Twin pregnancy is a common phenomenon. According to Hellin’s law discovered in 1895, it used to occur, on average, once per 80 births (Gall, Reference Gall1996). Later studies revealed strong variation in frequency of twin births among different populations. The lowest twinning rate of less than 0.9% is found in populations of South America and East, Southeast and South Asia, whereas the highest values of more than 1.8% are characteristic of many African populations (Smits & Monden, Reference Smits and Monden2011). The frequency of twin pregnancies in developed countries of Europe, Oceania and North America used to fall in between, but it has grown dramatically over the last four decades, reaching values observed in sub-Saharan Africa (Pison & D’Addato, Reference Pison and D’Addato2006; Pison et al., Reference Pison, Monden and Smits2015). The increase of occurrence of twin pregnancies is assumed to result from a shift in age at first reproduction (the share of twin pregnancies grows with the age of a mother) and from more and more common use of assisted reproduction technology (Blickstein et al., Reference Blickstein, Verhoeven and Keith1999; Doyle, Reference Doyle1996; Wimalasundera & Fisk, Reference Wimalasundera and Fisk2002).
Twin pregnancies are classified as dizygotic (DZ; resulting from fertilization of two ova by two sperms) and monozygotic (MZ; resulting from division of an embryo to two identical embryonic structures within the first 14–16 days from fertilization of one ovum by one sperm; Benson & Doubilet, Reference Benson and Doubilet1991; Golan et al., Reference Golan, Amit, Baram and David1981; Machin, Reference Machin1993). In European populations, MZ and DZ pregnancies constitute 30% and 70% of twin pregnancies, respectively (Blickstein et al., Reference Blickstein, Verhoeven and Keith1999; Machin, Reference Machin1993). While pharmacological ovarian stimulation and multiple embryo transfer are related to higher frequency of polyzygotic pregnancies, in vitro technology is suspected to provoke later divisions of embryos due to manipulation of the zona pellucida (Blickstein et al., Reference Blickstein, Verhoeven and Keith1999; Derom et al., Reference Derom, Derom, Vlietinck, Vanden Berghe and Thiery1987; Edwards et al., Reference Edwards, Mettler and Walters1986).
Multiple pregnancies, including twin pregnancies, are regarded as pathological in humans as far as they are related to 4–10 times higher rates of complications (including fatal outcomes) for a pregnant woman and for fetuses (Baldwin, Reference Baldwin1994; Campbell, Reference Campbell2000). Thus, they require special medical care during pregnancy. It concerns especially MZ twin pregnancies, which are characterized by at least 2.5 times higher mortality of fetuses (Landy & Nies, Reference Landy, Nies, Keith, Papiernik, Keith and Luke1995; Monteagudo et al., Reference Monteagudo, Timor-Tritsch and Sharma1994), mainly due to twin-to-twin transfusion syndrome (TTTS) in monochorionic diamniotic pregnancies, which constitute about two-thirds of MZ twin pregnancies (Simpson & Miller, Reference Simpson, Miller, Copel, D’Alton, Feltovich, Gratacós, Krakow, Odibo, Platt and Tutschek2018).
In recent years, testing of cell-free DNA (cfDNA) circulating in the plasma of pregnant women has become another, besides ultrasound examination, very useful, noninvasive method of prenatal screening, which is safe for both the mother and the fetus (Allyse et al., Reference Allyse, Sayres, King, Norton and Cho2012; Lo, Reference Lo2008; Lo, Hjelm et al., Reference Lo, Hjelm, Fidler, Sargent, Murphy, Chamberlain and Wainscoat1998; Mujezinovic & Alfirevic, Reference Mujezinovic and Alfirevic2007; Wright & Burton, Reference Wright and Burton2008). Apoptosis of placental cells leads to the presence of placental DNA in the mother’s bloodstream (Faas et al., Reference Faas, de Ligt, Janssen, Eggink, Wijnberger, van Vugt and Geurts van Kessel2012; Flori et al., Reference Flori, Doray, Gautier, Kohler, Ernault, Flori and Costa2004; Hochstenbach et al., Reference Hochstenbach, Nikkels, Elferink, Oudijk, van Oppen, van Zon and Page-Christiaens2015), which is known as feto-maternal microchimerism (Evans et al., Reference Evans, Lambert, Maloney, Furst, Moore and Nelson1999; Lo, Tien et al., Reference Lo, Tein, Lau, Haines, Leung, Poon and Hjelm1998; Tjoa et al., Reference Tjoa, Cindrova-Davies, Spasic-Boskovic, Bianchi and Burton2006). The content of placental DNA, commonly referred to in plasma cfDNA studies as fetal DNA, increases with gestational age, and according to various authors, it may constitute on average 3–10% of cfDNA circulating in plasma in early pregnancy and 6–20% of cfDNA in late pregnancy (Lo, Tien et al., Reference Lo, Tein, Lau, Haines, Leung, Poon and Hjelm1998; Lun et al., Reference Lun, Tsui, Chan, Leung, Lau, Charoenkwan and Lo2008; Wang et al., Reference Wang, Batey, Struble, Musci, Song and Oliphant2013).
