Introduction
The creation or restoration of compensatory habitat to mitigate biodiversity losses is a popular conservation strategy as a response to development pressures. Achieving effective outcomes requires an understanding not only of whether compensatory habitat is superficially similar to occupied habitats, but whether it will lead to the establishment and persistence of the target species (Armstrong & Seddon, Reference Armstrong and Seddon2008). However, the effectiveness of management plans is generally not well established and is limited in scope as a result of resource limitations (Armstrong & Seddon, Reference Armstrong and Seddon2008), ecological time lags (Morris et al., Reference Morris, Alonso, Jefferson and Kirby2006), insufficient monitoring (Edgar et al., Reference Edgar, Griffiths and Foster2005), and uncertainty of targets and outcomes (Maron et al., Reference Maron, Hobbs, Moilanen, Matthews, Christie and Gardner2012; Germano et al., Reference Germano, Field, Griffiths, Clulow, Foster, Harding and Swaisgood2015). Efforts to mitigate biodiversity losses are also often unsuccessful because conservation management decisions are not always based on scientific evidence (Pullin et al., Reference Pullin, Knight, Stone and Charman2004; Sutherland et al., Reference Sutherland, Pullin, Dolman and Knight2004; Stewart et al., Reference Stewart, Coles and Pullin2005).
Adaptive management is an evidence-based approach that combines conservation management and monitoring to reduce uncertainties, minimize risk and improve decision making (Keith et al., Reference Keith, Martin, McDonald-Madden and Walters2011; McCarthy et al., Reference McCarthy, Armstrong, Runge, Ewen, Armstrong, Parker and Seddon2012; Canessa et al., Reference Canessa, Guillera-Arroita, Lahoz-Monfort, Southwell, Armstrong and Chadès2016). This approach has been used in conservation management to compare alternative mitigation strategies and predict outcomes (Lyons et al., Reference Lyons, Runge, Laskowski and Kendall2008; Runge et al., Reference Runge, Converse and Lyons2011; McCarthy et al., Reference McCarthy, Armstrong, Runge, Ewen, Armstrong, Parker and Seddon2012; Moore et al., Reference Moore, Converse, Folk, Runge and Nesbitt2012). Greater efficiency and success rates in conservation could also be achieved with experimental trials. However, experimental designs are usually not feasible and are rarely used in conservation, as they require replication or large sample sizes (Seddon et al., Reference Seddon, Armstrong and Maloney2007; Armstrong & Seddon, Reference Armstrong and Seddon2008; Sheean et al., Reference Sheean, Manning and Lindenmayer2012). Nevertheless, a number of experimental trials have been conducted to improve decision making for reintroductions (Pedrono & Sarovy, Reference Pedrono and Sarovy2000; Hu & Jiang, Reference Hu and Jiang2002; Moseby & Bice, Reference Moseby and Bice2004; Kemp et al., Reference Kemp, Norbury, Groenewegen, Comer, Armstrong, Hayward, Moro and Seddon2015), as well as habitat creation and restoration projects (Nicol et al., Reference Nicol, Lieschke, Lyon and Koehn2004; Molony & Bird, Reference Molony and Bird2005). A trial may also be valuable prior to a large-scale implementation of compensatory habitat. The results could establish whether the management project was likely to achieve the desired results, and identify potential limiting factors that may affect establishment and persistence of the target species (Seddon et al., Reference Seddon, Armstrong and Maloney2007; Armstrong & Seddon, Reference Armstrong and Seddon2008; Kemp et al., Reference Kemp, Norbury, Groenewegen, Comer, Armstrong, Hayward, Moro and Seddon2015). However, we could find no published study in which an experimental trial was undertaken prior to the creation of a compensatory habitat offset.
Challenges in conservation management plans are evident for the green and golden bell frog Litoria aurea. This Australian species has undergone declines driven by disease and habitat disturbance since the 1970s (Mahony et al., Reference Mahony, Hamer, Pickett, McKenzie, Stockwell and Garnham2013), and is categorized as Vulnerable on the IUCN Red List (Hero et al., Reference Hero, Gillespie, Cogger, Lemckert and Robertson2004). As a result of its frequency of occurrence in areas of high development activity, L. aurea has been the subject of the largest number of management proposals of any Australian amphibian (White & Pyke, Reference White and Pyke2008a). Untypically for a threatened species, it is a colonizing species found in a broad range of environments, exhibits rapid growth and is highly fecund (Mahony et al., Reference Mahony, Hamer, Pickett, McKenzie, Stockwell and Garnham2013), and should represent a model candidate for successful conservation outcomes. However, most management plans have been unsuccessful in producing self-sustaining populations, with failures attributed to habitat quality, inadequate number of individuals released, predators or disease (Daly et al., Reference Daly, Johnson, Malolakis, Hyatt and Pietsch2008; Pyke et al., Reference Pyke, Rowley, Shoulder and White2008; Stockwell et al., Reference Stockwell, Clulow, Clulow and Mahony2008; White & Pyke, Reference White and Pyke2008a), although the lack of experimental methodology reported and transparency of outcomes in many of these management plans prevents confidence in causal inference. The only programme thus far to have produced a persisting population successfully in managed habitat was one with substantial financial backing, in Sydney Olympic Park, which had a large extant population (O'Meara & Darcovich, Reference O'Meara and Darcovich2015).
