protection of peatlands within their PPA.
Our results underscore the importance of strengthening the San Pablo de Valera PPA. The findings complement initial zoning, which did not distinguish peat-containing wetlands within the jalca. The lack of delineation of wetlands and peatlands from other ecosystems within a jalca landscape makes it challenging to plan protection efforts within protected areas, including PPAs (López Gonzales et al. Reference López Gonzales, Hergoualc’h, Angulo Núñez, Baker, Chimner and Del Águila Pasquel2020). Our results now allow the community to exclude cattle more carefully from the peatland areas.
Second, we show that approximately a fifth of the peatland is within a private property currently used for cattle grazing (Daniel Quintana, personal communication, 19 October 2021). This 90-ha area could be conserved via a private conservation mechanism.
Third, the Municipality of Valera has already used the data for its urban development plan, an essential spatial planning process that will underpin the district’s sustainable development. Based on our and other data, the municipality has proposed that 10 276 ha in the Valera district and the neighbouring San Carlos district be prioritized for biodiversity conservation and protection of hydrological ecosystem services. The municipality is pioneering planning efforts in Amazonas: among the 1874 districts in Peru, fewer than 10% have an urban development plan (Requena Calderón & Absi Drobkova Reference Requena Calderón and Absi Drobkova2021).
Our study demonstrates an accessible methodological approach employing freely available satellite imagery, field sampling and open-sourced statistical models, which can be replicated in other jalca landscapes and used to create a regional map of peatlands of this part of Peru. According to Maldonado Fonkén (Reference Maldonado Fonkén2014), the jalca peatlands are poorly reported, and, indeed, jalca peatlands were omitted from the recent National Inventory of Bofedales (INAIGEM 2023). The areas where they occur were filtered out by the criteria used for the macrozones for bofedal classification (INAIGEM 2023), which was all areas below 3000 m altitude, while we found areas dominated by peatlands above 2800 m.
The methodology we applied delivered very accurate results for peatland mapping at the catchment scale (overall accuracy of 97.14%). The combination of several covariates, as Minasny et al. (Reference Minasny, Berglund, Connolly, Hedley, de Vries and Gimona2019) recommended, including optical, radar and topographical covariables, positively trained the random forest machine learning algorithm. A similar methodology was successfully used to map wetlands and peatlands in the Andes (Hribljan et al. Reference Hribljan, Suarez, Bourgeau-Chavez, Endres, Lilleskov and Chimbolema2017, Chimner et al. Reference Chimner, Bourgeau-Chavez, Grelik, Hribljan, Clarke and Polk2019, Ross et al. Reference Ross, Mendoza, Drenkhan, Montoya, Baiker and Mackay2023) and Indonesia (Rudiyanto et al. Reference Rudiyanto, Minasny, Setiawan, Saptomo and McBratney2018). The need for such methods is internationally relevant. In a review of the challenges for wetland biodiversity monitoring in Africa, where data availability poses a similar problem as in our study area, Stephenson et al. (Reference Stephenson, Ntiamoa-Baidu and Simaika2020) underscore the potential of scaling up remote sensing solutions to support wetland conservation.
The resulting peatland map is also of global importance because coarse-scale peatland maps do not capture local peatlands, given their patchy distribution and small area (Minasny et al. Reference Minasny, Berglund, Connolly, Hedley, de Vries and Gimona2019). This is especially true in the Huancabamba Depression, where the geography lacks the flat plains (punas) and glacial valleys of Peru’s central cordillera (Chimner et al. Reference Chimner, Bourgeau-Chavez, Grelik, Hribljan, Clarke and Polk2019).
Understanding the distribution and area of peatland and other wetlands within the jalca ecoregion would create opportunities at national and international scales.
It could allow authorities to improve the planning of new protected areas focused on hydrological ecosystem services or carbon sequestration, given the importance of peatlands in this respect (Buytaert et al. Reference Buytaert, Célleri, De Bièvre, Cisneros, Wyseure, Deckers and Hofstede2006, Mosquera et al. Reference Mosquera, Marín, Stern, Bonnesoeur and Ochoa-Tocachi2022). Currently, only 0.139% of the jalca (178 992.99 ha) is protected at the national level, and even less is protected in Amazonas (0.027%, 34 542.7 ha; SERNANP 2023). In addition, peatlands in the jalca ecoregion are essential for climate change mitigation. Although there is increasing global understanding of the importance of peatlands for carbon storage (Harenda et al. Reference Harendra, Lamentowicz, Samson, Chojnicki, Zielinski, Sagan and Surosz2018), Peru has yet to integrate peatlands into its national strategies for reducing and compensating greenhouse gas emissions through carbon credit systems (López Gonzales et al. Reference López Gonzales, Hergoualc’h, Angulo Núñez, Baker, Chimner and Del Águila Pasquel2020). This is relevant for PPAs in Peru in particular. Peru is one of few South American countries to allow for campesino communities such as those of San Pablo de Valera to create PPAs. However, PPAs lack tangible support from the government despite their conservation successes (Shanee et al. Reference Shanee, Shanee and Horwich2015, Delgado et al. Reference Delgado, Meza Mori, Barboza, Rojas Briceño, Torres Guzmán and Oliva-Cruz2021, López de la Lama et al. Reference López de la Lama, Bennet, Bulkan, Boyd and Chan2023). Integrating PPAs into Peru’s climate strategies while focusing not only on forests but also on peatlands and other wetlands could broaden the country’s means for addressing the climate crisis, contributing to the fight against global warming.
Andean peatlands have hitherto received less attention than Amazonian peatlands. Our study has attempted to bridge some of these gaps in the jalca ecoregion, thereby contributing to local, national and international conservation efforts in this part of the Tropical Andes Biodiversity Hotspot.
Acknowledgements
Our thanks go to the campesino communities of San Pablo de Valera and Yurumarca and the Tourism Association of Cocachimba for providing access to and information about the study area. Special thanks are due to Andrés Ramos Vargas, Luis Sopla Ramos, Alex Vargas Pinedo, Daniel Quintana, Linder Torrejón, Giulio Sangiorgi, Walter Wust, Francisco Meléndez and Nacho Sarmiento for their additional logistical and technical support, and to Prof Rodney A Chimner, Bruno Monteferri, Caroline Meyer and the Naturaleza y Cultura Internacional (NCI) team in Chachapoyas for their valuable advice. Our thanks also go to the Universidad Nacional Toribio Rodríguez de Mendoza de Amazonas for providing access to their laboratory. Special thanks go to the anonymous reviewers and the journal editors for excellent comments, which allowed us to significantly improve our manuscript.
Author contributions
Study design: GFCF, SMG, CS. Fieldwork: GFCF, SMG, EDF, ECV, CB, CS. Soil sample analysis: JR, EDF, ECV. Data analysis: GFCF, SMG, EDF, PR, ECV. Writing: GFCF, SMG, RSL, MO-C, CS. Maps and tables: GFCF, EDF, PR.
Financial support
This study was funded through a Global Forest Watch Tech Fellowship to Christel Scheske and grants to the SPDA from the US Fish and Wildlife Service and Fondation Ensemble.
Competing interests
The authors declare none.
Ethical standard
All field expeditions to the Gocta drainage basin were conducted with express consent from the San Pablo de Valera campesino community and the Yurumarca community. Leaders of both communities accompanied the expeditions. The results of the study have been shared with both communities and the Tourism Association of Cocachimba.