Obesity is now recognised as a chronic progressive disease, by several professional organisations and countries(1–3). The causes of obesity are multifactorial and include genetics, metabolic conditions, drugs, socioeconomic and environmental factors(Reference Busetto, Sbraccia and Vettor4). Obesity is defined by the WHO as an ‘abnormal or excessive fat accumulation that presents a risk to health’. BMI, a person's weight measured in kilograms divided by the square of their height in metres, is used as a marker of adiposity. Individuals with a BMI of 25 kg/m2 or more, are classified as being overweight, whereas people with a BMI of 30 kg/m2 or more as having obesity(5). Obesity is associated with multiple life-limiting diseases, including type 2 diabetes (T2D), CVD, certain types of cancers, reducing quality of life(Reference Stephenson, Smith and Kearns6) and life expectancy of up to 3–13 years, depending on the severity, comorbidities and other risk factors(Reference Peeters, Barendregt and Willekens7).
Until recently, obesity management has focused mainly on personal responsibility and ‘eating less and moving more’(Reference Busetto, Sbraccia and Vettor4), this simplistic view assumes incorrectly that body weight regulation is entirely under volitional control. This narrative has resulted in weight stigma and difficulty in accessing evidence-based treatments such as anti-obesity medications and bariatric surgery(Reference Rubino, Puhl and Cummings8). However, this narrative is changing as a result of our increased scientific understanding of the complexities of body weight regulation, which has led to obesity being reframed as a disease or chronic medical condition.
Ensuring adequate intake of nutrients is essential for survival. Therefore, it is hardly surprising that we have developed multiple adaptive physiological mechanisms that drive energy intake to protect us against famine(Reference Yu, Vasselli and Zhang9). The homoeostatic regulation of body weight involves a myriad of signals from the periphery that inform the brain about acute energy intake and energy stores. Orexigenic and anorexigenic signals from the gastrointestinal tract, pancreas and adipose tissue, modulate central neural activity primarily within the hypothalamic and brainstem regions and regulate hunger, satiety and eating(Reference Badman and Flier10). These homoeostatic pathways drive the feeling of hunger. However, in our current environment, most people eat in the absence of hunger due to the presence of food cues, for emotional and social reasons and because eating is pleasurable and rewarding.
Over the past 30 years it is become clear that the gastrointestinal tract plays a key role in regulating energy and glucose homoeostasis through releasing a panoply of gut hormones, bile acids, microbiome and enteric nervous signalling. The advent of brain functional imaging, for example functional MRI, of the brain has provided critical insights into how body weight is regulated in human subjects and has revealed that gut hormones and adiposity signals modulate neural activity within homoeostatic and brain reward regions. Moreover, functional brain imaging has enabled changes within the brain in response to weight loss interventions to be characterised.
In an environment where food availability was limited, there was an evolutionary survival advantage for those with a genetic profile that encouraged a large food intake when food was present and low activity, and reduced energy expenditure during times of food scarcity(Reference Yu, Vasselli and Zhang9). Our environment has since changed to ‘obesogenic’ where highly palatable energy-dense food is readily available coupled with a sedentary lifestyle, yet our innate mechanisms that regulate and defend body weight have remained the same. This means that the systems that defending us from starvation are now driving the obesity pandemic.
Our understanding of the genetic architecture of body weight regulation has also improved and it is established that 40–70 % of variation in body weight is heritable(Reference Stunkard, Harris and Pedersen11–Reference Bouchard and Tremblay15). Moreover, constitutionally thin people have fewer genetic variants known to increase the risk of becoming overweight(Reference Riveros-McKay, Mistry and Bounds16). However people with obesity have an increased number of genetic variants linked to becoming overweight(Reference Harbron, van der Merwe and Zaahl17).
Once an individual develops obesity, their biology alters and predisposes them to further weight gain. These changes include alterations in circulating gut hormones, leptin and insulin resistance(Reference Rosenbaum and Leibel18), altered gut microbiome(Reference Rosenbaum and Leibel18–Reference Maruvada, Leone and Kaplan20), changes in brain structure and altered connectivity in homoeostatic and hedonic brain regions involved in energy regulation, eating behaviour and food reward(Reference Stopyra, Friederich and Lavandier21,Reference Cornier, Von Kaenel and Bessesen22) . Therefore, these pathophysiological factors are prime targets of pharmaceutical modification to reduce energy intake and body weight.