In this study we constructed an experimental habitat plot for L. aurea, to inform a broader compensatory habitat offset programme. Within the trial plot, half of the waterbodies were enclosed within an exclusion fence to separate the effects of habitat suitability from biological processes such as interspecific competition, predation, dispersal and disease. A natural wetland near the trial plot was also included for comparison. We released captive-bred tadpoles into both the trial plot and the nearby wetland, and monitored them on a weekly basis for 3 years. The aim was to determine whether L. aurea grows, persists and reproduces in created habitat. We compared growth rates between our trial plot population and a nearby naturally occurring extant population, and examined persistence and survival between age and sex classes over time, along with reproductive events. The presence of predators, competitors and disease were monitored, and habitat associations along with weather variables affecting detectability were identified to optimize resource allocation.
Study area
We conducted the study on Kooragang Island in New South Wales, Australia (Fig. 1), which currently supports one of the last remaining populations, and the largest extant population, of L. aurea in the region (Hamer & Mahony, Reference Hamer and Mahony2010). Kooragang is a heterogeneous estuarine island situated in the mouth of the Hunter River, with a history of extensive land clearing and hydrological alterations for agricultural and industrial use. The landscape is dominated by kikuyu grass Pennisetum clandestinum, salt marsh, mangroves, wetlands and cattle pasture. The north-west portion of the island has been reclaimed after extensive use as an agricultural zone, and is currently a nature reserve within the Hunter Wetlands National Park system. The south-east of the island is heavily industrialized and contains the coal loading facilities for the Port of Newcastle.
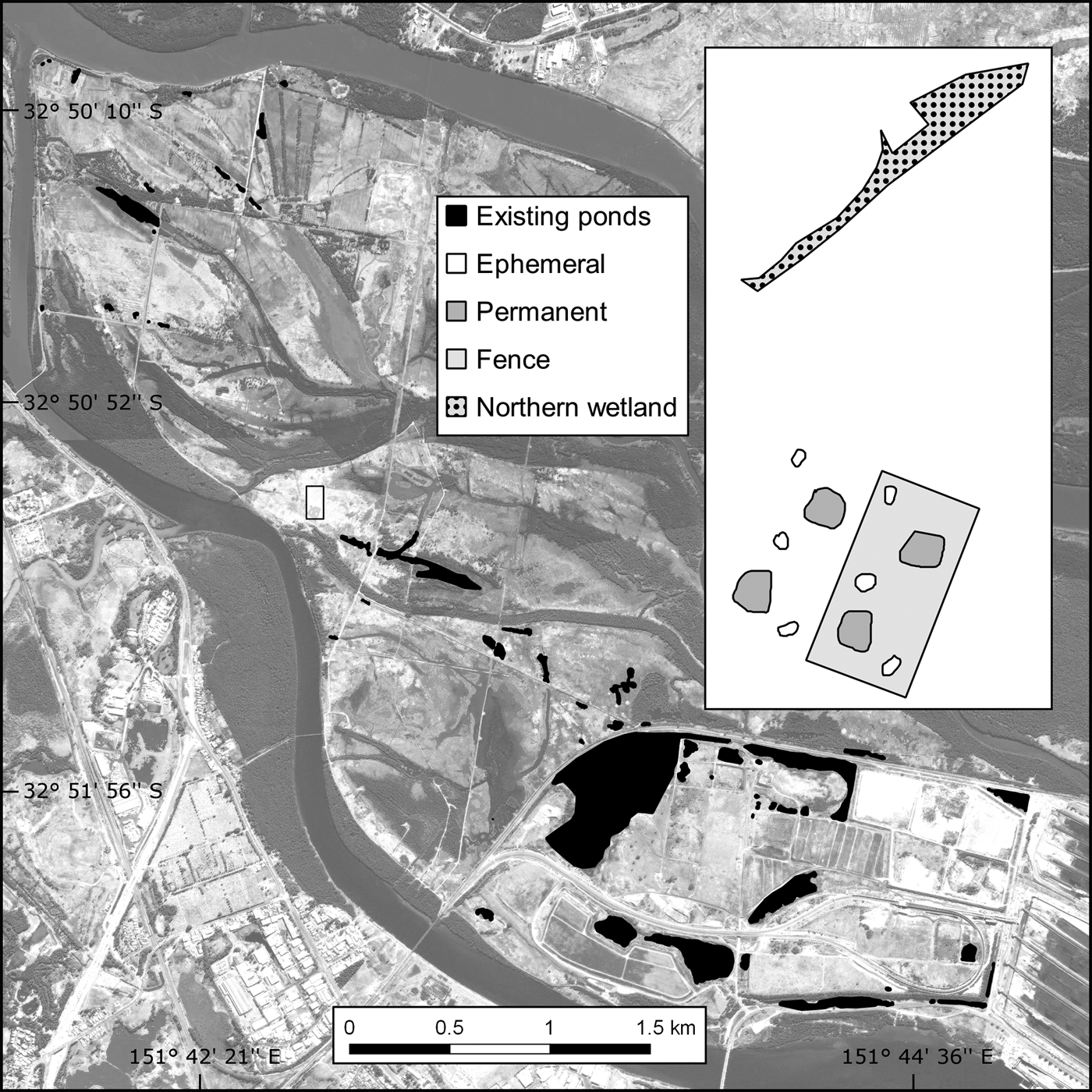
Fig. 1 The location of the study site and existing ponds on Kooragang Island, New South Wales, Australia.