Microsatellites, commonly known as short tandem repeats (STRs), constitute genome fragments with a variable number of tandem repeats of short repetitive units of 1–6 bp (Butler, Reference Butler2012). Due to a high level of polymorphism, these markers have found wide applications in forensic medicine for genetic human identification (Gill et al., Reference Gill, Kimpton, d’Aloja, Andersen, Bar, Brinkmann and Stenersen1994; Jobling & Gill, Reference Jobling and Gill2004; Moretti et al., Reference Moretti, Baumstark, Defenbaugh, Keys, Smerick and Budowle2001). Capillary electrophoresis is a technique used routinely in forensic genetics for separation and detection of STRs and enables differentiation of alleles differing in size by only one nucleotide. A significant number of observed alleles makes STR markers a perfect tool for analysis and differentiation of DNA mixtures coming from at least two individuals, which suggests their potential usefulness in detecting fetal alleles in the case of feto-maternal microchimerism.
Deletion/insertion polymorphism–STR (DIP–STR) markers constitute haplotypes comprising two polymorphic regions: deletion or insertion (DIP) and a microsatellite. In the case of DIP–STR markers, one of the primers recognizes a sequence complementary to deletion or insertion so that only an allele with deletion (S) or insertion (L) is amplified. In comparison to standard analysis of STR polymorphism, analysis of DIP–STR markers enables detection of an allele of a subject whose DNA constitutes a minority component in the mixture of DNA from two subjects, even if the content of this subject’s DNA is very low (Castella et al., Reference Castella, Gervaix and Hall2013; Cereda et al., Reference Cereda, Biedermann, Hall and Taroni2014; Oldoni et al., Reference Oldoni, Castella and Hall2015). One or two DIP–STR alleles of the minority component of highly unbalanced DNA mixtures can be identified when the major DNA contributor is DIP homozygous (SS or LL) and the minor DNA contributor is DIP heterozygous (SL), or when the major and minor DNA contributors are opposite DIP homozygous (SS/LL or LL/SS). When the minor DNA contributor has no unique S or L allele, DIP–STR typing of DNA mixtures resembles standard STR typing. Owing to the ability to detect alleles of the minority component in highly unbalanced DNA mixtures, DIP–STRs seem to be an even better tool for the detection of fetal alleles in DNA isolated from the plasma of pregnant women.
Every child inherits one allele from its mother and one from its father. The plasma of women with singleton pregnancies should contain one additional STR or DIP–STR allele inherited from a father, assuming that it is different to maternal alleles. Thus, detection of two additional nonmaternal STR or DIP–STR alleles in a plasma sample of a pregnant woman indicates a DZ twin pregnancy. The aim of our study was to assess usefulness of genotyping of STR and DIP–STR polymorphism in maternal plasma cfDNA as a cost-effective supplementary test for noninvasive prenatal diagnosis of twin zygosity in cases when chorionicity and zygosity cannot be reliably determined by ultrasound examination and a prognostic value may be provided by a DNA test determining pregnancy zygosity.
Materials and Methods
Materials
Samples from 28 twin pregnancies, obtained from the patients of the Department of Obstetrics at the Medical University of Gdańsk and from the patients of the Department of Obstetrics and Gynaecology at the St. Adalbert Hospital in Gdańsk, Copernicus Podmiot Leczniczy Sp. z o.o., were used in the study. For additional comparison of the amount of fetal DNA in singleton and twin pregnancies, samples from 15 singleton pregnancies with a male fetus were used. Samples of 3 mL of peripheral blood were collected from pregnant women (23–41 weeks of gestation, age: 22–46 years) into BD Vacutainer Plus Blood Collection Tubes (BD, Plymouth, UK) with K3-EDTA and stored at 2–8 °C for further processing. All samples of maternal peripheral blood were divided into two portions: 0.6 and 2.4 mL. The first portion (0.6 mL) was stored at −20 °C as reference material for determination of maternal DNA profile, while the remaining part (2.4 mL) was centrifuged within 8 h of sampling (Barrett et al., Reference Barrett, Zimmermann, Wang, Holloway and Chitty2011) in order to separate plasma (10 min at 1600 × g). The obtained plasma was centrifuged again in order to separate the remaining cell components (10 min at 16,000 × g; Ying et al., Reference Ying, Zimmermann, Rusterholz, Kang, Holzgreve and Hahn2004). Immediately after delivery, about 1.5 mL of umbilical cord blood was collected from each twin (56 samples in total) from the side of placenta after removal of the umbilical cord and stored at –20 °C until the time of DNA isolation. The study was approved by the Independent Bioethics Commission for Research at the Medical University of Gdańsk, and all patients provided their informed written consent for participation in the study.
