Anthocyanins are natural colourants derived from many flowers, fruits, vegetables and legumes. They are present exclusively as glycosidic compounds in plants (Harborne & Grayer, Reference Harborne, Grayer and Harborne1988). Depending on the pH, pigments of anthocyanins are red to purple or blue. Recently, anthocyanins have attracted interest in the food industry as safe and effective food colourants, and their consumption is on the increase as concentrated blueberry, elderberry and red grape extracts are commercially available. Several researchers have reported that anthocyanins have remarkable antioxidant and free radical scavenging activities (Wang et al. Reference Wang, Cao and Prior1997; Tsuda et al. Reference Tsuda, Horio and Osawa2000), and can inhibit LDL oxidation in vitro (Kähkönen & Heinonen, Reference Kähkönen and Heinonen2003), findings which suggest that anthocyanin contents of berries, grapes and the purple sweet potato may provide possible health benefits such as reduction of CHD (Zern et al. Reference Zern, Wood, Greene, West, Liu, Aggarwal, Shachter and Fernandez2005), anti-inflammatory activity (Afaq et al. Reference Afaq, Saleem, Krueger, Reed and Mukhtar2005) and anticancer activity (Hagiwara et al. Reference Hagiwara, Yoshino, Ichihara, Kawabe, Tamano, Aoki, Koda, Nakamura, Imaida, Ito and Shirai2002). An epidemiological study has also indicated that intakes of anthocyanin-rich fruits and vegetables are related to health benefits (Omenn, Reference Omenn1995).
Potato cultivars (Solanum tuberosum L.) are one of the most widely grown high carbohydrate crops in the world. In general, potatoes have not been regarded as being rich in antioxidants. However, depending on the genetic variety, they can contain considerable amounts of polyphenols, flavonoids, anthocyanins or ascorbic acid (vitamin C) (Augustin, Reference Augustin1975; Lewis et al. Reference Lewis, Walker, Lancaster and Sutton1998; Brown, Reference Brown2005). Recently, new potato varieties have been shown to be rich in antioxidants. Among these, coloured potatoes have attracted special interest in many countries (Johnson, Reference Johnson1995) due to their colour appeal and excellent taste (Sorenson, Reference Sorenson1992). Several researchers have studied the anthocyanin contents of coloured potato tubers. The pigments have been identified as anthocyanin derivatives (Lewis et al. Reference Lewis, Walker, Lancaster and Sutton1998; Rodriguez-Saona et al. Reference Rodriguez-Saona, Giusti and Wrolstad1998; Eichhorn & Winterhalter, 2005). In fact, the first anthocyanin in a coloured potato, malvidin-3-(p-coumaroryl-rutinoside)-5-glucose, was identified by Chmielewska (Reference Chmielewska1936) in the 1930s. Like anthocyanins from other plants, it has been suggested that analogous potato anthocyanins may also be important functional foods (Brown, Reference Brown2005). We have recently reported that purple potato extract prevents the liver injury induced by d-galactosamine in rats (Han et al. Reference Han, Hashimoto, Shimada, Sekikawa, Noda, Yanauchi, Hashimoto, Chiji, Topping and Fukushima2006). However, less well appreciated is the bioavailability of anthocyanins found in coloured potatoes, which may act as antioxidants in the human diet.
In the present study, we investigated the antioxidant effects of pigmented fractions prepared from Kitamurasaki (KM) and Hokkai no. 92 (H92) potato flakes on radical scavenging activity and linoleic acid oxidation in vitro. Furthermore, we examined the antioxidant effects of those potato flakes on serum antioxidant potential, hepatic lipid peroxidation and hepatic mRNA in rats.
Materials and methods
Chemicals
Trolox (6-hydroxy-2,5,7,8-tetramethyl-chroman-2-carboxylic acid), TCA, butylated hydroxytoluene, bovine serum albumin and thiobarbituric acid were purchased from Sigma Chemical Co. (St Louis, MO, USA). 2,2′-Azobis (2-amidinopropane) dihydrochloride and 1,1-diphenyl-2-picrylhydrazyl (DPPH) were obtained from Wako Pure Chemicals Industries (Osaka, Japan).
Preparation of potato flakes and pigmented fractions
Purple potato flakes of KM (light purple) and H92 (medium-dark purple) were a kind gift from Somatech Center, House Foods Corporation, Japan. The preparation of potato flakes was as follows: KM and H92 potatoes were thoroughly washed with water and air dried on filter paper, and then they were peeled and sliced. The sliced potatoes were treated with steam blanching to minimize enzymatic reactions that bring out degrading anthocyanins. Next, they were mashed and dried in a drum dryer, and finally ground to flakes.
For preparation of pigmented fractions, 50 g potato flakes were subjected to extraction by exposure to 5 % acetic acid three times overnight at room temperature. The suspension was centrifuged at 5500 g for 20 min, and extraction from the resultant precipitate was done under the same conditions. Again the supernatant was centrifuged at 28 400 g for 30 min. The upper layer was separated and directly applied on to Diaion HP-20 resin (Nippon Rensui Co., Tokyo, Japan). Sugars, proteins and salts were removed by washing the column with 1 % acetic acid. Anthocyanin was retained by the resin and finally eluted with a mixture of 70 % ethanol. Ethanol was removed using a rotary evaporator at 35°C, and the eluate was diluted with water and lyophilized to give anthocyanin-rich pigmented fractions.
