1. Introduction
One of the remarkable abilities of bilinguals is to be able to continuously control the use of the two languages. To do this in an efficient way, bilinguals need to monitor the language they intend to speak and, at the same time, avoid interference from the non-selected language. Green and Abutalebi (Reference Green and Abutalebi2013), in their adaptive control hypothesis, proposed that goal maintenance, conflict monitoring, and interference suppression are key language control processes that are at play when bilinguals restrict their communication to one language (single-language context) and to a greater extent when they switch back and forth between languages (dual-language context). At the neural level, several brain areas are crucially activated for these control processes. Prefrontal areas and the anterior cingulate cortex (ACC) have been directly related to monitoring and cross-language interference suppression. Posterior and subcortical areas (mainly basal ganglia) seem to be more activated during language maintenance and selection (Abutalebi & Green, Reference Abutalebi and Green2016; Calabria et al., Reference Calabria, Costa, Green and Abutalebi2018; Sulpizio et al., Reference Sulpizio, Del Maschio, Fedeli and Abutalebi2020).
Similarly, neuropsychological research in bilingual patients supports the involvement of these areas in language maintenance, monitoring, and interference suppression in two ways. First, prefrontal and subcortical lesions may produce language maintenance deficits such as involuntary language switching, or deficits of interference suppression that result in involuntary language mixing at word or sentence level (Abutalebi et al., Reference Abutalebi, Miozzo and Cappa2000; Aglioti & Fabbro, Reference Aglioti and Fabbro1993; Ansaldo et al., Reference Ansaldo, Saidi and Ruiz2010; Calabria et al., Reference Calabria, Marne, Romero-Pinel, Juncadella and Costa2014; Fabbro et al., Reference Fabbro, Skrap and Aglioti2000; García-Caballero et al., Reference García-Caballero, García-Lado, González-Hermida, Area, Recimil, Juncos Rabadán, Lamas, Ozaita and Jorge2007; Kong et al., Reference Kong, Abutalebi, Lam and Weekes2014; Leemann et al., Reference Leemann, Laganaro, Schwitter and Schnider2007; Mariën et al., Reference Mariën, Abutalebi, Engelborghs and De Deyn2005). Second, research with bilingual patients with neurodegenerative diseases suggests that the origin of their language switching deficits might be related to their deficits in interference suppression or inhibitory control (Cattaneo et al., Reference Cattaneo, Calabria, Marne, Gironell, Abutalebi and Costa2015, Reference Cattaneo, Costa, Gironell and Calabria2020).
To explore the role of interference suppression in bilingual language control, in this study we investigate the performance of bilingual patients with neurodegenerative disorders in a cross-language version of the Stroop task. Specifically, we explore the performance of individuals with Parkinson's disease (PD), Mild Cognitive Impairment (MCI), and Alzheimer's disease (AD). These neurodegenerative disorders have common functional or structural changes over the posterior parietal areas (Sampedro et al., Reference Sampedro, Marín-Lahoz, Martínez-Horta, Camacho, Lopez-Mora, Pagonabarraga and Kulisevsky2021; Talwar et al., Reference Talwar, Kushwaha, Chaturvedi and Mahajan2021). However, the hallmarks of each one are different and they are considered to be distinct clinical entities. For PD, it is frontostriatal dysfunction that leads to executive control deficits (e.g., Williams-Gray et al., Reference Williams-Gray, Evans, Goris, Foltynie, Ban, Robbins, Brayne, Kolachana, Weinberger, Sawcer and Barker2009). For MCI and AD, the neural hallmark is the entorhinal cortex and hippocampus that lead to long-term memory deficits as a first cognitive symptom, but also for executive control (Talwar et al., Reference Talwar, Kushwaha, Chaturvedi and Mahajan2021). Therefore, as these neurodegenerative conditions affect different brain networks, we can expect distinct language control deficits based on the neural architecture of bilingual language control (Abutalebi & Green, Reference Abutalebi and Green2008; Calabria et al., Reference Calabria, Costa, Green and Abutalebi2018; Green & Abutalebi, Reference Green and Abutalebi2008).
1.1. Cross-language interference in the Stroop task
Interference in bilingual speech production has been investigated by using tasks that require the simultaneous processing of the two languages by manipulating lexical, phonological, and semantic levels. Examples of such tasks are the word-picture interference naming task (e.g., Costa et al., Reference Costa, Miozzo and Caramazza1999; Costa & Caramazza, Reference Costa and Caramazza1999; Finkbeiner et al., Reference Finkbeiner, Almeida, Janssen and Caramazza2006a, Reference Finkbeiner, Gollan and Caramazza2006b; La Heij, Reference La Heij, Kroll and de Groot2005) and the Stroop task (e.g., Costa et al., Reference Costa, Albareda and Santesteban2008).
The Stroop task is crucially relevant as it was the task used in the present study. We adapted the version employed by Costa et al. (Reference Costa, Albareda and Santesteban2008) in which Catalan–Spanish young bilinguals were presented with words written in their non-dominant language (L2, Spanish) and were required to name the ink colour of the words in their dominant language (L1, Catalan). There were three conditions: 1) congruent, in which coloured words matched their ink colour (e.g., amarillo, ‘yellow’ in Spanish, ink colour yellow), 2) incongruent, in which coloured words did not match their ink colour (e.g., rojo, ‘red’ in Spanish, ink colour yellow), or 3) control, with non-coloured words (e.g., lleno, ‘full’ in Spanish, ink colour yellow). By comparing these three conditions, Costa et al. (Reference Costa, Albareda and Santesteban2008) found two main results.
First, participants showed slower naming latencies when required to name the ink colour in the incongruent condition (not matching with the colour name of the word) than in the control condition. This was interpreted as an interference effect of the semantically related words in the participants’ L1 activated by L2 words. In this cross-language condition, bilinguals have to filter out irrelevant information (semantically related words) that is activated by reading colour names in L2. This is one of the mechanisms underlying the Stroop effect that is known as interference suppression (e.g., Dulaney & Rogers, Reference Dulaney and Rogers1994; Esposito et al., Reference Esposito, Baker-Ward and Mueller2013). Similarly, in monolinguals interference suppression is needed because of the conflict generated by the automatic reading of the semantically related colour word (MacLeod, Reference MacLeod1991; for a review, Scarpina & Tagini, Reference Scarpina and Tagini2017).
Second, when the written word was the translation equivalent in the non-dominant language of the ink colour, participants were faster compared to the control condition. This effect, called the cross-language identity effect, indicates that the simultaneous activation of both languages does not result in a language competition, as opposed to interference in the Stroop condition. Similar results of interference and facilitation effects were reported in a study by Roelofs (Reference Roelofs2010) with Dutch–English bilinguals.