Weight loss leads to improved health and quality of life for individuals with obesity. However, despite the increasing prevalence of obesity and its related comorbidities, there are limited treatment options available for weight management compared to other diseases(Reference Enebo, Berthelsen and Kankam23,Reference Ruban, Stoenchev and Ashrafian24) . Guidelines for weight management follow a stepwise approach that begins with lifestyle intervention with dietary restriction, increased physical activity and modification of eating behaviour for those with a BMI ≥ 25 kg/m2(Reference Yumuk, Tsigos and Fried25). Lifestyle intervention is the cornerstone for all weight management strategies and weight loss of 5–10 % reduces the risk of developing T2D(Reference Look, Pi-Sunyer and Blackburn26) and leads to improvement in some comorbidities such as non-alcoholic fatty liver disease(Reference Hsu, Ness and Kowdley27) and hypertension(Reference Wing, Lang and Wadden28). However, for the majority of people with severe obesity, lifestyle interventions lead to insufficient sustained weight loss to impact the majority of their obesity-related health conditions.
Weight loss maintenance is challenging due to powerful biological compensatory responses that are activated in response to ‘dieting’, which is the modern day equivalent of famine, and weight loss. As soon as person starts to lose weight there is an increase in the circulating levels of orexigenic hormone ghrelin, a reduction in satiety hormones (peptide YY and glucagon-like peptide-1 (GLP-1)) and adiposity signals such as leptin and insulin(Reference Evert and Franz29–Reference Sumithran, Prendergast and Delbridge31) and enhanced brain response to high-energy food cues in homoeostatic and reward brain region(Reference Rosenbaum, Sy and Pavlovich32,Reference Hinkle, Cordell and Leibel33) . These changes lead to increased hunger, reduced satiety and increased drive to eat. In addition, resting energy expenditure is reduced. These compensatory mechanisms typically result in a weight loss plateau, and ultimately progressive weight regain, sometimes greater than the baseline weight(Reference Hall and Kahan34) (Fig. 1). A meta-analyses revealed that in individuals who underwent lifestyle intervention for weight loss at a 5-year follow-up >80 % of weight that was lost was regained(Reference Anderson, Konz and Frederich35). For this reason, pharmacotherapy is recommended as an additive treatment to a lifestyle intervention for those with a BMI ≥ 30 kg/m2, or BMI ≥ 27 kg/m2 with an obesity-related comorbidity, where lifestyle intervention alone has proved insufficient.

Fig. 1. Illustration depicting the powerful biological compensatory mechanisms that occur in response to ‘dieting’ that predispose to weight regain.
The history of pharmacotherapy for obesity management has been marred by limited efficacy and safety issues. For example fenfluramine was associated with valvular defects(Reference Connolly, Crary and McGoon36) and primary pulmonary hypertension(Reference Douglas, Munro and Kitchin37), rimonabant with severe depression and suicide(Reference Christensen, Kristensen and Bartels38), sibutramine with increased risk of stroke and non-fatal myocardial infarction(Reference James, Caterson and Coutinho39) and lorcaserin with increased cancer risk(40).
Anti-obesity medication must achieve a 5 % minimum weight loss to be considered for licensing by the European Medicines Agency or Food and Drug Administration (FDA). Until recently, approved anti-obesity medications have been limited in efficacy with between 5 and 10 % weight reduction at 1 year. Although this degree of weight loss is accompanied by improvement in cardiometabolic risk factors(Reference Magkos, Fraterrigo and Yoshino41), this is insufficient to lead to remission of T2D or improvement of many obesity-related comorbidities such as obstructive sleep apnoea(Reference Foster, Borradaile and Sanders42) and osteoarthritis(Reference Riddle and Stratford43) where ≥15 % weight loss is required. Thus, leaving a treatment gap between pharmacotherapy and weight loss achieved with bariatric surgery.
Bariatric surgery is currently the most effective treatment for people with severe obesity, leading to marked sustained weight loss with unparalleled health benefits. One study showed that at a 4-year follow-up, patients with an average BMI of 47⋅7(sd 7⋅8) kg/m2 who underwent Roux-en-Y bypass had lost an average of 27⋅5 % (95 % CI 23⋅8, 31⋅2) of their baseline weight, and at 10 years, 71⋅8 % maintained >20 % weight loss(Reference Maciejewski, Arterburn and Van Scoyoc44). This degree of weight loss is associated with resolution of most diseases related to obesity, such as T2D(Reference Yska, van Roon and de Boer45) and non-alcoholic steatohepatitis(Reference Mummadi, Kasturi and Chennareddygari46).