Identification of anthocyanins from pigmented fraction
Anthocyanin pigments were analysed using HPLC, liquid chromatography–MS, and 1H and 13C NMR. Analytical HPLC was carried out using an LC-10AD pump system (Shimadzu, Kyoto, Japan) and a TSKgel ODS-80Ts column (4·6 mm × 250 mm; Tosoh, Tokyo, Japan) with a flow rate of 1·0 ml/min and monitoring at 360 nm (SPD-10A wavelength detector; Shimadzu). The solvent system was composed of water, methanol and trifluoroacetic acid (70:30:0·1, by vol.). The molecular weights of the major peaks were determined by liquid chromatography–MS (ZQ 2000; Waters, Milford, MA, USA) using the same HPLC conditions as above. The major peaks on the HPLC that were collected by preparative HPLC were used for 1H and 13C NMR analyses (ECA-500; JEOL, Tokyo, Japan) to determine the chemical structures.
Dietary fibre and micronutrient contents
Dietary fibre, protein, lipid, carbohydrate, moisture and ash in KM and H92 potato flakes were determined by the Association of Official Analytical Chemists (1990) procedure.
Total polyphenol contents
Total polyphenol concentrations in KM and H92 potato flakes were determined by the Folin-Ciocalteu method (Singleton et al. Reference Singleton, Orthofer and Lamuela-Raventós1998). The absorbance was measured at 750 nm using a Shimadzu 1600-UV spectrophotometer. Total phenolic contents of pigmented fractions from KM and H92 potato flakes were converted into mg gallic acid equivalents per 100 g powder weight.
Flavonoid contents
The absorbance of flavonoids was measured at 510 nm with standards based on the known (+)-catechin concentration (Jia et al. Reference Jia, Tang and Wu1999). Flavonoid contents of pigmented fractions from KM and H92 potato flakes were converted into mg catechin equivalents per 100 g powder weight.
Total anthocyanin contents
The amounts of monomeric anthocyanins were measured by a pH differential method (Giusti & Wrolstad, Reference Giusti, Wrolstad and Wrolstad2001). A Shimadzu 1600-UV spectrophotometer was used to measure the absorbance at 525 and 700 nm in buffer at pH 1·0 and 4·5. Anthocyanin contents were calculated using the molar extinction coefficient of cyanidin 3-glucoside (26 900 l/cm per mg) and absorbance A = [(A525 − A700)pH1·0 − (A525 − A700)pH4·5]. Anthocyanin contents of pigmented fractions from KM and H92 potato flakes were converted into mg per 100 g powder weight.
Radical scavenging activity and inhibition of linoleic acid oxidation
DPPH is a radical-generating substrate widely used to monitor the free radical scavenging abilities of various antioxidants (Brand-Williams et al. Reference Brand-Williams, Cuvelier and Berset1995). First, 0·3 ml of various concentrations of pigmented-fraction solutions (0–100 μg) from KM and H92 potato flakes was mixed with 0·9 ml of a solution containing 0·3 ml 0·4 mm-DPPH in ethanol, MES buffer (pH 4·5) and 20 % ethanol. Then the mixture was shaken vigorously and left to stand for 30 min. The optical density (OD) of the solution was then measured at 520 nm, and radical scavenging activity was obtained from the following equation: Radical scavenging activity (%) = (ODcontrol − ODsample)/ODcontrol × 100, where ODsample represents the absorption of the sample solution, and ODcontrol is that for the control solution (not containing the sample).
Linoleic acid oxidation was done by a modification of the method of Mitsuda et al. (Reference Mitsuda, Yasumoto and Iwami1996). In brief, 4 ml 0·03 % potato pigmented fraction solution from KM or H92 potato flakes was mixed with 40 ml 30 mm-linoleic acid substrate in 0·1 m-phosphate buffer (pH 7·0)–ethanol (4:1, v/v), and incubated at 40°C for 0, 1, 2, 4 and 5 d. Control groups were treated with 4 ml distilled water or 0·03 % trolox solution. Fractions (2 ml) from substrate solutions were obtained in every 24 h period and mixed with 1 ml 20 % TCA and 2 ml 0·67 % thiobarbituric acid in acetic acid. The mixtures were incubated in a boiling water-bath for 15 min. After cooling, 2 ml chloroform were added and the samples were centrifuged at 2000 g for 10 min. Absorbance of the supernatant was measured at 532 nm using a Shimadzu 1600-UV spectrophotometer.