DNA Isolation and Measurement of DNA Concentration
cfDNA was isolated from plasma with the use of QIAamp DSP Virus Spin Kit (Qiagen, Hilden, Germany) in accordance with the manufacturer’s manual, while DNA from maternal whole peripheral blood (the frozen portions of 0.6 mL) and from umbilical cord blood was isolated with the use of a nonenzymatic method (Lahiri & Nurnberger, Reference Lahiri and Nurnberger1991). Concentration of total human and male DNA isolated from plasma samples for singleton and twin pregnancies was measured by quantitative polymerase chain reaction (qPCR) with the use of Plexor HY assay (Promega; sensitivity > 0.0064 ng/µL) and 7900HT Fast Real-Time PCR System (Applied Biosystems, Foster City, CA, USA). Concentration of DNA isolated from whole peripheral blood and umbilical cord blood was measured spectrophotometrically with the use of ND-1000 spectrophotometer (NanoDrop Technologies, Rockland, DE, USA; sensitivity > 0.5 ng/µL).
Amplification of STR and DIP–STR Markers
Commercial PowerPlex Fusion kit (Promega, Madison, WI, USA) was used to analyze polymorphism of 22 autosomal STRs (D1S1656, D2S441, D2S1338, D3S1358, D5S818, D7S820, D8S1179, D10S1248, D12S391, D13S317, D16S539, D18S51, D19S433, D21S11, D22S1045, CSF1PO, FGA, Penta D, Penta E, TH01, TPOX, vWA), one Y-chromosomal STR (DYS391) and a sex marker (amelogenin), with primers labeled with four different fluorescent dyes. PCR was conducted in Mastercycler Gradient thermal cycler (Eppendorf, Hamburg, Germany) in the total volume of 5 μL, containing 1 ng of genomic DNA from whole peripheral/umbilical cord blood or 1 ng of cfDNA isolated from plasma. For low cfDNA concentrations (<0.4 ng/μL), in order to allow addition of 1 ng of cfDNA to the reaction, the total volume of reaction mixture was increased to 10 μL. Except for the aforementioned modifications, DNA amplification performed with the use of PowerPlex Fusion kit was carried out in accordance with the manufacturer’s manual.
DIP–STR polymorphism was analyzed with the use of two homemade multiplex PCR systems, developed separately for amplification of deletion alleles (S) and insertion alleles (L). The analysis included DIP–STR markers with tri- and tetranucleotide repeats described so far (Castella et al., Reference Castella, Gervaix and Hall2013; Oldoni et al., Reference Oldoni, Castella and Hall2015), rejecting dinucleotide markers due to high stutter ratio and resulting difficulties in interpreting DNA mixtures. While designing multiplex systems, a possibility of primer dimer formation (by AutoDimer software; Vallone & Butler, Reference Vallone and Butler2004) and of overlapping of allele size ranges was verified. From each pair of markers with primers forming dimers, a marker with higher polymorphism (higher observed heterozygosity) was selected. Primers with overlapping allele size ranges were labeled with different fluorescent dyes. The last stage of multiplex design involved rejection of DIP–STR markers whose primers generated numerous nonspecific products, that is, rs71070706-STR, rs72406828-STR and rs146332920-STR, when added to the reaction mixture. Finally, each developed multiplex included seven DIP–STR markers and the amelogenin locus as the sex marker (Table 1).
Table 1. DIP–STR markers used in the study

For both homemade DIP–STR multiplex systems, PCR was conducted with the use of Multiplex PCR Plus Kit (Qiagen) in GeneAmp PCR System 9700 thermal cycler (Applied Biosystems) in the total volume of 5 μL containing 1 ng of genomic DNA from peripheral/umbilical cord blood or 1 ng of cfDNA isolated from plasma and primers with the final concentrations presented in Table 1. The 5′ ends of forward primers were labeled with three different fluorescent dyes (Table 1). For low cfDNA concentrations (<0.4 ng/μL), in order to allow addition of 1 ng of cfDNA to the reaction, the total volume of reaction mixture was increased to 10 μL. The thermal profile included 5 min of initial denaturation at 95 °C, 30 cycles of amplification (1 min at 94 °C, 1 min at 62 °C, 1 min at 72 °C) and 60 min of final elongation at 72 °C.