Methods
Trial plot
The c. 2,675 m2 trial plot was constructed adjacent to (within 10 m of) the proposed location of a larger habitat offset programme. Although the area is within the historical distribution of L. aurea, pre-construction surveys found no evidence of the species at or near the trial plot. Shelter and foraging microhabitats were included as per the L. aurea habitat template of terrestrial and emergent vegetation, with artificial habitat such as rock piles, terracotta tiles, tins and hay bales (Pyke & White, Reference Pyke and White1996). Half of the trial plot was completely enclosed with a predator-proof mesh fence and bird net, and the plot had four permanent ponds (c. 60 m2) and six ephemeral ponds (c. 10 m2) across the fenced and unfenced portions (Fig. 1). To compare the released population in the trial plot with a naturally occurring habitat, the study also included a naturally occurring northern wetland (c. 270 m2) located 50 m north-west of the trial plot.
Captive breeding
Litoria aurea tadpoles were bred in captivity from individuals collected from a natural waterbody 1.75 km from the trial plot. We released tadpoles during two periods: March–July 2011 and December 2011–January 2012. Between March and July 2011, 608 bell frogs (the product of six breeding events) were released as tadpoles or recently metamorphosed juveniles; half into the fenced area and half into the unfenced area. Between December 2011 and January 2012, 9,708 bell frog tadpoles (the product of nine breeding events) were released. Tadpoles were distributed evenly between the permanent ponds to avoid the risk of ephemeral ponds drying before tadpoles could metamorphose. A total of 1,804 tadpoles were released inside the fenced area, 5,208 in the unfenced area, and 3,000 in the northern wetland. A greater number of individuals were released in the unfenced sites to compensate for those lost to predation and dispersal. All tadpoles were implanted with a visible implant elastomer, with unique tag colours for each of the two halves of the trial plot and the northern wetland so we could identify trial plot individuals from the extant population and determine movement (Bainbridge et al., Reference Bainbridge, Stockwell, Valdez, Klop-Toker, Clulow, Clulow and Mahony2015). The chytrid fungus, Batrachochytrium dendrobatidis, was not detected prior to release.
Field methods
The trial plot was monitored weekly for 3 years, during April 2011–April 2014. Standardized auditory surveys (Scott & Woodward, Reference Scott, Woodward, Heyer, Donnelly, McDiarmid, Hayek and Foster1994) were used to identify the location and number of calling L. aurea and other amphibian species. Standardized visual encounter surveys were then conducted at each pond and throughout the surrounding terrestrial area (Crump & Scott, Reference Crump, Scott, Heyer, Donnelly, McDiarmid, Hayek and Foster1994). During visual encounter surveys all habitat structures were inspected and any L. aurea encountered were captured by hand in a thin plastic bag. We recorded the presence of L. aurea, terrestrial invertebrates and any other animal species found.
After visual encounter surveys were completed, we measured the snout-to-vent length and body weight of every L. aurea captured. We determined the sex and age class, based on secondary sexual characteristics. Individuals with a snout-to-vent length of < 45 mm were recorded as juveniles; those longer than 45 mm were recorded as males if nuptial pads were present, and as females if nuptial pads were absent. Sexual maturity and reproductive readiness in males were determined based on the colour of the nuptial pads, which are dark brown or black in sexually mature males during the breeding season and fade to off-white or pink during the remainder of the year (Pyke & White, Reference Pyke and White2001). We determined whether females were gravid by placing them on their dorsal surface over a light source and examining their abdominal cavity for the presence of eggs. Newly captured individuals > 35 mm were implanted with passive integrated transponder tags injected subcutaneously into the dorsal lymph space (Tropek et al., Reference Tropek, Kadlec, Karesova, Spitzer, Kocarek and Malenovsky2010). All captured individuals were scanned with a Trovan LID-560ISO (Trovan Ltd, UK) to identify previously tagged individuals, and the presence of visible implant elastomer tags was recorded. For captured individuals without visible implant elastomer or passive integrated transponder tags, a tissue biopsy was taken to determine genetics, if necessary (Supplementary Material). To detect the presence of the chytrid fungus, individuals were swabbed in a standardized manner on their ventral surface using fine-tipped sterile swabs.