Animals and diets
The experimental design was approved by the Animal Experiment Committee of Obihiro University of Agriculture and Veterinary Medicine. All animal procedures described conformed to National Institutes of Health guidelines (National Research Council, 1996). Male F344/DuCrj rats (8 weeks of age) were purchased from Charles River Japan (Yokohama, Japan). The animal facility was maintained on a 12 h light–dark cycle at a temperature of 23 ± 1°C and relative humidity of 60 ± 5 %. Animals were randomly assigned into three groups (n 5). There was no significant difference in body weight at the start of the experiment. Body weight and food consumption were recorded weekly and daily, respectively. The composition of each diet is shown in Table 1. Diets were based on the AIN-93G semi-purified rodent diet (Reeves et al. Reference Reeves, Nielsen and Fahey1993). Rats were fed KM or H92 potato flakes at 250 g/kg diet for 4 weeks. Control rats were fed a diet with cornstarch instead of potato flakes for 4 weeks. At the end of the experiment, rats were anaesthetized with Nembutal (sodium pentobarbital, 40 mg/kg body weight; Abbott Laboratories, Abbott Park, IL, USA) and killed. Blood samples were collected and taken into tubes without an anticoagulant. After the samples were allowed to stand at room temperature for 2 h, the sera were separated by centrifugation at 1500 g for 20 min. The livers were quickly removed, washed with cold saline (9 g NaCl/l), blotted dry on filter paper and weighed before freezing for storage at − 80°C.
Table 1 Experimental diets (g/kg diet)*
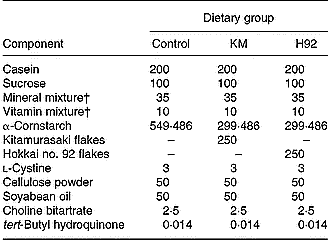
H92, Hokkai no. 92 potato flake diet; KM, Kitamurasaki potato flake diet.
* For details of diets, see p. 1127.
† Based on the AIN-93G diet composition (Reeves et al. Reference Reeves, Nielsen and Fahey1993).
Trolox equivalent antioxidant coefficient value of serum
The total antioxidant potentials of sera from rats fed the KM and H92 potato flake diets were determined using a commercial kit (NX 2332; Randox Laboratories, Antrim, UK), based on scavenging of 2,2′-azinobis(3-ethylbenzothiazoline-6-sulphonate) radical cations (Re et al. Reference Re, Pellegrini, Proteggente, Pannala, Yang and Rice-Evans1999).
Oxidation resistance of liver homogenate
Liver samples (0·5 g) were homogenized in 10 volumes of PBS (pH 7·4). The homogenates were treated with or without 2,2′-azobis (2-amidinopropane) dihydrochloride (final concentration 5 mm) at 37°C for 1 h in a shaking water-bath. Then the reaction was stopped by adding butylated hydroxytoluene (final concentration 60 μm). The degree of oxidation was immediately measured by the thiobarbituric acid reactive substances (TBARS) assay (Ohkawa et al. Reference Ohkawa, Ohishi and Yagi1979). Protein concentrations were determined by the Bradford assay (Bio-Rad, Hercules, CA, USA) (Bradford, Reference Bradford1976).
RNA isolation, RT–PCR and Southern blot analysis
Total RNA was isolated from the liver using ISOGEN reagent (Nippon Gene, Tokyo, Japan) according to the manufacturer's protocol (Chomczynski & Sacchi, Reference Chomczynski and Sacchi1987). The amounts of mRNA encoding Mn-superoxide dismutase (SOD), Cu/Zn-SOD, catalase, glutathione peroxidase (GSH-Px), glutathione reductase (GSH-R) and glycelaldehyde-3-phosphate dehydrogenase (GADPH, as an internal standard) were estimated by semi-quantitative RT–PCR and subsequent Southern blot analysis of PCR products with each inner oligonucleotide probe.
Total RNA samples were treated with DNase RQ1 (Promega, Madison, WI, USA) to remove genomic DNA, and were then subjected to RT–PCR using Moloney murine leukaemia virus RT (Gibco-BRL, Gaithersburg, MD, USA) and EX-Taq polymerase (Takara, Tokyo, Japan) with Mn-SOD primers of oligonucleotides (upstream primer, 5′-CGC TGT CAC TGT CAT CAT AAG-3′; downstream primer, 5′-GTC CGG TGC AGG GCG TCA TTC-3′), Cu/Zn-SOD primers of oligonucleotides (upstream primer, 5′-GTC CGG TGC AGG GCG TCA TTC-3′; downstream primer, 5′-CAA TCA CAC CAC AAG CCA AGC-3′), catalase primers of oligonucleotides (upstream primer, 5′-CTG GTT AAT GCG AAT GGA GAG-3′; downstream primer, 5′-TGG GGT AGT AGT AGT TGG GAG CAC-3′), GSH-Px primers of oligonucleotides (upstream primer, 5′-GCT GAA GGA GGC ACT TGG TCA-3′; downstream primer, 5′-TGA TGT ACT TGG GGT CGG TCA-3′), GSH-R primers of oligonucleotides (upstream primer, 5′-GCC GCA GCG TTA TTG TGG GTG-3′; downstream primer, 5′-CGA TAG GCG GGT GGC TGA AGA C-3′) and GAPDH primers of oligonucleotides (upstream primer, 5′-GCC ATC AAC GAC CCC TTC ATT-3′; downstream primer, 5′-CGC CTG CTT CAC CAC CTT CTT-3′). The reaction mixtures for the PCR contained 25 pmol each primer, 1·25 U EX-Taq polymerase, 1 × PCR buffer (Takara) and 200 μmol/l dNTP in a 50 μl reaction volume.