The interference suppression applied during the Stroop task seems to be produced at different levels of processing and there is no consensus among researchers about this point. This includes the semantic and response levels, and task configuration (for a discussion see Parris et al., Reference Parris, Augustinova and Ferrand2019, Reference Parris, Hasshim, Wadsley, Augustinova and Ferrand2022). At the semantic level, the conflict is generated from the stimulus of related items whereas, at the response level, it is related to the resolution of the conflict at the speech output level. At the task level, the conflict is generated from the automatic reading of the colour name that does not match with the ink colour that should be named. Despite there not being a clear consensus on the loci of the interference suppression, the presence of a conflict to be monitored and solved is common to all views that have tried to explain the Stroop effect. Indeed, a recent study on the construct validity of the Stroop test by Periáñez et al. (Reference Periáñez, Lubrini, García-Gutiérrez and Ríos-Lago2021) found that conflict monitoring along with working memory are the only significant predictors of the interference scores of the Stroop task. Additionally, neuroimaging studies consistently found that processing incongruent trials during the Stroop task activates the ACC, an area in which activity is associated with conflict monitoring and resolution in executive control tasks (Botvinick et al., Reference Botvinick, Carter, Braver, Barch and Cohen2001). The ACC is also the brain area within the bilingual language control network that monitors the conflict between languages during language switching tasks (for reviews, see Abutalebi & Green, Reference Abutalebi and Green2008; Sulpizio et al., Reference Sulpizio, Del Maschio, Fedeli and Abutalebi2020).
1.2. Interference in the Stroop task in neurodegenerative disorders
The research on language control in bilingual individuals with neurodegenerative disorders is limited to a few studies. Previous research has investigated the role of basal ganglia in managing the control of two languages in patients with PD (Cattaneo et al., Reference Cattaneo, Calabria, Marne, Gironell, Abutalebi and Costa2015, 2020) and Huntington's disease (HD) (Calabria et al., Reference Calabria, Pérez Pérez, Martínez-Horta, Horta-Barba, Carceller, Kulisevsky and Costa2021). These studies employed a language switching task to test whether language control abilities were affected by the frontostriatal impairments due to these two diseases. In this task, participants were required to name pictures according to the naming language that was cued by a flag. Overall, the results suggested that these two diseases may affect the control of a bilingual's two languages, as patients showed increased naming latencies in switching between languages as compared to healthy controls. Interestingly, the results from the two studies of participants with PD showed that the switching deficits were limited to control of the two languages. In their first study, Cattaneo et al. (Reference Cattaneo, Calabria, Marne, Gironell, Abutalebi and Costa2015) tested PD patients and healthy controls in a language switching task and a non-linguistic switching task. In the language switching task, participants were required to name pictures in two languages (Catalan and Spanish) according to a cue (flag). There were two types of trials: repeat and switch. In repeat trials, the target language was the same as the trial immediately encountered before, and in switch trials the target language was different than in the previous trial. Similarly, in the non-linguistic switching task, there were repeat and switch trials and participants were required to perform a matching task according to two criteria, by shape or colour. The difference in reactions times or naming latencies between switch and repeat trials is the switch cost. PD patients had larger switch costs in language switching tasks as compared to healthy controls, but they were not in a non-linguistic switching task. In a further study, Cattaneo et al. (Reference Cattaneo, Costa, Gironell and Calabria2020) found that PD patients were impaired in language switching but not in switching between grammatical categories (nouns and verbs) when the task was performed only in their dominant language.
Similarly, Calabria et al. (Reference Calabria, Pérez Pérez, Martínez-Horta, Horta-Barba, Carceller, Kulisevsky and Costa2021) found some evidence of language switching deficits in bilingual patients with HD. In that study, in healthy controls the switch costs were reduced for longer time intervals (1 second), as compared to simultaneous presentation, between the language cue and the stimulus to name. This is explained by the reduced time to reconfigure the new language and disengage from the previous one (Ma et al., Reference Ma, Li and Guo2016). Conversely, HD patients showed the same magnitude of the switch costs in both conditions, suggesting a relation between frontostriatal impairments and language reconfiguration/engagement. Switching back and forth between languages requires processes of engagement and disengagement from one language to another. In switch trials, participants need to withdraw the language in use (disengagement) then to activate or configure the other language (engagement). By manipulating the time between the cue and the stimulus, it is possible to study the time needed to engage in a new language. In our study (Calabria et al., Reference Calabria, Pérez Pérez, Martínez-Horta, Horta-Barba, Carceller, Kulisevsky and Costa2021), we found that bilingual patients with HD were only impaired in language engagement when the presentation of the cue and the stimulus was simultaneous, suggesting that they were impaired in reactivating the new language.
Interestingly, the same participants also performed a cross-language version of the Stroop task and HD patients performed in the same way as healthy controls. Therefore, the conclusion was that language engagement is to some extent separable from interference suppression, as patients were impaired in the language switching task, but not in the Stroop task.
HD is characterised by a similar basal ganglia pathology to PD, despite being neurological disorders with distinct clinical features (Montoya et al., Reference Montoya, Price, Menear and Lepage2006; Owen, Reference Owen2004). The degeneration in PD is through the nigro-striatal dopaminergic circuitry from the putamen, whereas in HD is from the striatum. Nevertheless, at cognitive level they share similar deficits for interference suppression in the Stroop task (Hsieh et al., Reference Hsieh, Chen, Wang and Lai2008; Sisco et al., Reference Sisco, Slonena, Okun, Bowers and Price2016; Snowden, Reference Snowden2017), suggesting that basal ganglia are involved in executive control. However, in our study with bilingual patients with presymptomatic HD (Calabria et al., Reference Calabria, Pérez Pérez, Martínez-Horta, Horta-Barba, Carceller, Kulisevsky and Costa2021) we did not find evidence of interference suppression deficits in the cross-language version of the Stroop task. Therefore, it remains unclear whether basal ganglia are crucially involved in cross-language interference or not. So, it would be useful to retest this hypothesis with a disease affecting these subcortical structures such as PD. This is the reason why we included bilinguals with PD in a cross-language version of the Stroop task.
Additionally, we extended the study to MCI and AD as they are two other neurodegenerative disorders that might also show language control deficits. Research in bilingual patients with MCI or AD has focused on the patterns of language deterioration (for a review, see Stilwell et al., Reference Stilwell, Dow, Lamers and Woods2016) and little is known about the impact of these disorders on cross-language interference (Mendez et al., Reference Mendez, Perryman, Ponton and Cummings1999). Nevertheless, there is evidence from monolinguals that interference suppression and other control processes are affected by AD and to some extent by MCI.