Despite its high efficacy, access to bariatric surgery is limited, also many patients are reluctant to undergo an invasive procedure and would prefer an alternative more convenient treatment option such as pharmacotherapy, and lastly there is a small risk of surgery-related complications. As a result, an estimated 3⋅6 million people in England are eligible for bariatric surgery, however, figures reveal that an average 5000–6000 surgeries a year are being performed(Reference Desogus, Menon and Singhal47).
Research into how bariatric surgery mediates its sustained weight loss effects has provided us with novel insights into the physiological regulatory mechanisms of appetite, metabolism and eating behaviour(Reference Ionut, Burch and Youdim48). Although the precise mechanisms mediating the sustained weight loss effect of bariatric surgery remain to be determined, altered signalling from the gastrointestinal tract to the brain, as a consequence of changing nutrient and/or biliary flow through the gut, plays a critical role. In contrast to dieting, bariatric surgery leads to favourable changes in gut hormones with a marked increase in circulating satiety hormones, improved microbiome and reduced brain response to food cues in reward regions.
Scientific efforts have focused on trying to mimic the effects of bariatric surgery medically, on the premise that approaches that target the body's own appetite regulating systems were likely to be more efficacious and, more importantly, safer. The remainder of this review will focus on advancements achieved in gut hormone-based anti-obesity medications.
Glucagon-like peptide-1 receptor analogues for weight management
Liraglutide
Pharmacotherapies that target GLP-1 receptors are widely used for T2D treatment due to its endogenous role in regulating insulin and glucagon secretion and glucose homoeostasis in response to nutrient intake(Reference Meloni, DeYoung and Lowe49). Liraglutide is a human GLP-1 receptor analogue (GLP-1RA) and was initially approved by the FDA in 2010 for T2D therapy at 1⋅8 mg(50). The additional benefit of GLP-1 to induce appetite suppression (via activation of hindbrain and hypothalamus) and delayed gastric emptying and motility(Reference Alruwaili, Dehestani and Le Roux51) led to its translation into an FDA-approved anti-obesity medication at an increased dose of 3⋅0 mg(52). Endogenous GLP-1 has a short circulating half-life of 1–2 min(Reference Hui, Farilla and Merkel53), whereas liraglutide has been modified to have a half-life of 13–15 h allowing for administration once daily via subcutaneous injection(Reference Rubino, Greenway and Khalid54).
Liraglutide 3⋅0 mg was approved by the FDA in 2014 for weight management, when used as an adjunct to lifestyle intervention, in adults with a BMI ≥ 30 kg/m2, or ≥27 kg/m2 and at least one obesity-related disease. It was later approved in 2020 for the treatment of obesity in adolescents aged 12+ years(55). Early studies in 2009 revealed the mean weight reduction in people with overweight and obesity without T2D was significantly greater with liraglutide 3⋅0 mg (−7⋅2 kg) than its comparator orlistat (−4⋅1 kg) and placebo (−2⋅8 kg) in adjunct to lifestyle intervention at 20 weeks(Reference Astrup, Rossner and Van Gaal56). Additionally, the Phase III SCALE Obesity and Prediabetes trial revealed that liraglutide 3⋅0 mg is highly effective for delaying the progression from pre-diabetes to T2D; the time to onset of T2D was 2⋅7× longer in the liraglutide group compared to placebo at a 3-year follow-up(Reference le Roux, Astrup and Fujioka57). Liraglutide also has an additive beneficial effect on blood pressure(Reference Astrup, Rossner and Van Gaal56) and lipid profile(Reference Aoki, Kamiyama and Takihata58) in people with overweight or obesity. The most common dose-dependent side effects of liraglutide are mild and primarily gastrointestinal (e.g. nausea, diarrhoea and constipation) which are typically lessened with a titration period(Reference Cignarella, Busetto and Vettor59,Reference Drucker60) .
Unfortunately, the weight-reducing efficacy of liraglutide 3⋅0 mg fails to rival weight loss achieved from bariatric surgery and was further revealed it is not as efficacious in those with T2D, which constitutes a large subgroup of those with overweight and obesity. The SCALE Diabetes randomised trial revealed liraglutide 3⋅0 mg produced on average weight loss of 5⋅4 % of baseline weight at 56 weeks in those with obesity and T2D, compared to 8⋅0 % in those without diabetes, compared to those in the placebo group (−2⋅0 %)(Reference Davies, Bergenstal and Bode61,Reference Pi-Sunyer, Astrup and Fujioka62) . The inferior weight-loss efficacy in those with T2D is likely due to a poorer metabolic profile and other peripheral effects such as insulin resistance which may impair satiety(Reference Tiedemann, Schmid and Hettel63).