Amplification products were electrophoresed on 2 % agarose gel, and transferred to a nylon membrane (Biodyne B; Pall Bio-Support, East Hills, NY, USA). Blots were hybridized with an Mn-SOD probe of a fifty-three-base oligonucleotide (5′-CAG TCA GGA GCC TAG CTT GGG TCT GTT GAT TTG TTC AGT AGT GAG GTA GAC CC-3′), Cu/Zn-SOD probe of a fifty-four-base oligonucleotide (5′-AGC AGC CAC ATT GCC CAG GTC TCC AAC ATG CCT CTC TTC ATC CGC TGG ACC GCC-3′), catalase probe of a fifty-four-base oligonucleotide (5′-AGT TGG CCA CGC GAG CAC GGT AGG GAC AGT TCA CAG GTA TCT GCA GAT AGT TTG-3′), GSH-Px probe of a fifty-four-base oligonucleotide (5′-CCT TCT CAC CAT TCA CCT GGC ACT TCT CAA ACA ATG TAA AGT TGG GCT CGA ACC-3′), GSH-R probe of a fifty-four-base oligonucleotide (5′-ACC ACA TGG AGT TCC AAG CCC GAT GAG GTC TTC TTT ACT TCC TTA ACC TGT GAG-3′) and GAPDH probe of a fifty-four-base oligonucleotide (5′-TGA TGA CCA GCT TCC CAT TCT CAG CCT TGA CTG TGC CGT TGA ACT TGC CGT GGG-3′). The probes were 3′-tailing labelled with digoxigenin, using a digoxigenin oligonucleotide tailing kit (Boehringer, Mannheim, Germany). Prehybridization, hybridization and detection were carried out with a digoxigenin luminescent detection kit that contained an alkaline phosphatase-conjugated anti-digoxigenin antibody (Boehringer) as recommended by the manufacturer. The relative quantity of mRNA was estimated by densitometry scanning with X-ray film.
Statistical analysis
Data are presented as means and standard deviations. The significance of differences between two groups was determined by Student's t-test. The means and standard deviations for linoleic acid oxidation at prescribed times were calculated. The significance of differences among treatment groups was determined by ANOVA with Duncan's multiple range test (SAS Institute, Cary, NC, USA).
Results
The yields of pigmented fractions from KM and H92 potato flakes based on the flake weight were 1·24 % and 1·93 %, respectively. The major anthocyanin (over 90 %) in pigmented fractions from KM and H92 potato flakes was identified as petunidin-3-O-[6-O-(4-O-E-p-coumaroyl-O-α-rhamnopyranosyl)-β-glucopyranoside]-5-O-β-glucopyranoside (Fig. 1) by comparison with previous reports (Fossen et al. Reference Fossen, Øvstedal, Slimestad and Andersen2003) on 13C and 1H NMR analyses and MS spectroscopy.

Fig. 1 HPLC chromatogram (A) of purified anthocyanin from pigmented fractions of Kitamurasaski and Hokkai no. 92 potato flakes, and the structure (B) of the main anthocyanin from both Kitamurasaski and Hokkai no. 92 potato flakes. The column was conditioned with water–methanol–trifluroacetic acid (70:30:0·1, by vol.) for 30 min at 40°C with a flow rate of 1 ml/min monitoring at 360 nm. *The major peak was identified as petunidin-3-O-[6-O-(4-O-E-p-coumaroyl-O-α-rhamnopyranosyl)-β-glucopyranoside]-5-O-β-glucopyranoside.
The contents of total phenols, flavonoids and anthocyanins in pigmented fractions from KM and H92 potato flakes are shown in Table 2. The contents of total phenols in pigmented fractions from KM and H92 potato flakes were 241·7 and 505·4 mg/100 g powder, respectively. The contents of flavonoids of pigmented fractions from KM and H92 potato flakes were 65·7 and 131·4 mg/100 g powder, respectively. Moreover, the contents of total monomeric anthocyanins in pigmented fractions from KM and H92 potato flakes were 55·5 and 213·8 mg/100 g powder, respectively. All contents of total phenols, flavonoids and anthocyanins in the pigmented fraction from H92 potato flakes were higher than those in the pigmented fraction from KM potato flakes.
Table 2 Micronutrient and antioxidant contents (g/100 g powder) in purple potato flakes†

H92, Hokkai no. 92; KM, Kitamurasaki.
† For details of procedures, see pp. 1126–1127.
DPPH radical scavenging activities of pigmented fractions from KM and H92 potato flakes were expressed as the OD of 0·4 mm-DPPH solution (Fig. 2(A)) and as the effective dose (ec50) for scavenging 50 % of the DPPH radicals (Fig. 2(B)). A lower ec50 shows higher radical scavenging activity. The amount of trolox required for ec50 was 28·9 μg/ml. H92 had a lower ec50 for DPPH radical scavenging activity (110·1 μg/ml) than KM (ec50 = 164·6 μg/ml). The pigmented fractions from KM and H92 potato flakes most successfully suppressed the linoleic acid oxidation (Fig. 3). The inhibition of linoleic acid oxidation by the equivalent of 300 μg/ml samples was higher in the order trolox>H92 ≥ KM>control.