The Stroop effect increases with age. It increases more from 60 years old and declines during late adulthood in monolinguals (Bugg et al., Reference Bugg, DeLosh, Davalos and Davis2007) and bilinguals (Kousaie & Phillips, Reference Kousaie and Phillips2012; Zied et al., Reference Zied, Phillipe, Karine, Valerie, Ghislaine, Arnaud and Didier2004). This increase due to age is partially attributable to general slowing but is also attributable to age-related changes in task-specific processes such as inhibitory control (Bugg et al., Reference Bugg, DeLosh, Davalos and Davis2007), working memory, and selective attention (e.g., (Andrés & Van der Linden, Reference Andrés and Van der Linden2000; Jeffrey & Jacoby, Reference Jeffrey, Jacoby, Bialystok and Craik2006). In MCI and AD patients, the Stroop effect is larger than healthy older adults (Amieva et al., Reference Amieva, Phillips, Della Sala and Henry2004; Bélanger et al., Reference Bélanger, Belleville and Gauthier2010; Spieler et al., Reference Spieler, Balota and Faust1996), suggesting that interference suppression deficits affect the performance of patients in tasks requiring controlled inhibitory control and conflict monitoring for selecting competitive responses, such as in the Stroop task. It is generally found that patients with MCI and AD are impaired in a variety of executive control tasks, besides the primary cognitive symptoms of these disorders that primarily affect long-term memory (e.g., Duke & Kaszniak, Reference Duke and Kaszniak2000; Swanberg et al., Reference Swanberg, Tractenberg, Mohs, Thal and Cummings2004).
Interference suppression deficits are commonly associated with MCI and AD, whereas goal maintenance in control tasks seems to be partially preserved in MCI but not in AD patients (Bélanger et al., Reference Bélanger, Belleville and Gauthier2010). As in older adults, the speed of processing only explains part of the larger magnitudes of the Stroop effect found in patients, suggesting that interference suppression is a specific control deficit that might be affected by these neurodegenerative conditions (Ben-David et al., Reference Ben-David, Tewari, Shakuf and Van Lieshout2014).
1.3. The present study
We tested four groups of highly proficient Catalan–Spanish bilinguals, such as older adults, PD, MCI, and AD patients with an adapted version of the cross-language Stroop task employed by Costa et al. (Reference Costa, Albareda and Santesteban2008) in young adults. From the version used by Costa et al. (Reference Costa, Albareda and Santesteban2008) we excluded the phono-translation condition (the printed word phonologically overlaps with the colour name).
This task allows testing both cross-language interference and facilitation. Interference is measured by the difference in naming latencies between incongruent (colour words not matching their ink colour) and control (non-colour words) conditions. This is also known as the Stroop effect. Facilitation is determined by the difference in naming latencies between the control and congruent (colour words matching their ink colour) conditions. The cross-language interference effect has been explained by the competition mechanisms during lexico-semantic selection within- and between-languages. Nevertheless, there is less consensus about the explanation of the facilitation effect, although it is thought to be independent of interference as it cannot be explained in terms of lexical competition (Costa et al., Reference Costa, Albareda and Santesteban2008; Roelofs, Reference Roelofs2010; Roelofs & Hagoort, Reference Roelofs and Hagoort2002).
The crucial comparison in this study is between patient groups. Abutalebi and Green (Reference Abutalebi and Green2007), in their neural model of bilingual speech production, proposed that both cortical and subcortical areas are involved in the control of two languages. Therefore, the comparison of AD, MCI, and PD offers an opportunity to investigate how cortical and subcortical damage may affect speech production in bilinguals, especially for cross-language interference.
For interference, we expected to find a larger Stroop effect in patients with MCI and AD. This hypothesis is based on the previous literature on monolingual speakers that suggests larger semantic interference effects in these patients compared to healthy controls (for a review, see Ben-David et al., Reference Ben-David, Tewari, Shakuf and Van Lieshout2014). There are only a few exceptions that indicate similar interference effects between AD patients and healthy controls (Spieler et al., Reference Spieler, Balota and Faust1996). Similarly, PD patients should show larger interference effects according to monolingual data (Hsieh et al., Reference Hsieh, Chen, Wang and Lai2008; Sisco et al., Reference Sisco, Slonena, Okun, Bowers and Price2016), even if, in some cases, its magnitude may be modulated by medication (Djamshidian et al., Reference Djamshidian, O'Sullivan, Lees and Averbeck2011). These authors found that the magnitude of the interference was reduced when patients performed the task in ‘on’ condition (they had just taken the L-dopa medication) as compared to when they were in ‘off’ (they had not taken their medication for at least 12 hours).
In the present study, the performance of bilingual patients with PD is informative about the role of the subcortical structures in suppressing the interference from the other language (written words in Spanish). According to the neural model of bilingualism proposed by Abutalebi and Green (Reference Abutalebi and Green2007), basal ganglia and their connections with the frontal areas are involved in the selection and suppression of lexical alternatives between two competing responses (languages).
For facilitation, we expected that patients would perform as did healthy controls. Facilitatory effects in the Stroop task have been little explored in patients with neurodegenerative diseases, and studies of monolinguals suggest that their magnitude can be even larger in patients with PD (Henik et al., Reference Henik, Singh, Beckley and Rafal1993) or AD (Spieler et al., Reference Spieler, Balota and Faust1996) compared to healthy controls. Indeed, the facilitation effects are explained by the automatic spreading of convergent semantic and phonological information both within- and between-languages (Roelofs, Reference Roelofs2010). As opposed to interference that is dependent on control processes (e.g., conflict monitoring and resolution), facilitation is mainly based on automatic processes of congruent information between colour words and their ink colour. It has been shown that the automatic spreading of information at the early stages of AD is spared as patients and healthy individuals perform the same in the congruent condition of the Stroop task (Faust & Balota, Reference Faust, Balota, Gorfein and MacLeod2008). Therefore, it is expected that patients would not differ from healthy controls in the congruent condition of the cross-language version of the Stroop task.
2. Methods
2.1. Participants
Sixty-two participants took part in the experiment: 16 with MCI, 16 with AD, 16 with PD, and 14 healthy controls. The groups were matched for age and years of formal education (see Table 1).
Table 1. Sociodemographic data and bilingualism profile of participants.