Other limitation that restricts clinical utility of liraglutide is that it is only suitable for a select number of patients due to its exclusion criteria. Tolerability is another limitation with GLP-RA, with up to 14⋅8 % of patients discontinuing liraglutide treatment within 3 months due to intolerable side effects, with many others remaining on lower therapeutic doses due to unendurable side effects experienced at 3⋅0 mg(Reference Balasanthiran, Munro and Watters64). Treatment should also be stopped if patients have not achieved at least 5 % weight loss after 12 weeks of treatment with 3⋅0 mg liraglutide daily(Reference Cignarella, Busetto and Vettor59).
Semaglutide
Semaglutide is the latest generation GLP-1RA for chronic weight management in adults with obesity or overweight with at least one weight-related comorbidity, as an adjunct to lifestyle intervention. Similar to liraglutide, semaglutide offers the unique advantage of glycaemic regulation by stimulating insulin secretion and decreases glucagon secretion which improves glycaemic control(Reference Eliaschewitz and Canani65). Semaglutide was initially approved for T2D therapy use of up to 1⋅0 mg via subcutaneous injection(66) or up to 14 mg once daily oral administration in 2017 and 2019, respectively, by the European Medicines Agency and FDA(67).
Semaglutide has a significant advantage over liraglutide and orlistat for patient utility and treatment adherence; it has been modified to have a half-life of 180 h allowing for reduced dosing to once-weekly subcutaneous injection(Reference Enebo, Berthelsen and Kankam23). Similar to other GLP-1RA, semaglutide has been shown in preclinical studies to modulate the activity of key neurones within the arcuate nucleus of the hypothalamus that regulate hunger and also reward regions of the brain(Reference Knudsen and Lau68).
Encouraging results from the comprehensive global Phase IIIa semaglutide treatment effect in people with obesity (STEP) trial programme revealed that in STEP 1, 1961 participants with obesity or overweight without T2D experienced an average weight reduction of 15⋅3 kg (−14⋅9 %) at 68 weeks from baseline (average 105⋅3 kg) with once-weekly semaglutide 2⋅4 mg with lifestyle intervention, compared to a 2⋅6 kg (−2⋅4 %) reduction in the placebo group(Reference Wilding, Batterham and Calanna69). Further, weight loss was similar to that following bariatric surgery in 32 % of participants who lost >20 % of their baseline body weight(Reference Wilding, Batterham and Calanna69). Similar to other GLP-1RA, transient and mild or moderate symptoms such as nausea, diarrhoea and injection site irritation were the most commonly reported adverse events.
The STEP 4 trial investigated the effect of semaglutide 2⋅4 mg for 68 weeks v. crossover to placebo at 20 weeks in participants with overweight or obesity (without T2D). This study highlighted the importance of long-term weight management therapies as those who discontinued semaglutide and switched to placebo experienced a mean weight regain of 6⋅9 %, whereas those who remained on semaglutide experienced a mean weight reduction of 7⋅9 %(Reference Rubino, Abrahamsson and Davies70).