Fig. 2 1,1-Diphenyl-2-picrylhydrazyl (DPPH) radical scavenging activities of purple-pigment fractions from Kitamurasaki (KM) and Hokkai no. 92 (H92) potato flakes expressed as the OD of 0·4 mm-DPPH solution (A) (♦, KM; □, H92) and as the effective dose (ec50) for scavenging 50 % of the DPPH radicals (B). For details of procedures, see pp. 1126–1127. Trolox, 6-hydroxy-2,5,7,8-tetramethyl-chroman-2-carboxylic acid.

Fig. 3 Antioxidant effects of purple-pigment fractions from Kitamurasaki (KM) and Hokkai no. 92 (H92) potato flakes on linoleic acid oxidation. Mixtures were incubated at 40°C for 0, 1, 2, 4 and 5 d. Each of the fractions from substrate solutions (n 3) was obtained in every 24 h period. For details of procedures, see pp. 1126–1127. Values are means with their standard deviations depicted by vertical bars. ●, control; ○, 6-hydroxy-2,5,7,8-tetramethyl-chroman-2-carboxylic acid (trolox); ♦, 0·03 % KM; □, 0·03 % H92. a,b,c Mean values with unlike superscript letters were significantly different (P < 0·05).
Food intakes, body weight gains and liver weights of rats in KM and H92 groups were not significantly different from those in the control group (Table 3). However, body weight gain in the H92 group was significantly (P < 0·05) lower than in the KM group.
Table 3 Body weight, food intake and liver weight in rats fed Kitamurasaki and (KM) Hokkai no. 92 (H92) potato flakes for 4 weeks† (Mean values and standard deviations for five rats per group)
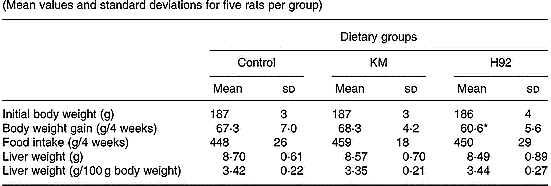
Mean value was significantly different from that of the KM group: *P < 0·05.
† For details of diets and procedures, see pp. 1126–1127.
The total antioxidant capacities measured as Randox trolox equivalent antioxidant capacity in sera of rats fed the control, KM and H92 potato flake diets over a period of 4 weeks are shown in Fig. 4. Serum antioxidant potential in the KM group was similar to that in the control group, whereas the serum antioxidant potential in the H92 group was significantly (P < 0·05) higher than in the control group.
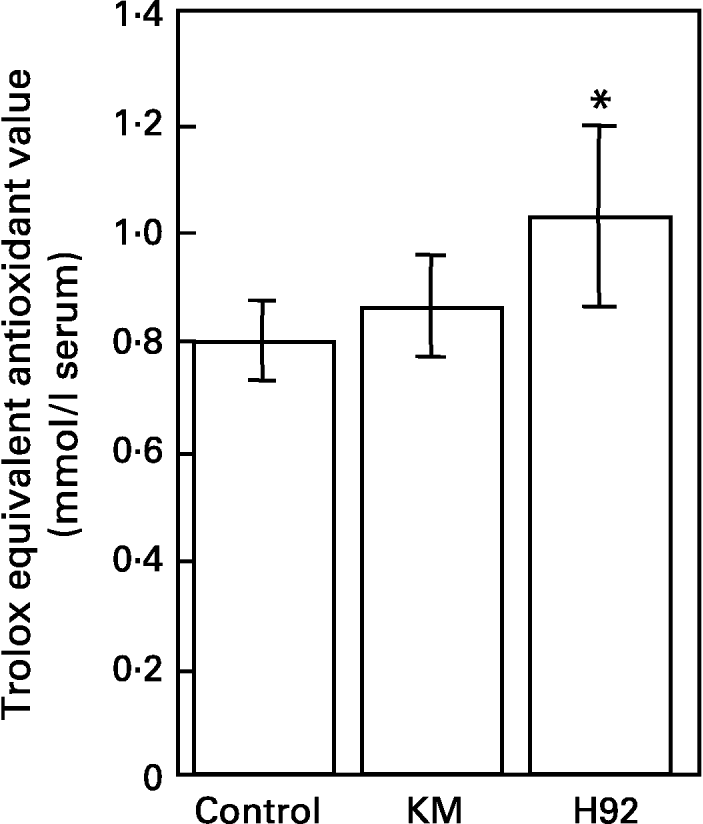
Fig. 4 Total antioxidant potentials of sera from rats fed the basal (control), Kitamurasaki (KM) and Hokkai no. 92 (H92) potato flake diets for 4 weeks determined by scavenging of 2,2′-azinobis(3-ethylbenzothiazoline-6-sulphonate) radical cations. For details of procedures and diets, see pp. 1126–1127 and Table 1. Values are means with their standard deviations depicted by vertical bars (data obtained from five animals). Mean value was significantly different from that of the control group (Student's t test): *P < 0·05. Trolox, 6-hydroxy-2,5,7,8-tetramethyl-chroman-2-carboxylic acid.