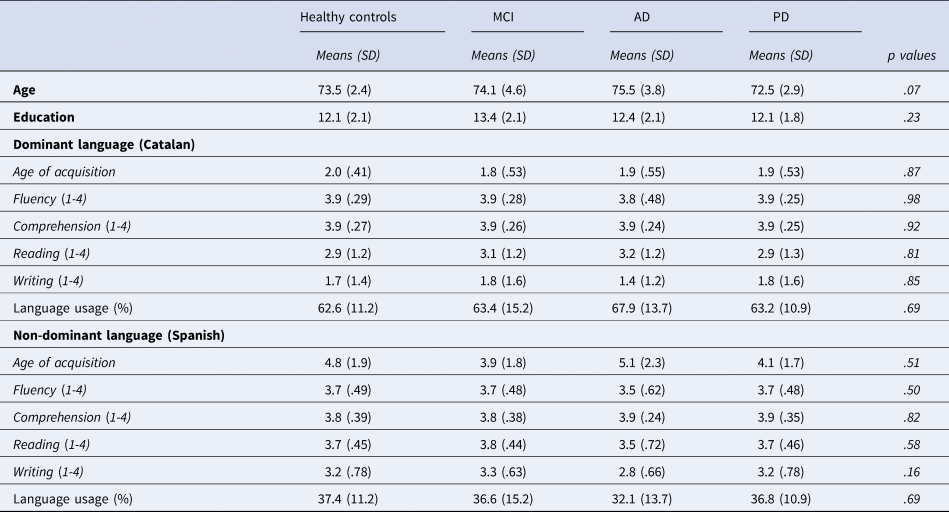
All the participants were highly proficient bilinguals, and they have Catalan as their L1 and dominant language, and Spanish as their L2 and non-dominant language. The age of acquisition of Spanish was below six years. Language proficiency was investigated through a questionnaire for comprehension, fluency, writing, and reading in Spanish and Catalan on a four-point scale. The low level of proficiency for writing and reading in Catalan is because formal education was only provided in Spanish, as Catalan was not allowed to be used at school. Language usage was calculated as a mean from infancy to adulthood of using languages in different contexts (school, family, social, and work). For details of the questionnaires that we used see Calabria et al. (Reference Calabria, Pérez Pérez, Martínez-Horta, Horta-Barba, Carceller, Kulisevsky and Costa2021).
For AD, the clinical criteria were those based on the recommendations from the National Institute on Aging–Alzheimer's Association workgroups on diagnostic guidelines for Alzheimer's disease (McKhann et al., Reference McKhann, Knopman, Chertkow, Hyman, Jack, Kawas, Klunk, Koroshetz, Manly, Mayeux, Mohs, Morris, Rossor, Scheltens, Carrillo, Thies, Weintraub and Phelps2011). According to these criteria, AD is defined as a decline with slow progression, with memory impairment as the principal feature and with an onset after the age of 65 (usually in the late 70s or thereafter).
For MCI, the diagnosis was based on the recommendations of Albert et al. (2011), consisting of the following: lower performance in one or more cognitive domains including episodic memory, independence of function in daily life, and no evidence of significant impairment in social and occupational functioning. Single-domain and multiple-domain subtypes were classified as MCI only.
For PD, the diagnosis was based on the clinical criteria of the UK Parkinson's Disease Brain Bank (Hughes et al., Reference Hughes, Daniel, Kilford and Lees1992). Based on the Unified Parkinson Disease Rating Scale (UPDRS, mean = 11.7 ± 4.2 out of 159, range = 8–21; Goetz, Reference Goetz2003), all patients were in the mild stage of the disease, and their Mini Mental State Examination (MMSE) (Folstein et al., Reference Folstein, Folstein and McHugh1975) scores indicated that they did not have dementia (mean 26.9 ± 1.4, range = 25–30).
All AD patients were receiving acetylcholinesterase inhibitors as pharmacological treatment and in the mild to moderate stage of the disease, whereas the MCI participants were not receiving any medication for their diagnosis. All PD patients were stable, without motor fluctuations, and receiving anti-Parkinsonian pharmacological treatment.
Patients with potentially confounding neurological (other than their primary diagnosis) and psychiatric disorders, clinically known hearing or vision impairments, a past history of alcohol abuse, and/or psychosis were excluded from the study. Healthy individuals had no previous neurological or psychiatric diseases. Additionally, healthy individuals were excluded from the study if they showed signs of cognitive deficits in a brief neuropsychological assessment.
To establish a common set of test scores for all the participants, we designed a brief neuropsychological assessment specifically for this study that included: a) MMSE and Global Deterioration Scale (GDS) that measured general cognitive decline; b) CERAD Word List Memory (Morris et al., Reference Morris, Heyman, Mohs, Hughes, van Belle, Fillenbaum, Mellits and Clark1989) that measured long-term episodic memory (recall and recognition); c) forward and backward digit spans (Peña-Casanova et al., Reference Peña-Casanova, Quiñones-Úbeda, Quintana-Aparicio, Aguilar, Badenes, Molinuevo, Torner, Robles, Barquero, Villanueva, Antúnez, Martínez-Parra, Frank-García, Sanz, Fernández, Alfonso, Sol and Blesa2009b) that measured short-term memory and working memory, respectively; d) Trail Making Test part A (Reitan, Reference Reitan1958) that measured processing speed and visuomotor tracking; e) Pyramids and Palm Trees Test (PPT) that measured semantic memory (Howard et al., Reference Howard and Patterson1992); f) semantic fluency that measured lexical retrieval from a semantic category (Peña-Casanova et al., Reference Peña-Casanova, Quiñones-Úbeda, Gramunt-Fombuena, Quintana-Aparicio, Aguilar, Badenes, Cerulla, Molinuevo, Ruiz, Robles, Barquero, Antúnez, Martínez-Parra, Frank-García, Fernández, Alfonso, Sol and Blesa2009a). See Table 2 for the results.
Table 2. Neuropsychological test scores of participants.
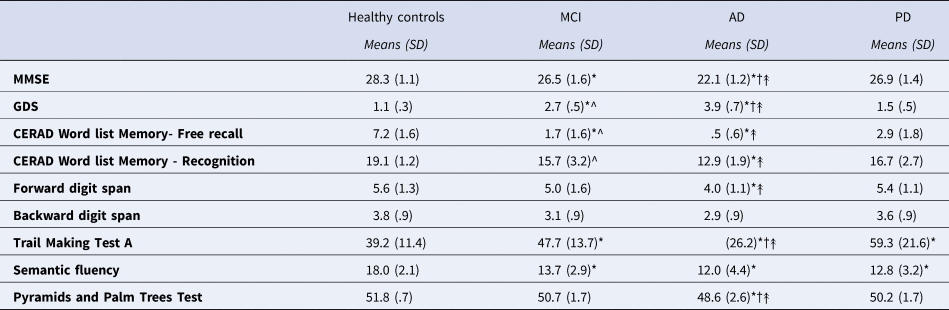
*: different from controls, p < .05; †: AD different from MCI, p < .05; ↟: AD different from PD, p < .05;
^: MCI different from PD, p < .05
MMSE: Mini Mental State Examination
GDS: Global Deterioration Scale
CERAD: Consortium to Establish a Registry for Alzheimer's Disease
2.2. Materials and procedure
Nine Spanish non-colour words [LLENO (full), CORTO (short), LIGERO (light), FELIZ (happy), SANO (healthy), SERIO (serious), ABIERTO (open), ALTO (high), and EGOISTA (selfish)] and three Spanish colour words were selected [AZUL (blue), ROJO (red), AMARILLO (yellow)] to generate the three conditions of the task (congruent, incongruent, and control). Blue, red, and yellow were selected as the ink colours. These ink colours were selected because they are not cognate words between Spanish and Catalan, so to exclude any phonological effects across languages.