The STEP 8 head-to-head trial revealed that semaglutide 2⋅4 mg has superior weight-reducing efficacy than its current comparator liraglutide 3⋅0 mg in participants with overweight or obesity without diabetes. Results showed clinically relevant mean weight loss of −15⋅8 % with semaglutide v. −6⋅4 % with liraglutide at 68 weeks and was also accompanied by greater improvements in several cardiometabolic parameters. The number of participants achieving ≥10, ≥15 and ≥20 % weight loss were also greater with semaglutide v. liraglutide (70⋅9 v. 25⋅6 %, 55⋅6 v. 12⋅0 % and 38⋅5 v. 6⋅0 %, respectively)(Reference Rubino, Greenway and Khalid54). These results highlight superior weight reduction from semaglutide when compared to other currently available anti-obesity medications. It remains to be established the underlying reason for this, although, it has been theorised that this may be due to enhanced central nervous system bioavailability with greater GLP-1R affinity and potency to achieve superior activation of key satiety centres within the brain(Reference Knudsen and Lau68). Semaglutide has also been reported to induce reductions in energy-dense food cravings and preferences and reward-related eating behaviour, which may lead to further reduced energetic intake and subsequent weight loss(Reference Blundell, Finlayson and Axelsen71). It may also be attributed to higher rates of treatment adherence due to its convenient once-weekly treatment regimen, which reduces the likelihood of missed doses and injection site irritation(Reference Wilding, Batterham and Calanna69). However, it is important to note that similar to liraglutide, participants with T2D experienced less weight loss with semaglutide 2⋅4 mg (−9⋅6 %) at 68 weeks compared to those without T2D in the STEP 2 trial. Nevertheless, 68⋅8, 45⋅6 and 25⋅8 % of participants with T2D achieved ≥5, ≥10 and ≥15 % weight loss, respectively(Reference Davies, Faerch and Jeppesen72). It was further revealed there was a significant sex-related difference in weight loss response of semaglutide observed with average weight reductions of 8⋅8 kg in women and 6⋅0 kg in men(Reference McDermid73), which is likely due to pharmacokinetic variability. This finding warrants further research to investigate adjusted dose modifications for men to enhance weight loss efficacy to reach comparability to women.
The results of the STEP programme led to the approval of semaglutide in 2021 at an elevated dose of 2⋅4 mg for management of people with overweight or obesity, regardless of T2D status, by the FDA and Medicines and Healthcare products Regulatory Agency(74).
As aforementioned, self-administered subcutaneous injections is a significant barrier for patients' treatment adherence due to injection site-related side-effects and reluctancy to self-inject. Promisingly, an oral administration of semaglutide has already been developed and approved for T2D therapy, which shows great potential for translation into an anti-obesity medication with a convenient mode of administration(67). In 2017, a phase II study using an oral formulation of semaglutide was tested in T2D patients with poorly controlled glycaemia to determine efficacy of various oral doses (2⋅5, 5, 10, 20 and 40 mg) when compared to subcutaneous administration of 1⋅0 mg. Promisingly, dose-dependent weight losses were observed after 26 weeks ranging from −2⋅1 to −6⋅9 kg with the highest dose comparable to semaglutide 1⋅0 mg (−6⋅4 kg) v. placebo (−1⋅2 kg)(Reference Davies, Pieber and Hartoft-Nielsen75). The Phase 3a PIONEER 4 trial in 2019 reported that 14 mg once-daily oral semaglutide resulted in significantly greater weight loss compared to the diabetes treatment dose of subcutaneous liraglutide (1⋅8 mg) (−4⋅4 v. 3⋅1 kg) in participants with T2D at 26 weeks(Reference Pratley, Amod and Hoff76). Further trials are warranted into the use of oral semaglutide in patients with obesity without T2D and to compare oral semaglutide with subcutaneous liraglutide and semaglutide at their approved weight management doses of 3⋅0 and 2⋅4 mg, respectively.
Cagrilintide
Another gut hormone-based monotherapy under investigation for weight management is cagrilintide (AM833). Cagrilintide is a long-acting amylin analogue that reduces food intake and bodyweight in a dose-dependent manner by activating both amylin and calcitonin receptors(Reference Enebo, Berthelsen and Kankam23). Native amylin, co-secreted with insulin, is a neuroendocrine peptide secreted by pancreatic β cells that acts as a satiety signal, slows gastric motility and suppresses post-prandial glucagon in response to nutrient intake, by acting predominantly through the brain stem and binding to the area postrema(Reference Braegger, Asarian and Dahl77–Reference Zakariassen, John and Lutz79). As endogenous amylin has a half-life of approximately 13 min(Reference Boyle, Lutz and Le Foll80), cagrilintide has been modified to increase its half-life to 180 h, allowing for once-weekly subcutaneous dose(Reference Enebo, Berthelsen and Kankam23).
Cagrilintide (AM833) has proven efficacy as a monotherapy for weight loss in phase 2 randomised, double-blind placebo and active controlled trial, which compared escalating doses of once-weekly cagrilintide with a placebo or once-daily liraglutide 3⋅0 mg, the current active comparator that is available for weight management in people with overweight and obesity. At 26 weeks, all doses of cagrilintide (0⋅30–4⋅5 mg) led to dose-dependent weight reduction of up to 10⋅8 % at the highest dose (4⋅5 mg) with greater efficacy than liraglutide 3⋅0 mg (−9⋅0 %) and placebo (−3⋅0 %) in people with overweight or obesity and at least one obesity-related comorbidity. On the highest dose of cagrilintide, 88⋅7 % of participants achieved ≥5 % weight loss, 53⋅5 % of participants achieved ≥10 % and 18⋅7 % achieved >15 % at week 26(Reference Lau, Erichsen and Francisco81).