The TBARS levels from hepatic homogenates of control, KM and H92 groups are shown in Fig. 5. The hepatic TBARS level in the KM group was slightly lower than that in the control group; however, there was no significant difference, whereas the hepatic TBARS level in the H92 group was significantly (P < 0·01) lower than that in control group (Fig. 5(A)). Susceptibility to lipid peroxidation in hepatic homogenates from the control and H92 groups was induced by 2,2′-azobis (2-amidinopropane) dihydrochloride at 37°C for 1 h. The hepatic TBARS level in the H92 group was slightly but significantly (P < 0·05) lower than that of the control group (Fig. 5(B)).

Fig. 5 Thiobarbituric acid reactive substances (TBARS) levels from hepatic homogenate (A) without and (B) treated with 2,2′-azobis (2-amidinopropane) dihydrochloride (AAPH) in rats fed the basal (control), Kitamurasaki (KM) and Hokkai no. 92 (H92) potato flake diets for 4 weeks. Reaction was initiated by the addition of 5 mm-AAPH at 37°C for 1 h. For details of procedures and diets, see p. pp. 1126–1127 and Table 1. Values are means with their standard deviations depicted by vertical bars (data obtained from five animals). Mean values were significantly different from those of the control group (Student's t test): *P < 0·05; **P < 0·01.
The relative quantities of mRNA were determined by Southern hybridization of PCR-amplified Mn-SOD, Cu/Zn-SOD, catalase, GSH-Px and GSH-R cDNA in the rat liver. The values of Mn-SOD, Cu/Zn-SOD, catalase, GSH-Px and GSH-R mRNA were expressed relative to the value of GAPDH mRNA in all groups (Fig. 6). The relative hepatic Mn-SOD, Cu/Zn-SOD and GSH-Px mRNA levels in the H92 group were significantly (P < 0·01, P < 0·05 and P < 0·05, respectively) higher than those in the control group. Significant (P < 0·05) differences in Mn-SOD and Cu/Zn-SOD mRNA levels between the KM and control groups were also found. However, there were no significant differences in hepatic catalase and GSH-R mRNA levels among the groups.
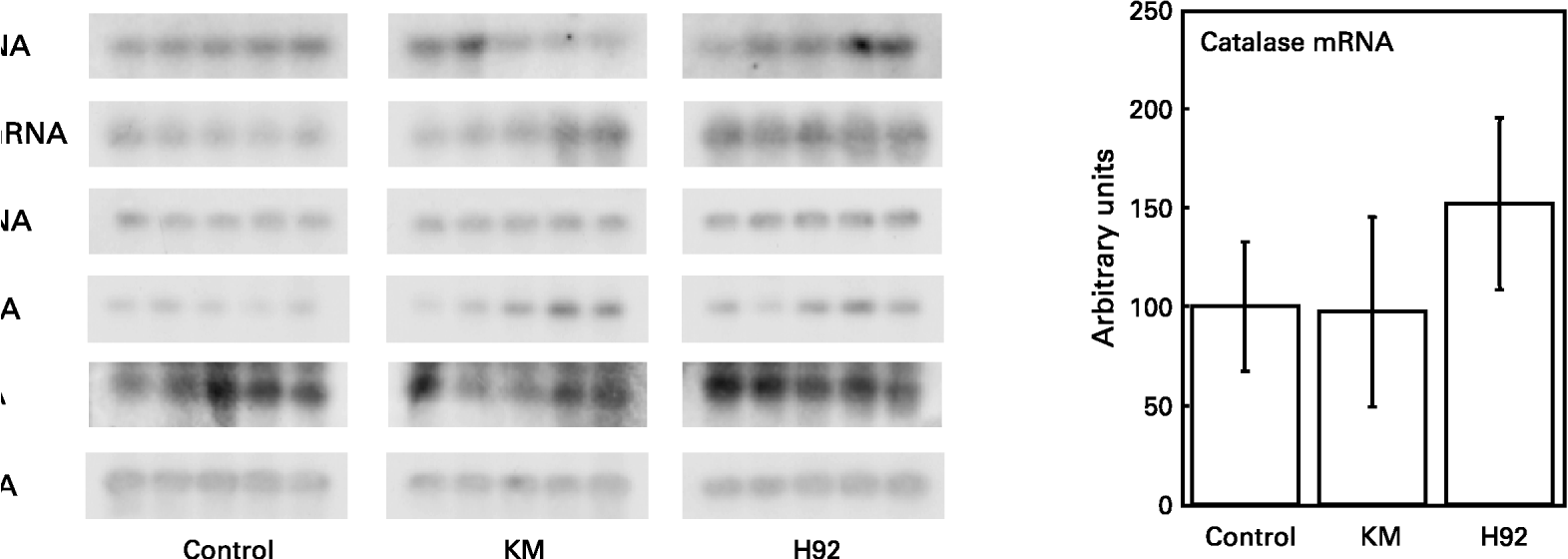
Fig. 6 Hepatic Mn-superoxide dismutase (SOD), Cu/Zn-SOD, catalase, glutathione peroxidase (GSH-Px) and glutathione reductase (GSH-R) mRNA expression in rats fed the basal (control), Kitamurasaki (KM) and Hokkai no. 92 (H92) potato flake diets for 4 weeks. For details of procedures and diets, see pp. 1126–1127 and Table 1. Values are means with their standard deviations depicted by vertical bars (data obtained from five animals). Mean values were significantly different from those of the control group (Student's t test): *P < 0·05; **P < 0·01. The values for Mn-SOD, Cu/Zn-SOD, catalase, GSH-Px and GSH-R mRNA are expressed relative to glyceraldehyde-3-phosphate dehydrogenase (GAPDH) mRNA in all groups. Representative samples illustrate mRNA levels measured by RT–PCR and Southern blotting.