Dip-netting surveys were also conducted to determine the presence and relative abundance of macroinvertebrates, fish and tadpoles in the ponds. Water quality parameters such as temperature, salinity and pH were measured in each pond using a hand-held YSI Professional Plus (Xylem, USA) water quality probe. A Kestrel 3500 weather meter (Nielsen-Kellerman, USA) was used to measure air temperature, relative humidity and wind speed, and percentage cloud cover was estimated visually. As periods of heavy rainfall and flooding events initiate L. aurea activity and reproduction (Hamer et al., Reference Hamer, Lane and Mahony2008), the amount of rainfall in the previous 24 hours and in the 30 days prior to each survey was determined based on records from a weather station at Newcastle University (Station 061390), 4 km away (Bureau of Meteorology, 2016). To compare individual body sizes and reproductive activities of our captive-bred population with free-living populations in the natural landscape, we also conducted visual encounter surveys across 58 existing ponds on Kooragang Island each year during the breeding seasons (pond sizes: min. = 31 m2, max. = 250,332 m2, mean = 8,094 m2).
Statistical analysis
A linear regression analysis was conducted to determine differences between growth rates in the trial plot and the extant Kooragang Island population. The relationship between snout-to-vent length and the cube root of weight of captured individuals was examined. Growth rates were compared by estimating the regression slope coefficient of the interaction between the sites and snout-to-vent length (mm), with cube root of weight (g) as the response variable. To compare reproductive trends with the Kooragang Island population, χ2 tests were used to determine whether the number of calling males and the number of reproductive events differed between the three breeding seasons across Kooragang Island. χ2 tests were also used to determine differences between the fenced and unfenced areas by comparing the total numbers of competitor and predator species found.
Apparent survival (ϕ) and recapture (p) probabilities were estimated using Cormack–Jolly–Seber population models in MARK v. 8.0 (White & Burnham, Reference White and Burnham1999). Models were compared with combinations of constant (.) and time (year), and as a grouped function of site (site). Akaike information criterion corrected for small sample sizes (AICc) was used to assess the best supported model (Burnham & Anderson, Reference Burnham and Anderson2002). All candidate models were ranked by AICc, and those with ΔAICc of < 2 were considered to be the best of the model set (Burnham & Anderson, Reference Burnham and Anderson2002). Akaike weights were calculated to quantify the relative strength of evidence in support of a model given the data available.
To determine the factors affecting the relative weekly abundance of L. aurea, we used generalized linear models with the package pscl in R v. 3.3.2 (R Development Core Team, 2016). The data were overdispersed, and therefore a negative binomial regression model was used. Relative abundance between the sites was tested by comparing differences between the sites over time, accounting for the total number of tadpoles released. Habitat parameters assumed to be representative of habitat quality were included, such as water quality (salinity, pH, water temperature), habitat type (ephemeral pond, permanent pond, terrestrial) and species diversity (amphibians, tadpoles, invertebrate taxa). Climatic variables that could alter L. aurea activity patterns (e.g. season, relative humidity, wind speed, cloud cover, air temperature, and rainfall amount in the previous 24 hours and 30 days) were also investigated to determine the best conditions for optimizing future survey efforts. Habitat and climate covariates were added individually to the base model and retained if it improved the AICc. The lowest AIC scores and highest Akaike weights were used to select the most parsimonious model.
Results
Growth
We found a significant relationship between snout-to-vent length and the cube root of weight for all L. aurea individuals captured (t = 129.78, P < 0.001) (Fig. 2). No significant difference was observed between the body sizes of the trial plot population and the extant Kooragang Island population (t = 1.80, P = 0.0713), and no interaction between body size and the two sites (trial plot and Kooragang Island) was found (t = 1.83, P = 0.0669).