Another study reported that cagrilintide-induced weight loss did not reach a plateau at 26 weeks(Reference Lau, Erichsen and Francisco81), which has been reported with liraglutide (weight loss plateau observed at week 40)(Reference Pi-Sunyer, Astrup and Fujioka62) and semaglutide (weight loss plateau observed at weeks 60–68)(Reference Rubino, Abrahamsson and Davies70). However, clinical trials with a longer duration are required to identify whether an eventual weight loss plateau occurs with cagrilintide usage. Cagrilintide may present an additive regulatory effect on food preferences and eating behaviour by influencing hedonic aspects of eating, such as reward and pleasure(Reference Boyle, Lutz and Le Foll80). This was reflected in participants self-reported improvement in cognitive restraint and emotional and uncontrolled eating behaviour which likely contributes to treatment efficacy(Reference Lau, Erichsen and Francisco81).
The fundamental difference between cagrilintide and GLP-1RA is that they operate on different regulatory pathways; cagrilintide acts predominantly in the area postrema and nucleus of the solitary tract of the hindbrain(Reference Boccia, Gamakharia and Coester82) whereas GLP-1RA acts predominantly through the hypothalamus(Reference Daniels and Mietlicki-Baase83). Utilising agents with varying mechanisms of action highlights the potential not only for combined therapies to provide a synergistic effect for enhanced weight loss efficacy but also to expand treatment options if other anti-obesity medications prove unsuitable or inadequate for patients.
Combination therapies
Given the complex nature of obesity and that multiple systems regulate body weight, it is unlikely that a monotherapy can emulate the efficacy of bariatric surgery, which targets and corrects various pathophysiological mechanisms. The lower efficacy of single-target therapies is likely due to activation of compensatory mechanisms when hormones are therapeutically altered(Reference Rodgers, Tschop and Wilding84). Therefore, the current rationale for anti-obesity medication is to model bariatric surgery by utilising a poly-therapeutic approach with complementary mechanisms of actions to maximise weight loss, minimise adverse effects and reduce redundancy mechanisms. This has led to the development of dual and tri-agonists of endogenous gut hormones (GLP-1, glucagon, amylin and glucose-dependent insulinotropic polypeptide (GIP)) to activate several different receptor signalling pathways.
Cagrilintide and semaglutide
Concomitant administration of semaglutide 2⋅4 mg with cagrilintide is currently under investigation. In the single-centre 20-week randomised phase 1b trial, various doses of cagrilintide (0⋅16, 0⋅3, 0⋅6, 1⋅2, 2⋅4 and 4⋅5 mg) were combined with semaglutide 2⋅4 mg in ninety-five participants with overweight or obesity (mean BMI 31(sd 3⋅2) kg/m2). This pharmacological combination produced clinically meaningful weight reductions as high as 17⋅1 % (with the highest dose of cagrilintide 2⋅4 mg) when compared to placebo combined with semaglutide 2⋅4 mg (−9⋅8 %), without lifestyle intervention(Reference Enebo, Berthelsen and Kankam23). These results highlight the potential of dual agonist therapies, compared to single-target therapies, to enhance weight loss.
With weight reductions of 25 % typically observed after bariatric surgery, these results provide assurance of narrowing the treatment gap between pharmacotherapy and bariatric surgery. Importantly, the combination of semaglutide 2⋅4 mg with cagrilintide was not associated with an increase in adverse events when compared to semaglutide monotherapy. However, larger and longer trials are needed to assess the efficacy and safety of this pharmacological combination before it can be approved.
A phase 2 trial (Clinical Trials.gov identifier: NCT04982575) is currently recruiting to investigate the effectiveness of combination cagrilintide and semaglutide for weight reduction and HbA1c levels in people with overweight or obesity and T2D. Development of anti-obesity medications that are suitable and effective in people with T2D is crucial considering 90 % of those with T2D have overweight or obesity(Reference Gatineau, Hancock and Holman85).