Capillary Electrophoresis of Amplification Products
A total of 9 μL of Super-DI Formamide (MCLAB, South San Francisco, CA, USA) were supplemented with 1 μL of amplification products and with 0.4 μL of ILS 500 size standard (Promega) for PowerPlex Fusion kit or 0.4 μL of Orange DNA Size Standard (MCLAB) for DIP–STR multiplexes. The samples were denatured for 2 min at 95 °C and immediately cooled for 3 min on ice. Subsequently, PCR products were injected for 5 s at 3 kV and separated by capillary electrophoresis at 60 °C and 15 kV in 3130 Genetic Analyzer (Applied Biosystems) with the use of NanoPOP-4 polymer (MCLAB) and a 36-cm 4-capillary array. Genotypes of the analyzed samples were determined with the use of GeneMapper ID software v3.2 (Applied Biosystems) by comparison to an allelic ladder in case of PowerPlex Fusion kit or to a CEPH 1347-02 reference sample (Applied Biosystems) with a known, published DIP–STR genotype (Castella et al., Reference Castella, Gervaix and Hall2013; Oldoni et al., Reference Oldoni, Castella and Hall2015).
Statistical Analysis
Male DNA fraction in plasma cfDNA was calculated as a ratio of male DNA concentration and total human DNA concentration, measured by qPCR. A nonparametric Mann–Whitney U test was carried out using STATISTICA 12 software (StatSoft, Tulsa, OK, USA) to test for differences between STR and DIP–STR markers in their ability to detect fetal alleles in maternal plasma and to correctly diagnose dizygosity. The same software was employed to assess correlation between the fraction of male DNA in plasma cfDNA and the number of STR or DIP–STR markers with at least one detected fetal allele.
Results
At least one fetal STR allele was observed in each of 28 plasma samples obtained from women with twin pregnancies. In case of DIP–STR markers, two pregnancies (7.1%) revealed no fetal alleles. On the average, fetal alleles were detected in 37.2% (range: 4.5–68.2%) and 39.3% (range: 0–71.4%) of the tested 22 STRs and 7 DIP–STRs per twin pregnancy, respectively. Control DNA samples of the umbilical cord blood from twins coming from 28 pregnancies showed 15 twin-pairs with different genotypes (DZ pregnancies, 53.6%) and 13 twin-pairs with identical genotypes (MZ pregnancies, 46.4%). In case of MZ pregnancies, blood plasma collected during pregnancy always contained at most one additional STR or DIP–STR allele in comparison to maternal DNA profile obtained from peripheral blood. In case of DZ pregnancies, two additional nonmaternal alleles, clearly indicating DZ twin pregnancy, were detected in 10 plasma samples (66.7%) for STRs and in 2 plasma samples (13.3%) for DIP–STRs. Both twin pregnancies identified as DZ by DIP–STR typing of plasma cfDNA were also identified as DZ by STR typing. Examples of DNA profiles indicative of DZ twin pregnancy for STR and DIP–STR are shown in Figures 1 and 2.

Fig. 1. STR typing of cfDNA from maternal plasma, indicating DZ twin pregnancy, collated with DNA profiles of the mother and two twins (results for marker TH01 from PowerPlex Fusion kit). Two higher peaks correspond to maternal alleles (6 and 8), which were also observed in DNA extracted from maternal peripheral blood (genotype of a mother: 6,8). Two lower peaks correspond to fetal alleles (7 and 9.3), which were later detected in umbilical cord blood samples of twins after the birth (genotypes of twins: 7,8 and 6,9.3). Detection of fetal DNA by STR typing is possible when it constitutes at least 5% of cfDNA.