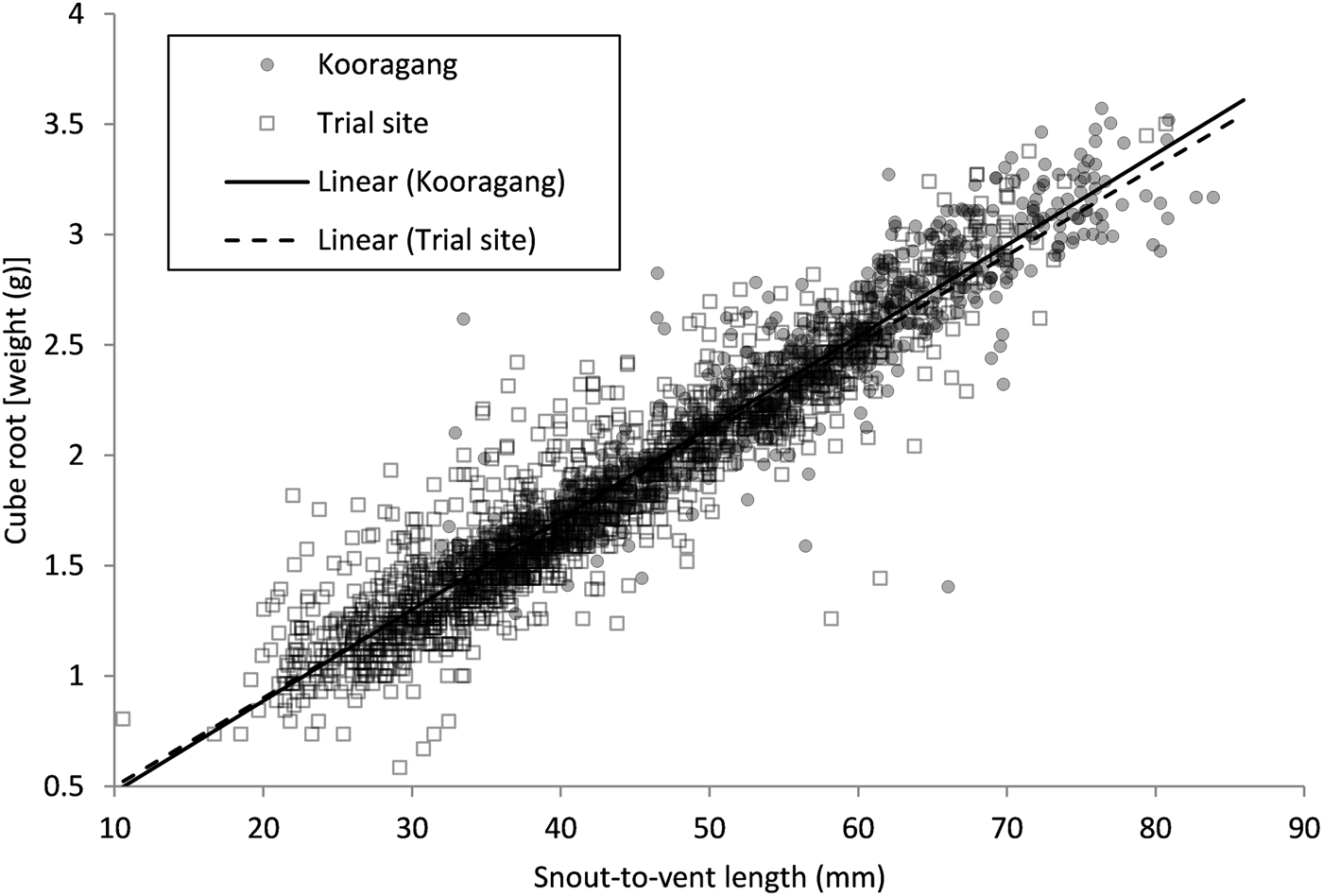
Fig. 2 The cube root of body weight relative to snout-to-vent length of Litoria aurea individuals captured from the trial plot population and the extant Kooragang Island population.
Reproduction
Males reached reproductive maturity within the first year following release, as indicated by calling and darkly pigmented nuptial pads, mostly in the fenced area (Fig. 3). Most males were heard calling (87%) during the first year, with greater numbers in the fenced area (Fig. 3a). The number of males with darkened nuptial pads increased every year, with 62% overall found in the unfenced area (Fig. 3b). No gravid females were found during the first year but were found during the second year and increased in number in the third year (Fig. 3c). Gravid females were found in the northern wetland only during the second year, in the fenced area only in the third year, and in the unfenced area during both the second and third years (Fig. 3c). Across the extant population surveyed on Kooragang Island, no difference was found in the total number of calling males between the two areas (trial site and Kooragang Island) (χ 2 = 3.768, df = 2, P = 0.15) or between the three breeding seasons (n = 32, 47, 33, respectively). Of the 58 extant ponds, reproduction occurred in five ponds during both 2011 and 2012, and in three ponds in 2013, with no difference found between the years (χ 2 = 6.15, df = 2, P = 0.74). Despite the presence of reproductively mature adults in the second and third years after release, no L. aurea tadpoles or metamorphosed individuals were found in the trial plot or northern wetland. However, juveniles that were too young to have been part of the release programme and did not possess visible implant elastomer tags were found in the unfenced portion of the trial plot in the second year. Genetic analyses indicated a high likelihood that breeding between released and wild individuals occurred near the trial plot (Supplementary Material).

Fig. 3 Yearly L. aurea count totals from weekly surveys of the fenced and unfenced areas of the trial plot, and a nearby northern wetland, on Kooragang Island (Fig. 1) during April 2011–April 2014: (a) calling males; (b) males with dark nuptial pads; and (c) gravid females.
Competitors and predators
The occurrence of other amphibian species differed between the fenced and unfenced areas, with 78 individuals found in the unfenced area, and 14 in the fenced area (χ 2 = 44.52, df = 1, P < 0.001). Potential predators, such as skinks, snakes and mice, were found under tins and terracotta tiles 246 times in the unfenced area and 220 times in the fenced area, with no difference found between the sites (χ 2 = 1.451, df = 1, P = 0.228). We found L. aurea under tins and terracotta tiles only eight times during the 3-year period.
Persistence
A total of 2,738 bell frogs were observed during the weekly visual encounter surveys, of which 568 were unique individuals identified by passive integrated transponder tags. Of these, 59.3% were juveniles, 15.56% were males and 25.14% females. Most tagged individuals encountered were found in the fenced area, which had a declining trend in encounters over the 3 years (Fig. 4a). We found L. aurea at lower relative densities in the unfenced area and the northern wetland, with an increase observed over the years in the unfenced area and an increase in the second year in the wetland (Fig. 4b,c). Whereas the number of juveniles in the fenced area decreased over time, the number of juveniles in the unfenced area and the wetland increased (Fig. 4a,b,c), probably as a result of the breeding event that occurred in the second year. Adult and juvenile frogs were recaptured 1–15 times throughout the study. Of those tagged, 57% were not recaptured. Of those that were recaptured, 38.82% were recaptured within the same year they were tagged. Although 10% of individuals were captured the year after being tagged, no individuals from the first year were recaptured in the last year. The fenced area had the highest percentage (9.26%) of recaptured tagged individuals, compared to the unfenced area (6.27%) and the wetland (3.67%).