The three conditions were as follows: 1) non-colour words printed in yellow, red, or blue, called the “control” condition (e.g., the word LLENO printed in yellow ink); 2) names of the colours printed with a congruent ink colour, the “congruent” condition (e.g., the word AMARILLO printed in yellow ink); and 3) names of the colours printed with a different ink colour, the “incongruent” condition (e.g., the word ROJO, printed in yellow ink).
Nine additional non-colour words [CURIOSO (curious), GRACIOSO (funny), JOVEN (young), BONITO (beautiful), LINDO (pretty), TRISTE (sad), MALO (bad), PEQUEÑO (small), ASTUTO (astute)] and three additional ink colours (orange, green, and brown) were used as fillers. Each colour was presented in combination with three different words. Fillers were included to increase the statistical power of the effects, but they were not analysed.
Each word-colour combination was presented eight times for a total of 144 trials (72 experimental and 72 fillers) divided into eight blocks of 18 trials each. The eight blocks were separated in two sequences of four blocks with a break between the two parts of the experiment.
Participants were tested individually in a quiet room of the hospital. They were required to name the ink colour in Catalan as soon and accurately as possible. Each experimental session started with a practice block of ten trials. The words in the practice were not presented in the other blocks of the experiment.
Each trial was organised as follows: a fixation point mark appeared in the centre of the screen for 500 ms, then a word appeared on the screen for 3,000 ms. The experiment was controlled by the software DMDX (Forster & Forster, Reference Forster and Forster2003), which recorded the participants’ vocal responses.
2.3. Analysis
Naming latencies were analysed offline using Checkvocal (Protopapas, Reference Protopapas2007). Naming latencies exceeding the three standard deviations, from incorrect responses and fillers, were excluded from the analysis. Errors were classified as follows: ‘omission’ when the participant was unable to name the colour within the time limit; ‘semantic’ when they produced an incorrect colour name or a word that was semantically related to the target; ‘cross-language intrusion’ when they produced the correct colour name but in the incorrect language; ‘phonological’ when they deleted, substituted, or added phonemes to the correct word; and ‘unrelated’ when they produced a word with no relation, semantic or otherwise, to the target word.
First, naming latencies and accuracy were analysed into two separate repeated measures ANOVAs, with Group (healthy controls, MCI, AD, and PD) as a between-subjects factor and Condition (congruent, control, and incongruent) as a within-subjects factor.
Second, if the effects of facilitation or interference were modulated by differences in overall processing speed between groups, a one-way ANCOVA was conducted with the naming latencies of the control as a covariate. The facilitation effect was calculated as the difference in the naming latencies and accuracy between the control and the congruent conditions. The interference effect was calculated as the difference between the naming latencies and accuracy of the incongruent and the control conditions.
Finally, two separate stepwise regression analyses were performed on the facilitation and interference effects to investigate whether they were predicted by neuropsychological deficits and any bilingualism variable. The variables included as predictors were the scores of the neuropsychological tests described in Table 2 and the variables associated with bilingualism described in Table 1. The regression analyses were performed for all the participants.
The alpha value for significance was set at .05 and the size effects were reported as partial eta squared values (η2p). Tukey's correction was applied for post-doc analyses.
3. Results
3.1. Naming latencies and accuracy
Naming latencies
The analysis showed significant main effects of Group [F(3, 58) = 19.58, p < .001; η2p = .37] and Condition [F(2, 116) = 95.30, p < .001, η2p = .15]. The Group x Condition interaction was also statistically significant [F (6, 116) = 2.50, p = .03; η2p = 0.01].
Post-hoc analysis for the main effect of Group showed the following. The AD patients were overall slower to perform the task (1,396 ms) than healthy controls (958 ms, p < .001), MCI (1,219 ms, p = .01), and PD patients (987 ms, p < .001). The MCI patients were significantly slower than healthy controls and PD patients (p < .001). The PD patients were not significantly different from healthy controls (p = .67) (see Figure 1A and Table 3).
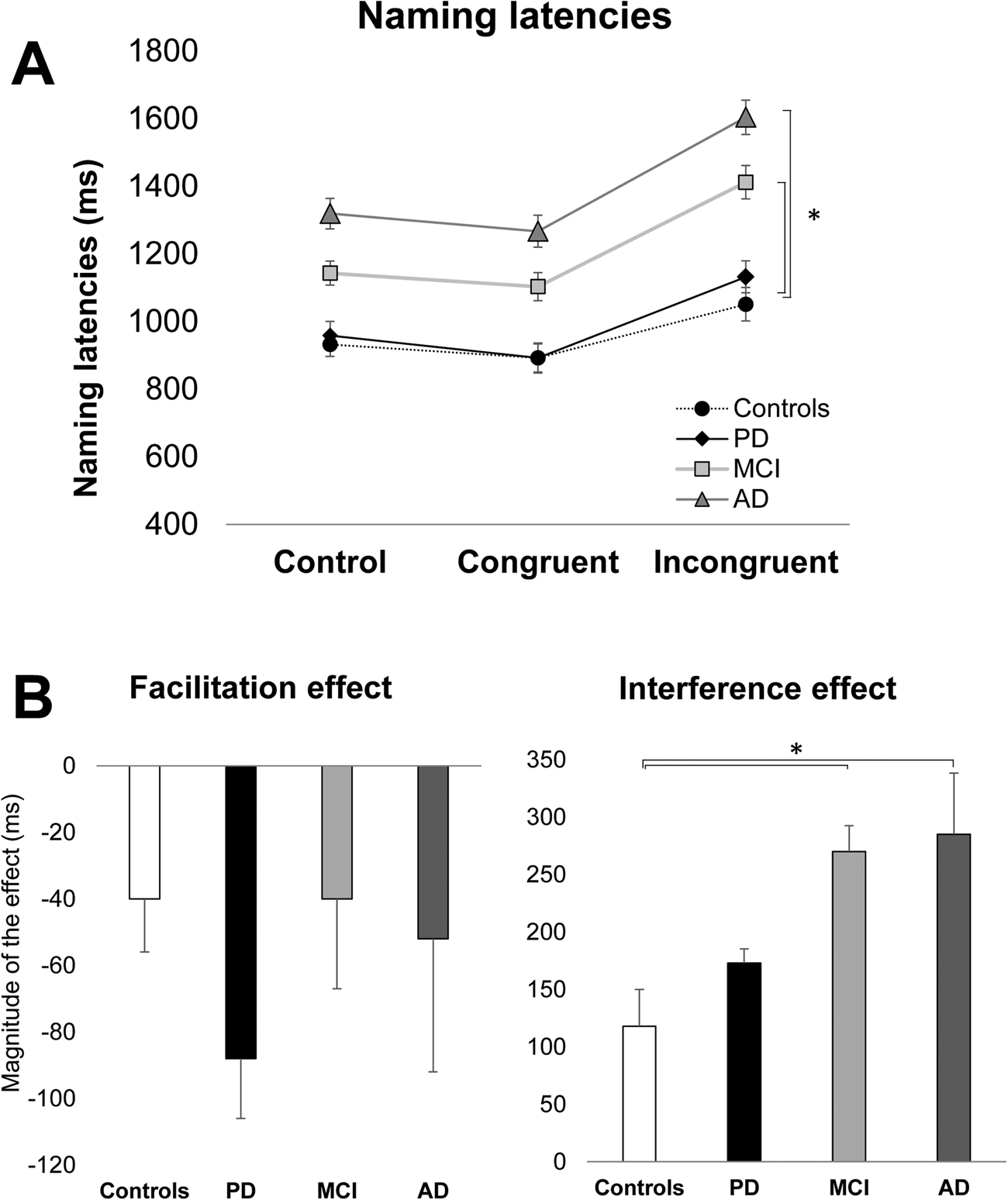
Figure 1 A. Naming latencies as a function of group (healthy adults, PD, MCI, and AD) and condition (control, congruent, and incongruent). B. Magnitude of facilitation and interference as a function of group (older adults, MCI, and AD). Bars display standard errors.