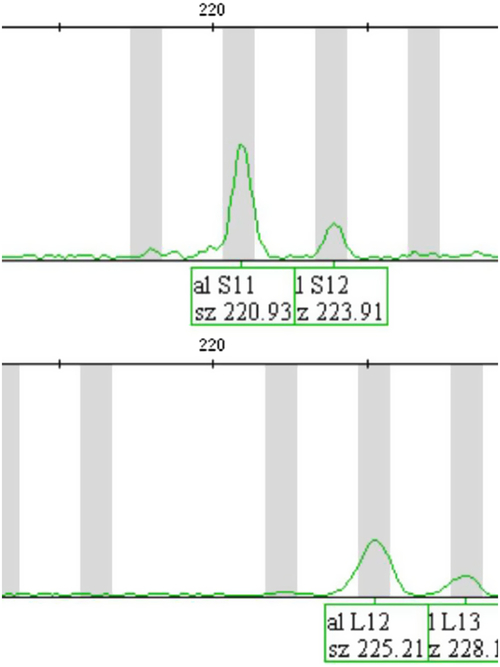
Fig. 2. DIP–STR typing of cfDNA from maternal plasma, indicating DZ twin pregnancy (a result for marker rs2308142-STR). The upper panel shows maternal alleles (S11 and S12) amplified by a multiplex PCR system for analysis of alleles with deletion (S alleles), which were also observed in DNA extracted from maternal peripheral blood (genotype of a mother: S11,S12). The lower panel shows fetal alleles (L12 and L13) amplified by a multiplex PCR system for analysis of alleles with insertion (L alleles), which were later detected in umbilical cord blood samples of twins after the birth (genotypes of twins: S12,L12 and S12,L13). As S and L alleles are amplified by different primer pairs in two separate multiplex PCR systems, detection of fetal DNA in this case is possible even when it constitutes only 0.1% of cfDNA.
In order to compare which polymorphic markers, STRs or DIP–STRs, are more robust to detect twin pregnancy dizygosity, we counted for each marker the number and percent of twin pregnancies in which at least one fetal allele was detected, and the number and percent of DZ twin pregnancies, in which two fetal alleles (indicating dizygosity of pregnancy) were detected (Table 2). No statistically significant differences between STRs and DIP–STRs were observed in the ability to detect fetal alleles in plasma cfDNA (37.2% vs. 39.3% of twin pregnancies, on average, respectively; Mann–Whitney U test: p = .57). Fetal alleles in plasma cfDNA were the most frequently observed for D12S391 (78.6% of twin pregnancies), and the least frequently for D5S818 and TPOX (7.1% of twin pregnancies). Among DIP–STRs, the most useful marker to detect fetal alleles in plasma cfDNA was rs2308142-STR (53.6% of twin pregnancies), while the least useful one was rs111478323-STR (25.0% of twin pregnancies). Similarly, no statistically significant differences between STRs and DIP–STRs were observed in the ability to detect DZ pregnancy (5.5% vs. 2.9% of twin pregnancies, on average, respectively; Mann–Whitney U test: p = .67). Two fetal alleles indicating DZ pregnancy were observed the most frequently for D8S1179 and D12S391 (26.7% of DZ twin pregnancies). rs2308142-STR also appeared to be the most useful marker to detect dizygosity among DIP–STRs (13.3% of DZ twin pregnancies).
Table 2. Comparison of the tested STR and DIP–STR markers in their ability to detect fetal alleles in maternal plasma cfDNA and to detect dizygosity of twin pregnancy

The mean concentration of cfDNA isolated from plasma was 0.56 ng/μL for twin pregnancies and 0.38 ng/μL for singleton pregnancies. For twin pregnancies with female fetuses, DNA concentration measurement by qPCR did not show presence of male DNA in any of the plasma samples. For singleton or twin pregnancies with at least one male fetus, male DNA constituted on average 6.0% of total cfDNA (range: 0.6–20.9%), corresponding to the mean concentration of male DNA equal to 0.031 ng/µL. A significantly higher fraction of fetal DNA (corresponding to male DNA measured by qPCR in pregnancies without female fetuses) was observed in twin pregnancies with two male fetuses (9.3% on average) in comparison with singleton pregnancies with one male fetus (4.6% on average; the Mann–Whitney U test: p = .04).
No correlation between the fraction of male DNA in plasma cfDNA and the number of STR markers that showed presence of at least one fetal allele was observed for 10 twin pregnancies with two male fetuses, regardless of their zygosity (R 2 = .36, p = .07), and for 5 twin pregnancies with one male fetus (R 2 = .75, p = .06), but, as reflected in p values, it was close to statistical significance in both groups of twin pregnancies. Similarly, no correlation between the fraction of male DNA in plasma cfDNA and the number of DIP–STR markers that showed the presence of at least one fetal allele was seen in twin pregnancies with two male fetuses (R 2 = .28, p = .12) and in twin pregnancies with one male fetus (R 2 = .03, p = .79).