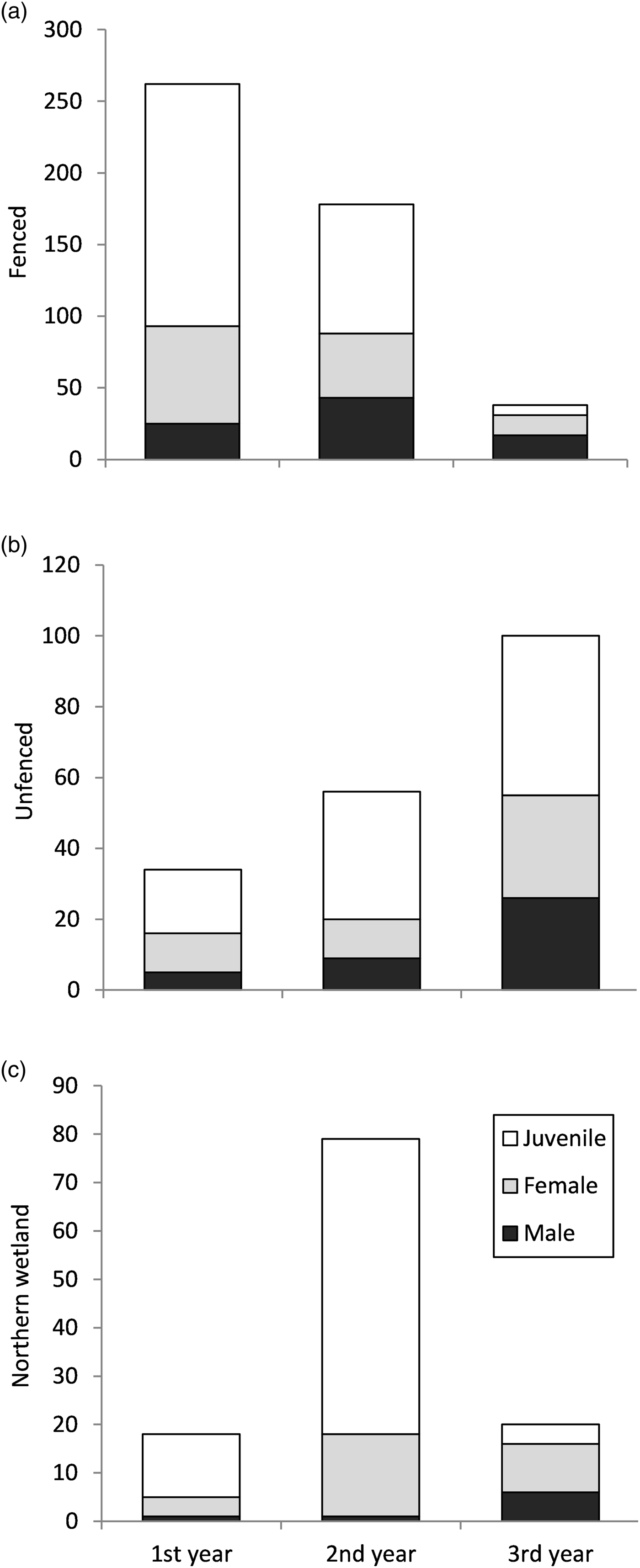
Fig. 4 Total numbers of tagged L. aurea individuals, separated by sex and age class, encountered during weekly monitoring of (a) the fenced and (b) unfenced areas of the trial plot, and (c) the nearby northern wetland, on Kooragang Island (Fig. 1) during April 2011–April 2014.
Apparent survival
The top supported model indicated survival was dependent on year, sex and site, whereas recapture was dependent on site (Table 1, Fig. 5). Adults had higher survival rates than juveniles, and survival decreased for all individuals in all areas over time (Fig. 5a). Survival rates were lowest in the northern wetland, which also had lower recapture rates compared to the trial plot areas (Fig. 5b).

Fig. 5 Parameter estimates and standard errors from the best model predicting (a) probability of survival and (b) recapture rate for L. aurea in the fenced and unfenced areas of the trial plot, and a nearby northern wetland, on Kooragang Island (Fig. 1).
Table 1 Top five model sets with covariates in apparent survival and relative abundance of Litoria aurea in the trial plot and northern wetland on Kooragang Island in New South Wales, Australia (Fig. 1). Models are ranked by ascending ΔAICc, used to estimate survival (φ) and recapture probability (p) modelled as constants (.) or as functions of year, site, and sex/age class.