Table 3. Naming latencies and accuracy (%) in the cross-language Stroop task.
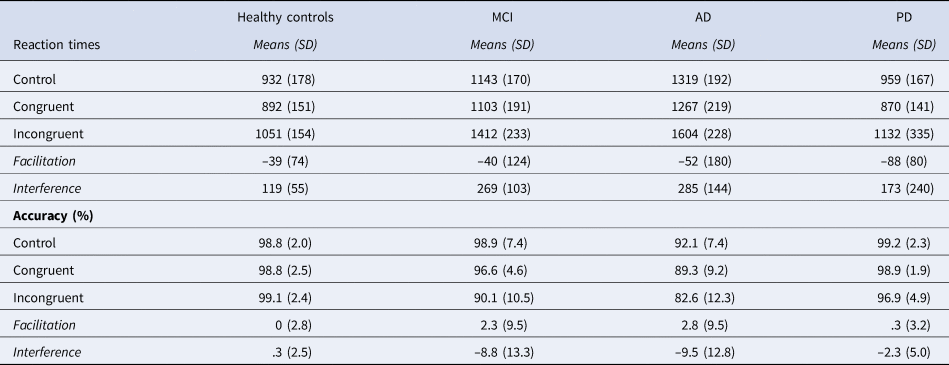
The post-hoc analysis for the main effect of Condition showed the following. The congruent condition (1,033 ms) was significantly faster than the control condition (1,088 ms, p < .001) and the incongruent condition (1,300 ms, p < .001) was significantly slower than the control condition.
To explore the Group x Condition interaction, two one-way ANOVAs were performed on the magnitude of the interference and facilitation effects.
The analysis for the interference effect showed a significant main effect of Group [F (3, 58) = 3.98, p = .01; η2p = .17]. The two patient groups that were significantly different from healthy controls (119 ms) were MCI (269 ms, p = .04) and AD (285 ms, p = .02). The main effect of the group was statistically significant when the naming latencies of the control condition were entered into the analysis as a covariate [F (3, 58) = 3.79, p = .01; η2p = .16]. In the post-hoc analysis, the MCI and AD patients were significantly different from the healthy controls (ps < .05), but not from PD patients (see Figure 1B).
The analysis of the facilitation effect showed a non-significant effect of Group [F (2, 58) = 0.53, p = 0.66].
Accuracy
The analysis of accuracy revealed significant main effects for Group [F (2, 58) = 20.19, p < .001; η2p = .27] and Condition [F (2, 116) = 11.15, p < .001; η2p = .07]. The Group x Condition interaction was also significant [F (6, 116) = 2.44, p = .03; η2p =.04] (see Figure 2A and Table 3).
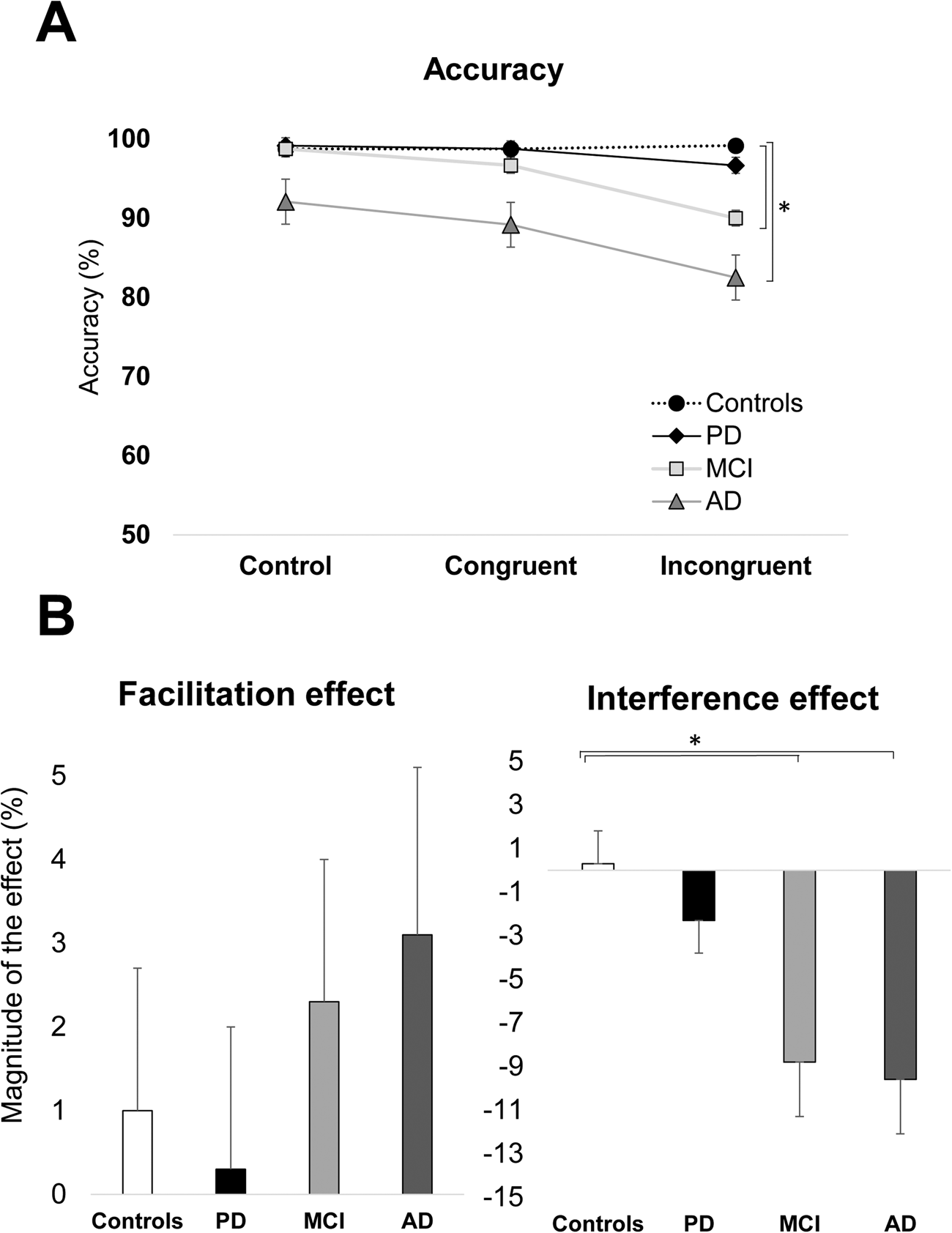
Figure 2 A. Accuracy (%) as a function of group (healthy adults, PD, MCI, and AD) and condition (control, congruent, and incongruent). B. Magnitude of facilitation and interference as a function of group (older adults, MCI, and AD). Bars display standard errors.