Discussion
The first distinct study of anthocyanin in potatoes was reported in 1936, and the first identified anthocyanin in the potato was malvidin-3-(p-coumaroryl-rutinoside)-5-glucose (Chmielewska, Reference Chmielewska1936). Thereafter, potato tubers were found to have various anthocyanins, depending on the genetic variety, such as malvidin, peonidin, pelagonidin and petunidin (Brown, Reference Brown2005; Eichhorn & Winterhalter, Reference Eichhorn and Winterhalter2005). Recently, Lewis et al. (Reference Lewis, Walker, Lancaster and Sutton1998) reported that light to medium purple potato tubers contained petunidin together with smaller concentrations of malvidin, while dark purple-black tubers contained petunidin together with much higher concentrations of malvidin. In the present study, the pigmented fractions from both KM and H92 potato flakes mostly contained petunidin, which were similar findings to the results of Fossen et al. (Reference Fossen, Øvstedal, Slimestad and Andersen2003) and Lewis et al. (Reference Lewis, Walker, Lancaster and Sutton1998).
Some studies have indicated that phenolic compounds in coloured potatoes have antioxidant activities in vitro (Brown, Reference Brown2005; Reyes et al. Reference Reyes, Miller and Cisneros-Zevallos2005). In this study, the pigmented fractions from both KM and H92 potato flakes had antioxidant activities. The antioxidant capacity of the pigmented fraction from H92 potato flakes was higher than that of the pigmented fraction from KM potato flakes with regard to radical scavenging activity (H92, ec50 = 110·1 μg/ml and scope = − 0·0062; KM, ec50 = 164·6 μg/ml and scope = − 0·0057). This difference might have been due to the higher concentrations of phenolic constituents in the pigmented fraction from H92 potato flakes as the concentration of monomeric anthocyanins in the extract equivalent to the pigmented fraction from H92 potato flakes (111 μg/mg) was higher than that in the pigmented fraction from KM potato flakes (45 μg/mg). Furthermore, the concentration of total polyphenols in the extract equivalent to the pigmented fraction from H92 potato flakes (262 μg/mg) was higher than that in the pigmented fraction from KM potato flakes (195 μg/mg).
We also investigated the antioxidant effects of purple potato flakes in vivo, and found that H92 potato flakes enhanced the serum trolox equivalent antioxidant capacity values and suppressed hepatic TBARS levels in rats. Several researchers have reported that consumption of anthocyanins leads to increases in serum antioxidant potential in experimental animals (Auger et al. Reference Auger, Laurent, Laurent, Besancon, Caporiccio, Teissedre and Rouanet2004) as well as in human subjects (Cao et al. Reference Cao, Russell, Lischner and Prior1998; Pedersen et al. Reference Pedersen, Kyle, Jenkinson, Gardner, McPhail and Duthie2000; Mazza et al. Reference Mazza, Kay, Cottrell and Holub2002). Furthermore, Ramirez-Tortosa et al. (Reference Ramirez-Tortosa, Andersen, Gardner, Morrice, Wood, Duthie, Collins and Duthie2001) reported that an anthocyanin-rich extract decreased hepatic lipid peroxidation in vitamin E-depleted rats. The present findings suggest that the anthocyanin-rich H92 potato flake may also contribute to analogous effects on the antioxidant potential in vivo. It is reported that the increase in serum antioxidant potential caused by anthocyanins is due to the stability of intestinal absorption of anthocyanins in acidic media like gastric juice (Passamonti et al. Reference Passamonti, Vrhovsek, Vanzo and Mattivi2003). It has also been suggested that the effect on hepatic lipid peroxidation might be due to anthocyanin derivatives present in the liver (Miyazawa et al. Reference Miyazawa, Nakagawa, Kudo, Kayo and Someya1999) that scavenge free radicals via the formation of a DNA co-pigmentation complex (Sarma & Sharma, Reference Sarma and Sharma1999). In the present study, however, it is unlikely that the effect on serum and liver homogenate of the rats fed purple potato flakes was directly attributable to dietary anthocyanins. This is because not only are anthocyanins poorly absorbed by the intestine (Prior, Reference Prior2003) but also because the antioxidant properties of dietary polyphenols, including anthocyanins, in serum are impaired by hydroperoxide (Tsuda et al. Reference Tsuda, Horio and Osawa1998; Halliwell, Reference Halliwell2003). In addition, the serum level of flavonoids is usually in the range of 1 μm and in the case of anthocyanins may be much lower (Prior, Reference Prior2003). However H92 potato flakes increased the serum trolox equivalent antioxidant capacity values to approximately 200 μm (enhanced from 0·8 to 1·0 mm). Therefore, the increased serum trolox equivalent antioxidant capacity value in the H92 group may not only be related to increases in dietary polyphenols due to their poor bioavailability. However, it is well known that the serum antioxidant capacity is mostly due to certain antioxidants such as ascorbate, α-tocopherol, bilirubin and urate (Benzie & Strain, Reference Benzie and Strain1996; Cao et al. Reference Cao, Russell, Lischner and Prior1998). Augustin (Reference Augustin1975) reported a large range of vitamin C content among potato cultivars, varying from 84 to 145 mg/100 g on a dry basis, and Cao et al. (Reference Cao, Russell, Lischner and Prior1998) suggested that the increase in serum antioxidant capacity by consumption of strawberries and vitamin C was related to increases in the serum ascorbate and urate levels in man. Therefore, the increased antioxidant capacity in serum following the intake of purple potato flakes may be attributable to absorbed vitamin C or enhanced production of uric acid from purine metabolism. However, we do not have data for the serum urate and ascorbate levels.