*Covariates estimating relative abundance include season, number of tadpoles released (rel), invertebrate diversity (invertdiv), frog species diversity (frogdiv), tadpole diversity (taddiv), water temperature (wtemp), cloud cover (cc), and an interaction between site and area.
Relative abundance
The best-fitting model predicting weekly relative abundance (Table 1) included site of release over time (Fig. 6a), number of tadpoles released (Fig. 6b), season (Fig. 6c), water salinity (Fig. 6d), number of tadpoles (Fig. 6e), number of aquatic invertebrate taxa (Fig. 6f), water temperature (Fig. 6g), and wind speed (Fig. 6h). Collinearity was not found between significant covariates in the final model.
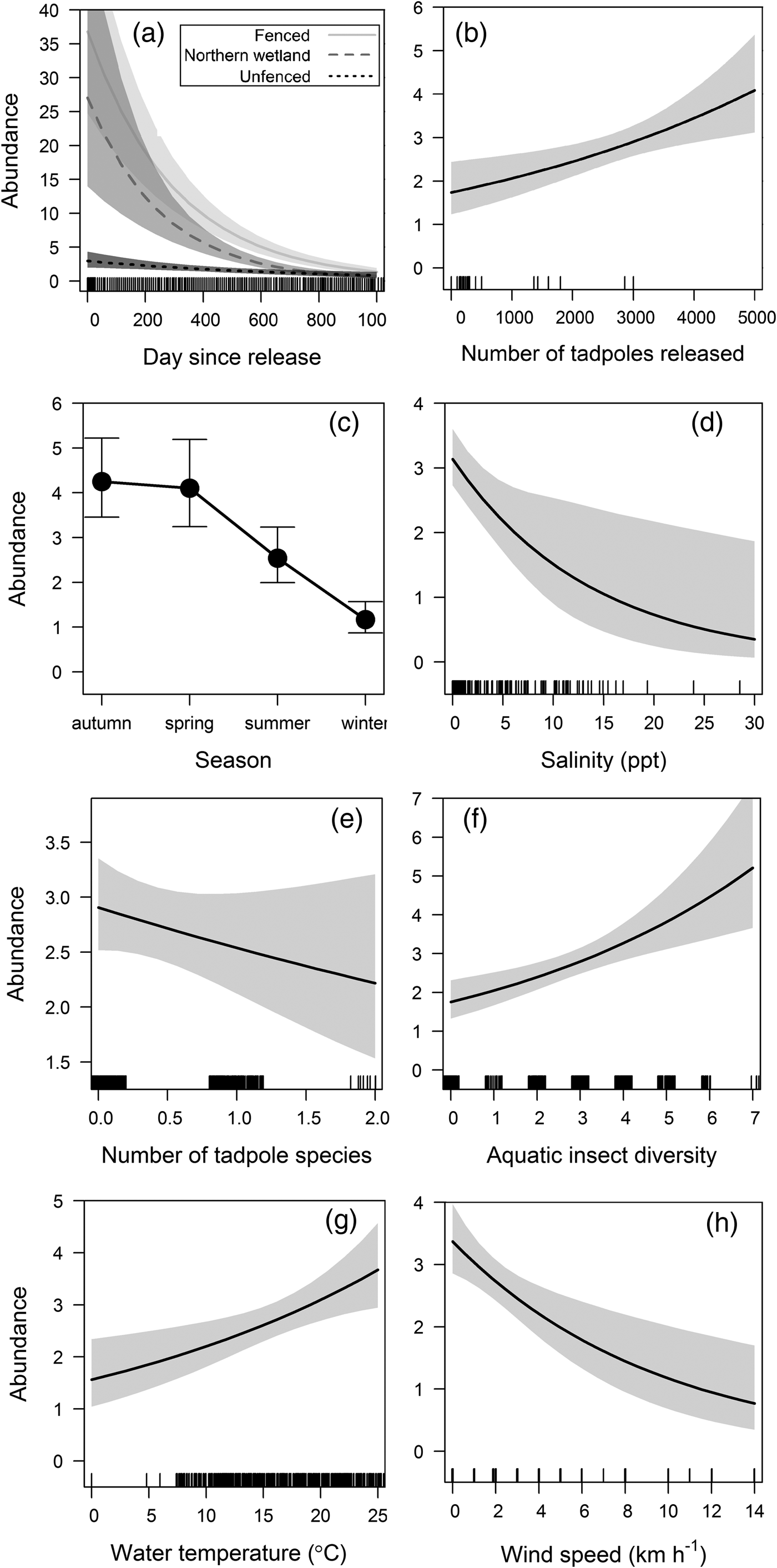
Fig. 6 Estimates of the best model predicting weekly relative abundance of L. aurea in the trial plot and northern wetland on Kooragang Island (Fig. 1), showing relationships between predicted abundance and (a) site of release over time, (b) number of tadpoles released, (c) season, (d) water salinity, (e) number of tadpole species, (f) number of aquatic invertebrate taxa, (g) water temperature, and (h) wind speed, with 95% confidence intervals.