Post-hoc analysis for the main effect of Group showed the following. The AD patients performed significantly poorer (88%) than the healthy controls (98.9%, p < .001), MCI patients (95.0%, p < .001), and PD patients (98.3% p < .001). The MCI and PD patients performed the same as the healthy controls (ps > .05).
Post-hoc analysis for the main effect of Condition showed that the accuracy for the incongruent condition (92.1%) was significantly lower than the control condition (97.1%, p < .001) and the congruent condition (95.4%, p < .001). The congruent condition was not significantly different from the control condition (p = .22).
To explore the Group x Condition, one-way ANOVAs were performed on the magnitude of the interference and facilitation effects.
The analysis for the interference effect showed a significant main effect of Group [F (3, 58) = 3.74, p = .01; η2p = .17]. In the post-hoc analysis, both AD (−9.5%, p = .01) and MCI patients (-8.8%, p = .04) were significantly different from healthy controls (.03%), but not from PD patients (see Figure 2B).
The analysis of the facilitation effect showed a non-significant effect of Group [F (2, 58) = 0.67, p = 0.56].
In the AD group, 52.3% of total errors were omissions, 17.4% were cross-language intrusions, and 30.0% were semantic errors. In the MCI group, 41.2% of total errors were omissions, 16.5% were cross-language intrusions, and 42.3% were semantic intrusions.
3.2. Regression analysis
The correlation between the facilitatory and interference effects was not significant [r(61) = .03 p = .81, Spearman's rho r(61) = .04, p = .78].
For interference, the regression model that significantly predicted the magnitude of the effect [F (1, 27) = 8.63, p < .001, R2 = .36] only included the PPT score (B = - .41, p = .02).
For facilitation, the regression model that significantly predicted the magnitude of the effect [F (1, 27) = 6.57, p = .02, R2 = .20] only included the PPT score (B = .45, p = .02).
For both interference and facilitation, the variables associated with bilingualism were not significant predictors of the magnitude of the effects.
4. Discussion
The aim of the present study was to investigate the language control of bilingual patients with AD, MCI, and PD. Specifically, the study focused on cross-language interference by using a bilingual version of the Stroop task that had previously been used in bilingual young adults (Costa et al., Reference Costa, Albareda and Santesteban2008) and in patients with HD (Calabria et al., Reference Calabria, Pérez Pérez, Martínez-Horta, Horta-Barba, Carceller, Kulisevsky and Costa2021). The main interest of the study was the comparison between groups, especially AD and MCI to PD.
First, the main result for interference is that in comparison to each other the patient groups behaved differently and also in comparison to healthy groups. Healthy controls showed a significant cross-language interference, and its magnitude was larger than that found in young bilingual adults (88 ms) reported by Costa et al. (Reference Costa, Albareda and Santesteban2008). This was expected, since it is known that aging reduces the efficiency of the control processes in many tasks (Jeffrey & Jacoby, Reference Jeffrey, Jacoby, Bialystok and Craik2006), including the Stroop task (Bugg et al., Reference Bugg, DeLosh, Davalos and Davis2007).
PD patients performed similarly to healthy controls, as they were not significantly different from them for their processing speed and the magnitude of the interference effect. These results replicate those found in our previous study with HD patients (Calabria et al., Reference Calabria, Pérez Pérez, Martínez-Horta, Horta-Barba, Carceller, Kulisevsky and Costa2021). Interestingly, these findings support the dissociation between language engagement/disengagement (language switching) and cross-language interference suppression (Stroop task). In both HD (Calabria et al., Reference Calabria, Pérez Pérez, Martínez-Horta, Horta-Barba, Carceller, Kulisevsky and Costa2021) and PD patients (Cattaneo et al., Reference Cattaneo, Calabria, Marne, Gironell, Abutalebi and Costa2015, Reference Cattaneo, Costa, Gironell and Calabria2020) we consistently found that they were impaired in language engagement and disengagement while they performed a language switching task. Nevertheless, previous findings in HD patients (Calabria et al., Reference Calabria, Pérez Pérez, Martínez-Horta, Horta-Barba, Carceller, Kulisevsky and Costa2021) and those from the present study show that they are not impaired when they must deal with cross-language interference as they did not show larger Stroop effects in comparison to healthy controls. Therefore, the conclusion is that these two control processes are dissociated, and they play different roles in language control in dual-language contexts. These results support the view that basal ganglia are crucially involved in switching between languages (Abutalebi & Green, Reference Abutalebi and Green2008) and not in suppressing the language not in use.
The MCI and AD patients showed a significantly larger effect of interference as compared to the healthy controls. This larger interference effect in these two groups of patients was not explained by the slowing of their processing speed. Indeed, once the processing speed was controlled in the analysis, the effect was still significant between patients and healthy controls. This means that MCI and AD patients take longer when two competing pieces of information are to be processed and one has to be suppressed. In the Stroop condition, when the Spanish word ROJO (red) was presented in yellow ink it competed with the word to be produced – ‘groc’ (yellow in Catalan) – because of their high semantic relatedness. To correctly produce the word ‘groc’, the other competitors, in Catalan but also in Spanish, have to be suppressed. Therefore, both MCI and AD patients needed some extra time to solve the conflict.
Similarly, the results for accuracy suggest that MCI and AD patients were more prone to errors in the incongruent condition than healthy controls, meaning that they cannot resolve the conflict. In the incongruent condition of the Stroop task there are two conflicts to resolve, such as one between the colour words that do not match with their ink colour, and one between the two languages (word in Spanish and naming in Catalan). Branzi et al. (Reference Branzi, Della Rosa, Canini, Costa and Abutalebi2016) showed that the resolution of the conflict given by incongruent information in bilingual speech production could be associated with the cortical activation of prefrontal and inferior parietal areas. These authors proposed that these brain areas are engaged in language selection during bilingual speech production. Parietal areas, along with the entorhinal cortex and hippocampus, are functionally and structurally affected by MCI and in the early stages of AD (e.g., Talwar et al., Reference Talwar, Kushwaha, Chaturvedi and Mahajan2021). Therefore, the behavioural results from MCI and AD patients in the Stroop task are potentially compatible with the neuropathological alterations of the parietal areas. Additionally, AD patients were also more prone to errors than healthy controls and MCI in all conditions, suggesting that they could be also impaired in monitoring the information across all trials.