Owing to limited absorption and conversion to other metabolites (Stahl et al. Reference Stahl, van den Berg and Arthur2002; Prior, Reference Prior2003), the antioxidant properties of dietary polyphenols from purple potato flakes may be exerted mainly in the gastrointestinal tract. The consumption of a meal rich in lipids and/or carbohydrates increases the susceptibility of the organism to oxidative damage (Bae et al. Reference Bae, Bassenge, Kim, Kin, Kim, Lee, Moon, Lee, Park and Schwemmer2001; Sies et al. Reference Sies, Stahl and Sevanian2005). However, the postprandial oxidative stress is restricted when meals containing excess lipids and/or carbohydrates are consumed together with foods rich in polyphenols (Natella et al. Reference Natella, Belelli, Gentili, Ursini and Scaccini2002). This inhibitory action is explained by the fact that during gastrointestinal passage, polyphenols decompose lipid hydroperoxide (Stahl et al. Reference Stahl, van den Berg and Arthur2002; Sies et al. Reference Sies, Stahl and Sevanian2005) and chelate autoxidation-promoting metal ions (Yoshino & Murakami, Reference Yoshino and Murakami1998). In the present study, scavenging of such adverse food constituents in vivo might have resulted in up-regulation of the expression of antioxidant enzymes (Moskaug et al. Reference Moskaug, Carlsen, Myhrstad and Blomhoff2004; Yeh & Yen, Reference Yeh and Yen2006) and reduction of oxidative damage in the liver (Ramirez-Tortosa et al. Reference Ramirez-Tortosa, Andersen, Gardner, Morrice, Wood, Duthie, Collins and Duthie2001). Another possible inhibitory action might be reduced primary or secondary carbohydrate autoxidation products such as glycated proteins in the gastrointestinal tract. Thus, it might also be that the postprandial oxidative damage in the intestinal epithelial cells induced by potato carbohydrate autoxidation products was lower in the present study because the carbohydrate concentrations in purple potato groups (H92, 705 g/kg diet; KM, 712 g/kg diet) were lower than in the control group (750 g/kg diet). Recently, Yeh & Yen (Reference Yeh and Yen2006) reported that hepatic SOD and GSH-Px mRNA expression in rats with phenolic compound supplementation was increased. In the present study, the hepatic Mn-SOD, Cu/Zn-SOD and GSH-Px mRNA expression in the KM or H92 groups was higher than in the control group. Therefore, it is assumed that factors up-regulating the expression of SOD and GSH-Px mRNA of rats following the intake of purple potato flakes may lead to inhibition of hepatic lipid peroxidation, which may be involved in the postprandial oxidative stress induced by potato carbohydrate autoxidation products or putative counteraction by polyphenols present in the gastrointestinal tract (Sies et al. Reference Sies, Stahl and Sevanian2005).
In conclusion, the present study suggests that pigmented fractions from purple potato flakes have antioxidant functions with regard to radical scavenging activity and inhibit linoleic acid oxidation in vitro. In addition, H92 potato flakes may indirectly reduce hepatic lipid peroxidation in rats by modulating the expression of hepatic Mn-SOD, Cu/Zn-SOD and GSH-Px mRNA. However, further study is necessary to clarify the antioxidant capacities of purple potatoes in vivo.
Acknowledgements
We are grateful to Dr Hiroaki Yamauchi (The National Agricultural Research Center for the Hokkaido Region) who kindly offered the potato tubers for this study. This work was supported in part by a grant from the Research and Development Program for New Bio-industry Initiatives of the Bio-oriented Technology Research Advancement Institution, by a Grant-in-Aid for JSPS Fellows, by a grant from Cooperation of Innovative Technology and Advanced Research in Evolutional Area (CITY AREA), and by a grant from the 21st Century COE Program (A-1), Ministry of Education, Culture, Sports, Science and Technology of Japan.