Discussion
Zygosity of twins is frequently determined in the postnatal period to satisfy the parents’ curiosity, and also for the purpose of potential tissue and organ donation, and to predict the risk of genetic diseases (Brown, Reference Brown2015; Craig et al., Reference Craig, Segal, Umstad, Cutler, Keogh, Hopper and Harris2015). Determination of zygosity of twins is possible after birth on the basis of the sex of twins, pregnancy chorionicity, dermatoglyphs, serological examination and DNA typing (Adamski et al., Reference Adamski, Ciach, Kiełbratowska, Szczerkowska and Preis2016; Derom et al., Reference Derom, Bakker, Vlietinck, Derom, van den Berghe, Thiery and Pearson1985; Derom et al., Reference Derom, Bryan, Derom, Keith and Vlietinck2001). As sex and chorionicity of the twin pregnancy may also be established in the ultrasound prenatal examination, it is possible to assess zygosity before delivery as well.
It is assumed that it is more important for the course of twin pregnancy to know its chorionicity than zygosity (Bajoria & Kingdom, Reference Bajoria and Kingdom1997; Carroll et al., Reference Carroll, Tyfield, Reeve, Porter, Soothill and Kyle2005). Diagnosis of chorionicity is performed routinely by high-resolution ultrasound examination based on intertwin membrane thickness and the presence or absence of lambda or T sign between 10 and 14 weeks (Sepulveda et al., Reference Sepulveda, Sebire, Hughes, Odibo and Nicolaides1996). However, in the midpregnancy, this evaluation becomes unclear, and there are patients who miss their early pregnancy scan. Moreover, it is not always possible to ascertain chorionicity by ultrasound examination due to technical limitations, intrauterine crowding of fetuses, reduced amount of amniotic fluid and obesity of a pregnant woman (Lee et al., Reference Lee, Oh, Lee, Kim and Jun2010). Prediction of risk for twin pregnancy is even more complicated by false positive ultrasound diagnoses of dichorionicity (Yamashita et al., Reference Yamashita, Ishii, Hidaka, Yonetani, Hayashi, Takeuchi and Mitsuda2015) and by such phenomena as fusion of chorions in DZ pregnancy or division of a chorion in MZ pregnancy (Arabin & van Eyck, Reference Arabin and van Eyck2001; Benson & Doubilet, Reference Benson and Doubilet1991, Reference Benson and Doubilet2000; Dalton & Dudley, Reference Dalton and Dudley1989; Hickey & Goldberg, Reference Hickey and Goldberg1996;). In all the aforementioned cases, a DNA test to determine pregnancy zygosity may have a prognostic value (Appelman et al., Reference Appelman, Manor, Magal, Caspi, Shohat and Blickstein1994; Norton et al., Reference Norton, D’Alton and Bianchi1997). Although circa 50% of DZ pregnancies may be identified by ultrasound examination due to opposite sex of twins, there are known a number of cases of sex-discordant MZ twins (Bohec et al., Reference Bohec, Douet-Guilbert, Basinko, Le Bris, Marcorelles, Audrézet and De Braekeleer2010; Wachtel et al., Reference Wachtel, Somkuti and Schinfeld2000). Diagnosis of dizygosity indicates an extremely low risk for TTTS, which affects as much as 5–10% of MZ pregnancies (Simpson & Miller, Reference Simpson, Miller, Copel, D’Alton, Feltovich, Gratacós, Krakow, Odibo, Platt and Tutschek2018) and was reported only in several cases of DZ pregnancies in the world (Chen et al., Reference Chen, Chmait, Vanderbilt, Wu and Randolph2013; McNamara et al., Reference McNamara, Kane, Craig, Short and Umstad2016). Furthermore, observation of malformations in only one twin with confirmed dizygosity of pregnancy could restrict invasive diagnostic procedures to only one fetus.