Abutalebi and Green (Reference Abutalebi and Green2007, Reference Abutalebi and Green2008) proposed that the parietal areas are involved in language control by supporting disengagement from the language not in use and recruited for assessing conflict choices in attentional demanding tasks in assessing conflict choices. In their most recent model (Abutalebi & Green, Reference Abutalebi and Green2016), they proposed that the parietal lobes are connected to the frontal inferior areas in managing the control of the two languages for response selection. Therefore, the damage over the posterior brain areas in MCI and AD patients would be the potential neural substrate for the conflict resolution deficits found in our patients while they performed the Stroop task.
The larger Stroop effects in MCI and AD patients, as compared to healthy controls, are consistent with previous findings in monolinguals (Bélanger et al., Reference Bélanger, Belleville and Gauthier2010; Bondi et al., Reference Bondi, Serody, Chan, Eberson-Shumate, Delis, Hansen and Salmon2002; Girelli et al., Reference Girelli, Sandrini, Cappa and Butterworth2001; Spieler et al., Reference Spieler, Balota and Faust1996; Tse et al., Reference Tse, Balota, Yap, Duchek and McCabe2010). The only difference is that in our study the Stroop effect was found when the conflict comes from two different languages. Since the written input (in Spanish) was orthographically different from the verbal response (in Catalan), the semantic interference might be explained as a cross-linguistic effect.
Faust and Balota (Reference Faust, Balota, Gorfein and MacLeod2008) proposed the attentional control framework to explain the resolution of the conflict that occurs in the Stroop task. In their model, two different pathways are activated (one appropriate and one inappropriate) from the stimulus set and the attention control system detects the conflict. To solve the conflict, the attentional control system must be effective to regulate the relative activation of the two pathways. Hence, in the Stroop task, there is a pathway of activation of the name of the ink colour and the other pathway for the written word that must be inhibited. If the attentional control system is effective, the conflict is solved, but when it is less efficient or impaired, the interference will be larger. This is what happens in MCI and AD patients, but not in PD patients.
Second, the cross-language facilitation was reliable in all groups, and it was not different in magnitude between patients and controls. In this condition, participants were required to name the colour of the ink of the word that was the translation equivalent in Catalan (e.g., the word AMARILLO, yellow in Spanish, when the response was ‘groc’, yellow in Catalan). Young adult bilinguals are about 100 ms faster compared to the control condition, as reported by Costa et al. (Reference Costa, Albareda and Santesteban2008). In the present study, we found that older adults were faster compared to the control condition (40 ms), but not as fast as young adults. Patients showed the same facilitation effect as older adults, suggesting that the benefits of converging information between the two languages is explained as an aging effect and not by neurodegeneration.
Monolingual AD patients also show facilitation in the congruent condition of the Stroop task (Faust & Balota, Reference Faust, Balota, Gorfein and MacLeod2008) when the distractor word is the same as the colour to be named. In the present study, the written word was in the non-dominant language, so the facilitation required the activation of the non-selected language by the task. The fact that patients do show facilitation means that they have access to their non-dominant language as they do for their dominant one. The activation of their non-dominant language may be an automatic process from converging information (colour and word) that is spared in patients with subcortical and cortical degenerative diseases. This is in line with previous studies on the integrity of automatic and controlled processes in patients with AD. The common finding is that, in language tasks that require automatic lexical access (e.g., semantic priming), AD patients show the same facilitation as healthy controls. Conversely, AD patients are impaired from the earlier stage of the disease in tasks that involve controlled processes, such as those that require more attentional resources and flexibility (Adam et al., Reference Adam, Collette, Van Der Linden, Lemauvais and Salmon2005; Duong et al., Reference Duong, Whitehead, Hanratty and Chertkow2006). In the Stroop condition, the activation of the non-dominant language is supposed to be automatic, but not for controlled processes, such as for the interference suppression that is needed to reduce the semantic interference from the conflict between the colour and the word.
According to the converging information hypothesis, both facilitation and interference could be explained by the same mechanisms (Cohen et al., Reference Cohen, Dunbar and McClelland1990; Melara & Algom, Reference Melara and Algom2003; Roelofs, Reference Roelofs2010). Roelofs (Reference Roelofs2010) found both effects in Dutch–English speakers and argued that these results are compatible with the hypothesis that the locus of interference and facilitation is the same, and the difference rests on the degree of convergence of the information. Nevertheless, two results from this study are not completely compatible with the view of a single locus of these two processes. First, the magnitudes of effects for interference and facilitation were uncorrelated, suggesting that they are not completely dependent on each other. If they were, we should expect a certain degree of correlation between them. Second, interference was found to be increased in AD and MCI but not in PD patients, whereas facilitation was the same across all groups. Therefore, these results are probably more compatible with the hypothesis that facilitation is the result of the integrity of the automatic processes and interference of the controlled processes. In bilingual individuals, this extends to the simultaneous activation of the two languages (Costa et al., Reference Costa, La Heij and Navarrete2006; Finkbeiner et al., Reference Finkbeiner, Almeida, Janssen and Caramazza2006a, Reference Finkbeiner, Gollan and Caramazza2006b).
Finally, facilitation and interference were only predicted by the PPT score. In order to find an explanation of such an effect in terms of cognitive deficits, all the neuropsychological scores were introduced into the regression analysis. The result indicated that only the semantic association task was predicting both task effects, but in the opposite direction. The PPT scores were negatively associated with interference, indicating that larger effects were correlated with poor performance in the semantic task. Conversely, PPT was positively associated with facilitation. These results seem to suggest that some semantic processing is required for the execution of the Stroop task and perhaps those are more related to semantic control. In our study, only AD patients showed significantly poorer performance in the PPT as compared to controls, suggesting the presence of semantic deficits in this patient group. Arguably, the relationship between facilitation, interferences and PPT scores is mediated by the presence of semantic processing deficits.
In conclusion, we found that the cross-language interference, as a measure of language control in bilingual speakers, is differentially affected by the type of neurodegeneration. Indeed, interference suppression was found to be impaired in more cortical types of neurodegeneration (MCI and AD), but unimpaired in subcortical types such as PD. These findings have implications for the neural models of bilingual language control and the nature of the relationship between interference suppression and other control processes, such as those involved in language switching.
Acknowledgements
The author thanks Paula Marne for helping in data collection and all the participants who gave their time generously.
This research was supported with funding from Agencia Estatal de Investigación (AEI, National Research Agency) and Fondo Europeo de Desarrollo Regional (FEDER, European Regional Development Fund) under the project RED2018-102615-T.