Tirzepatide
Tirzepatide is a novel dual GIP and GLP-1RA with a half-life of 5 d which allows administration as a once-weekly subcutaneous injection(Reference Min and Bain86). In preclinical models, GIP has been shown to decrease food intake, increase glucagon secretion, stimulate insulin secretion and increase energy expenditure therefore resulting in weight reductions(Reference NamKoong, Kim and Jang87), and when combined with a GLP-1RA, may result in greater effects on glucose and body weight(Reference Min and Bain86).
The promising therapeutic efficacy of tirzepatide was demonstrated in the head-to-head SURPASS-2 Phase 3 trial comparing once-weekly tirzepatide with semaglutide 1⋅0 mg (T2D therapy dose) in patients with uncontrolled T2D with a mean body weight of 93⋅7 kg. During 40 weeks of treatment, a dose-dependent effect was evidenced with tirzepatide, with all treatment doses showing superiority over semaglutide with greater reductions in HbA1c and mean weight reductions of 7⋅6 kg (−8⋅5 %), 9⋅3 kg (−11 %) and 11⋅2 kg (−13⋅1 %) with the 5, 10 and 15 mg doses, respectively, compared with a 5⋅7 kg weight reduction with semaglutide(Reference Frias, Davies and Rosenstock88). Importantly, the frequency and severity of adverse events in the tirzepatide group were comparable to those taking semaglutide. Future head-to-head studies are warranted to investigate the efficacy of tirzepatide v. the approved weight-management dose of semaglutide 2⋅4 mg and in those without T2D.
Multi-receptor agonists
Poly-agonist-based pharmacotherapies, which intervene at multiple targeted physiological pathways for comprehensive metabolic regulation are currently under early clinical research and development.
LY3437943
Systemic levels of GLP-1, GIP and glucagon are significantly elevated post-bariatric surgery(Reference Jimenez, Casamitjana and Viaplana-Masclans89,Reference Tschop, Finan and Clemmensen90) . LY3437943, a novel balanced GLP-1/GIP/glucagon tri-agonist that has shown encouraging efficacy in preclinical models of obesity and diabetes(Reference Finan, Yang and Ottaway91) with weight loss exceeding that observed with tirzepatide. The therapeutic aim of this approach is that the presence of both GLP-1 and GIP, which induce satiety, increases energy expenditure and reduces energy intake, will counteract the hyperglycaemic effects of glucagon agonism by elevating insulin secretion(Reference Capozzi, DiMarchi and Tschop92,Reference Muller, Bluher and Tschop93) . As a result, this may permit the use of greater therapeutic doses of GLP-1 and GIP, whilst minimising adverse events, to achieve greater weight loss.
Phase II placebo controlled studies are currently recruiting to investigate LY3437943 in healthy subjects (ClinicalTrials.gov: NCT03841630), those with obesity without T2D (ClinicalTrials.gov: NCT04881760) and in those with T2D (ClinicalTrials.gov: NCT04143802) to investigate optimal dose and its weight reducing and glycaemic effects with highly anticipated data.
These promising multi-agonist developments provide an insight into the potential of future anti-obesity medications treatment, which aim to mimic the physiological responses induced by bariatric surgery, to optimise weight loss and simultaneously target obesity-related diseases. Further consideration and incorporation of other gut hormones that play a role in improved metabolic control and weight loss post-surgery are required. Table 1 provides a summary of studies outlined earlier.
Table 1. Summary of studies on gut hormone based pharmacotherapy for obesity.