Initially, prenatal determination of zygosity in multiple pregnancies based on analysis of DNA polymorphism was conducted with the use of biological material collected during amniocentesis, chorionic villus biopsy or cordocentesis (Chen et al., Reference Chen, Chern and Wang2000; Cirigliano et al., Reference Cirigliano, Cañadas, Plaja, Ordoñez, Mediano, Sánchez and Farrán2003; Fang et al., Reference Fang, An, Wu, Liu and Feng2004; Kovacs et al., Reference Kovacs, Shahbahrami, Platt and Comings1988; Levy et al., Reference Levy, Mirlesse, Jacquemard and Daffos2002). In order to determine zygosity on the basis of DNA typing of such samples, molecular probes were used to analyze restriction fragment length polymorphism (Azuma et al., Reference Azuma, Kamiura, Nobunaga, Negoro, Saji and Tanizawa1989; Kovacs et al., Reference Kovacs, Shahbahrami, Platt and Comings1988) or polymorphism of sequences of PCR products (Levy et al., Reference Levy, Mirlesse, Jacquemard and Daffos2002). Later, quantitative fluorescent PCR of STR markers was implemented for this purpose (Chen et al., Reference Chen, Chern and Wang2000; Cirigliano et al., Reference Cirigliano, Cañadas, Plaja, Ordoñez, Mediano, Sánchez and Farrán2003; Fang et al., Reference Fang, An, Wu, Liu and Feng2004). However, amniocentesis, chorionic villus biopsy and cordocentesis are invasive for pregnancy and are characterized by a high risk of complications. New possibilities for diagnosis of pregnancy zygosity have appeared with studies of free fetal DNA circulating in the mother’s blood. Smid et al. (Reference Smid, Galbiati, Vassallo, Gambini, Ferrari, Restagno and Cremonesi2003) performed quantification of the SRY gene located on the Y chromosome in plasma of pregnant women and showed that the amount of male DNA in cfDNA is correlated with the number of male fetuses. These findings are in accordance with the results of our study based on qPCR of male DNA, which showed that the amount of fetal DNA in plasma of women with twin pregnancies is approximately twice as high as in singleton pregnancies, which confirms the possibility to use cfDNA from maternal plasma in prenatal genetic analysis of twins.
As we have found no studies of pregnancy zygosity testing based on STR polymorphism in cfDNA isolated from plasma of pregnant women, the aim of our study was to analyze the usefulness of microsatellite markers in the diagnosis of zygosity of twin pregnancies. Our preliminary results based on 22 STR loci have shown significant usefulness of STR genotyping in cfDNA of maternal plasma for prenatal diagnosis of zygosity of twin pregnancies. Regarding detection of dizygosity of twin pregnancies, the set of 22 STR markers showed 67% sensitivity, 100% specificity and 82% accuracy. It means that after genotyping of 22 STRs, roughly two-thirds of DZ twin pregnancies may be classified as low-risk twin pregnancies, narrowing the number of cases for strict and frequent ultrasound supervision and reducing costs of prenatal care. Also, as discussed later, it should be noted that accuracy of an assay seems to depend on the number of tested markers. The newest capillary electrophoresis instruments, yet unreleased to the market (e.g., Spectrum CE System from Promega), employ 8-dye technology that roughly doubles the number of markers that may be included in one multiplex PCR system and will allow development of new STR assays with much higher sensitivity for detection of DZ twin pregnancies than the PowerPlex Fusion kit used in our preliminary study.
The majority of cfDNA isolated from the plasma of a pregnant woman is maternal DNA, while the minority component (in this case, fetal DNA) may not be detected by STR typing if it constitutes less than 5% of the mixture (1:20 ratio; Butler, Reference Butler2015). As fetal DNA constitutes on the average 3–20% of the total plasma cfDNA, fetal alleles may remain undetected in analysis of STR polymorphism by quantitative fluorescent PCR. For this reason, our attention was turned to DIP–STR markers, which enable detection of alleles of the minority component (in our case, fetal DNA) in the presence of the majority component (in our case, maternal DNA) even when the ratio of both components is 1:1000 (Castella et al., Reference Castella, Gervaix and Hall2013). Castella et al. (Reference Castella, Gervaix and Hall2013) demonstrated the usefulness of genotyping of DIP–STR markers in DNA mixtures also in the case of feto-maternal microchimerism. Our results of DIP–STR polymorphism analysis in plasma samples from women with twin pregnancies showed lower usefulness of the analyzed set of DIP–STR markers in detecting dizygosity of pregnancy (13% sensitivity, 100% specificity, 54% accuracy) in comparison to the PowerPlex Fusion kit. However, statistical analysis revealed no significant differences between the tested STRs and DIP–STRs in capability to diagnose dizygosity of twin pregnancies, which suggests that the higher usefulness of the PowerPlex Fusion kit in comparison with the homemade multiplexes of DIP–STR markers merely results from a higher number of markers included in the PowerPlex Fusion kit (22 STRs vs. 7 DIP–STRs). It should also be noted that our preliminary study was conducted on women up to 41 weeks of gestation, when the fetal DNA content in plasma is the highest. It is possible that limitation of the studied group to pregnant women in early pregnancy, when fetal DNA constitutes only 3–10% of plasma cfDNA, would show an advantage of DIP–STR markers over typical microsatellites.
Although correlation between the fraction of male DNA (corresponding to the fraction of fetal DNA in twin pregnancies without female fetuses) and the number of detected fetal alleles was not observed, it was very close to statistical significance in the case of STRs, but not in the case of DIP–STRs. It may result from the fact that in the case of the latter, detection of minority alleles does not depend so strongly on the fraction of fetal DNA as far as DIP–STR alleles may be successfully genotyped even if the minority component (fetal DNA) constitutes much less than 5% of the DNA mixture (plasma cfDNA). However, these observations were based on only 10 twin pregnancies with two male fetuses and 5 twin pregnancies with one male fetus, so further studies with larger numbers of cases are required to confirm the higher robustness of DIP–STRs in prenatal zygosity testing of twin pregnancies. Nevertheless, p values at the limit of statistical significance for STR markers indicate that detection of twin pregnancy dizygosity by the STR assay is more likely to depend on the fraction of fetal DNA. Thus, sensitivity of the STR assay will grow with gestational age and will be higher if release of maternal cfDNA is prevented; for example, by stabilization of maternal blood cells after sample collection. In our study, blood samples collected in K3-EDTA tubes were used for isolation of plasma cfDNA within 8 h of sample collection (Barrett et al., Reference Barrett, Zimmermann, Wang, Holloway and Chitty2011), so the impact of maternal cfDNA release on the results seems to be negligible.
Determination of zygosity of twin pregnancies on the basis of cfDNA from plasma of pregnant women is also currently possible with the use of massively parallel sequencing (MPS; Qu et al., Reference Qu, Leung, Jiang, Liao, Cheng, Sun and Lo2013; Zheng et al., Reference Zheng, Xu, Guo, Wei, Ge, Li and Jiang2013). However, this method requires relatively large amounts of cfDNA, reaching up to 30 ng (Qu et al., Reference Qu, Leung, Jiang, Liao, Cheng, Sun and Lo2013), while the amount of DNA obtained from maternal plasma is often scarce (in our study, 10 ng from 200 μL of plasma, on average) and would not allow determination of zygosity with the use of MPS in many our patients, even if cfDNA was isolated from 600 μL of plasma, as in the study by Zheng et al. (Reference Zheng, Xu, Guo, Wei, Ge, Li and Jiang2013). Moreover, MPS is associated with relatively high costs, reaching several hundred US dollars for one patient. On the contrary, a total cost of zygosity testing with the use of our sets of STR or DIP–STR markers does not exceed USD10 for one patient with a twin pregnancy. Furthermore, twin pregnancy zygosity testing by STR and DIP–STR genotyping is very rapid and simple, whereas MPS requires laborious sample processing and advanced bioinformatic analyses. As shown in Figures 1 and 2, interpretation of STR and DIP–STR profiles is straightforward and unequivocal with four alleles detected at one or more STR/DIP–STR markers indicating DZ twins. It should also be emphasized that studies of zygosity involving the MPS method published so far were based only on a few cases of twin pregnancies (eight cases studied by Qu et al., Reference Qu, Leung, Jiang, Liao, Cheng, Sun and Lo2013 and four cases studied by Zheng et al., Reference Zheng, Xu, Guo, Wei, Ge, Li and Jiang2013), while our preliminary study was based on 28 twin pregnancies.
A certain limitation of our assays is the risk of a false positive result (false detection of DZ pregnancy) in the case of trisomy resulting from paternal nondisjunction. Therefore, detection of four alleles at STR or DIP–STR markers located on one chromosome, especially on chromosome 21, 18 or 13, should be diagnosed as a result indicating DZ twin pregnancy only if trisomy is excluded by other tests. Detection of four alleles is also possible in MZ twin pregnancy if pregnancy results from in vitro fertilization with egg cell donation. Therefore, diagnosis of zygosity of twin pregnancy should always be preceded by medical history regarding assisted reproduction technology.
Owing to 100% specificity and lower sensitivity, results of genotyping of STR/DIP–STR markers in plasma cfDNA indicate either that a twin pregnancy is DZ or that zygosity status is undetermined. Thus, prenatal diagnosis of twin dizygosity does not need to be confirmed after birth.
In summary, relatively high sensitivity, specificity and accuracy of the assays, low DNA input requirements, low costs and simplicity of the analysis make genotyping of STR/DIP–STR markers a useful tool for noninvasive prenatal diagnosis of zygosity of twin pregnancies.
Financial support
This research received no specific grant from any funding agency, commercial or not-for-profit sectors.
Conflict of interest
None.
Ethical standards
The authors assert that all procedures contributing to this work comply with the ethical standards of the relevant national and institutional committees on human experimentation and with the Helsinki Declaration of 1975, as revised in 2008.