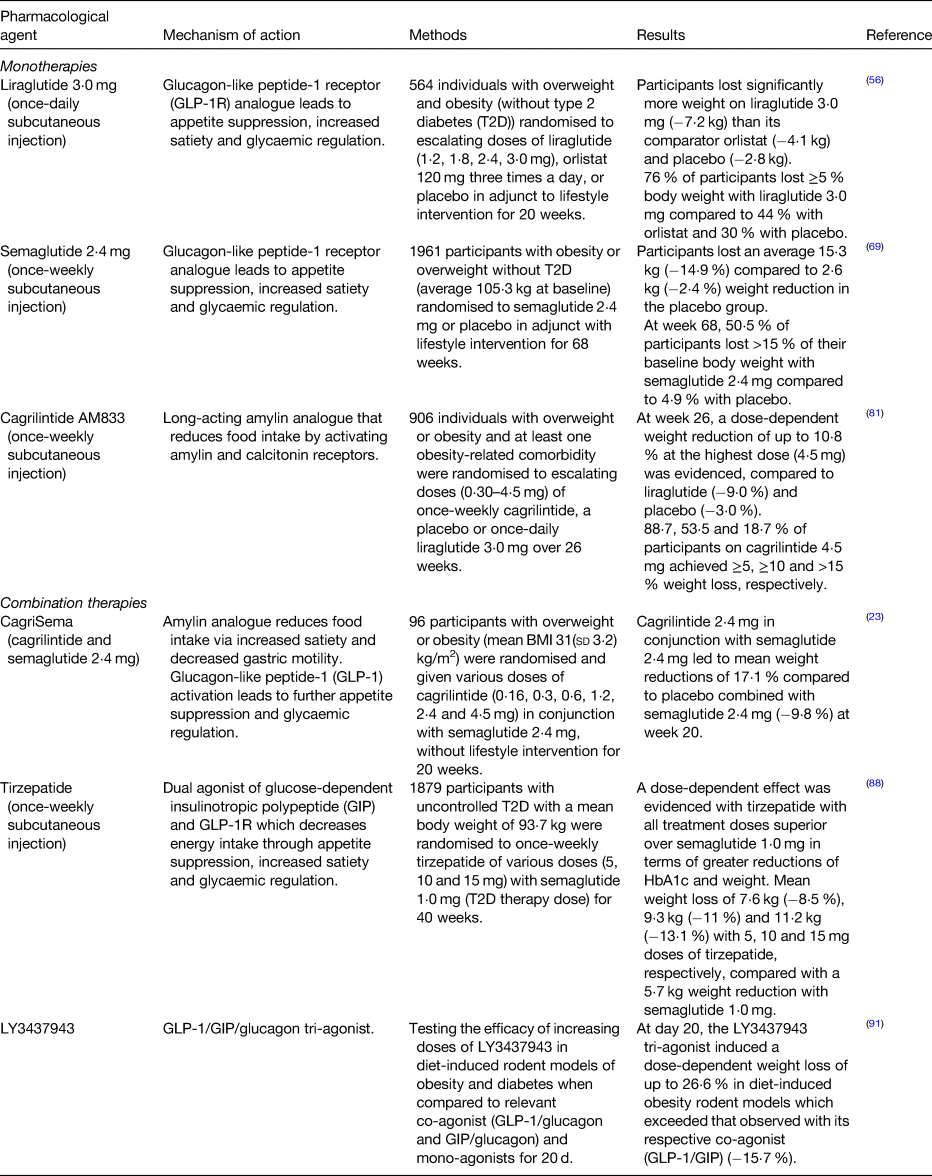
Conclusions
Scientific advancements have revealed the potential of therapeutic modification of gut peptides that modulate neural signalling involved in body weight regulation. The translation of the scientific understanding of body weight regulation has resulted in new anti-obesity medications that engender ≥10 % weight loss and are closing the treatment gap. Similar to lifestyle intervention and bariatric surgery, responses to pharmacotherapies for obesity are highly heterogenous. It is unlikely there will be a ‘one size fits all’ pharmacotherapy which is successful for all individuals living with obesity. Approval of a diverse range of therapeutics is crucial to address the obesity epidemic, narrow the treatment gap and to improve the health and quality of life in those with overweight and obesity. The future management of obesity is evolving towards gut hormone-based combination approaches to mimic post-surgery changes to optimise weight loss. Further research efforts should focus on personalised medicine approaches tailored to the individual.
Acknowledgements
We thank the reviewers for taking the time to review the manuscript and for their valuable comments and suggestions.
Financial Support
R. L. B. and C. F. receive funding from NIHR; R. L. B. also receives funding from Sir Jules Thorn Charitable Trust, Rosetrees Trust and Stoneygate Trust.
Conflict of Interest
Within the past 36 months and outside of the submitted work, R. L. B. reports research grants from National Institute for Health Research, Rosetrees Trust and Sir Jules Thorn Trust, and personal fees from Novo Nordisk, ViiV Healthcare Ltd, Pfizer, Gila Therapeutics Inc., Eli-Lilly and International Medical Press. R. L. B. also reports unpaid roles with Royal College of Physicians, the Association for the Study of Obesity, British Obesity and Metabolic Surgery Society, National Bariatric Surgery Registry, BSR, International Federation for the Surgery of Obesity and Metabolic Disorders, European Society of Endocrinology, Obesity Empowerment Network UK and National Institute for Health and Care Excellence. C.F declares no conflict of interest.
Authorship
Both authors contributed equally to the writing of the manuscript.