Discussion
Our results indicate the trial plot provided L. aurea with sufficient resources to produce body sizes similar to those in the extant population and persist for a minimum of 3 years. Despite the presence of key threatening processes, which co-occur in the extant Kooragang Island population (Stockwell et al., Reference Stockwell, Bower, Bainbridge, Clulow and Mahony2015), the range of abiotic and biotic variables was suitable for growth and survival from the tadpole to the adult stage. Body sizes were similar to those in the extant population, suggesting there were sufficient resources. With the unfenced component of the study, we were also able to show that L. aurea occupied this habitat despite having the ability to disperse. However, although the population persisted for 3 years, it may not persist in the long term, as there was an overall decline in population and survival over time and no breeding occurred. As an r-selected species with high turnover, L. aurea requires consistently sufficient recruitment to compensate for low survival (Mahony et al., Reference Mahony, Hamer, Pickett, McKenzie, Stockwell and Garnham2013; Pickett et al., Reference Pickett, Stockwell, Bower, Pollard, Garnham, Clulow and Mahony2014). The high turnover rates were reinforced by our models, which indicated a negative relationship between abundance and the number of days since release, as a result of mortality and emigration over time. It is likely that the fenced site reached carrying capacity, whereas the unfenced area and the wetland were affected by interspecific competition and dispersal. Furthermore, a chytrid outbreak occurred in our population, with 15 mortalities in the trial plot, 12 in the fenced area, two in the unfenced area, and one in the wetland. The lack of dispersal and greater density within the fenced area may explain the greater mortality from chytrid.
Accounting for release numbers, L. aurea were more abundant and had a larger proportion of individuals in the fenced area. This suggests that the created habitat supported growth, but interspecific competition, predation, and dispersal reduced numbers in the unfenced area. Although we could not separate these effects, dispersal was probably a major factor in the low abundance and persistence in the unfenced sites. During visual-encounter surveys across Kooragang Island, tagged individuals from the unfenced area and the wetland were found in ponds up to 1 km away. Furthermore, a few individuals that were released inside the fence were later found outside. As a colonizing species, L. aurea commonly disperses over large distances (Hamer et al., Reference Hamer, Lane and Mahony2008; White & Pyke, Reference White and Pyke2008a), and despite being relatively small the species requires a large landscape because of its long-distance dispersal behaviour (Valdez et al., Reference Valdez, Stockwell, Klop-Toker, Clulow, Clulow and Mahony2015). Future management plans should therefore focus on landscape-level initiatives (Hamer & Mahony, Reference Hamer and Mahony2010; Pickett et al., Reference Pickett, Stockwell, Bower, Garnham, Pollard, Clulow and Mahony2013).
During winter at the end of the first year the aforementioned chytrid outbreak occurred in the trial plot, and some of the losses and the low rates of individual persistence can be attributed to this disease. These results are consistent with global chytrid infection dynamics, in which cold temperatures increase virulence because of lowered immune responses in amphibians (Berger et al., Reference Berger, Speare, Daszak, Green, Cunningham and Goggin1998; Carey & Alexander, Reference Carey and Alexander2003). Not only is chytrid thought to have been a driving force in the original decline of L. aurea (Mahony et al., Reference Mahony, Hamer, Pickett, McKenzie, Stockwell and Garnham2013), it has also been implicated in the failure of a number of management programmes for the species (Stockwell et al., Reference Stockwell, Clulow, Clulow and Mahony2008; White & Pyke, Reference White and Pyke2008a). However, despite the typical overwintering die-offs and a lack of recruitment, this population overcame the outbreak. Their persistence may be attributable to the large numbers of individuals released. Similarly, there was no significant decline in the large population in Sydney Olympic Park despite a chytrid outbreak (Penman et al., Reference Penman, Muir, Magarey and Burns2008). Compared with the wetland and the unfenced area, the highest number of chytrid-related deaths and the largest decline over the 3-year study period occurred in the fenced site. The high level of mortality within the fenced area is probably attributable to the hindrance to dispersal and the ease of detection of sick and moribund individuals. Furthermore, chytrid may spread quickly in a dense population in which individuals cannot avoid infected and deceased conspecifics.
An essential requirement for the persistence of a species within a landscape is reproduction. In this study we found no evidence of L. aurea breeding in the trial plot. By comparison, breeding occurred every year in the extant Kooragang Island population, indicating suitable climatic conditions. Although there was an increase in the number of males that reached maturity during the first and second years of the study, the number of calling males decreased after the second year. This was a trend specific to our site, as no difference was found between the years across the extant Kooragang Island population. This is problematic, as gravid females were detected only during the last 2 years, when there were few occurrences of males calling. Litoria aurea males are known to mature in as little as 3 months, whereas females typically become sexually mature after 2 or 3 years (Hamer & Mahony, Reference Hamer and Mahony2007). Thus, the successful management of the species may require at least 2–3 years of survival prior to any reproduction events occurring, and initiatives may be susceptible to failure if males disperse during this period to find other breeding opportunities. Moreover, these sex differences coupled with chytrid could lead to a drastic decline if sexually mature individuals die prior to contributing to the population, especially given that juveniles have lower survival rates. Nevertheless, we found juveniles appearing in the unfenced area at the end of the second season, and genetic analyses revealed that a breeding event had occurred near the trial plot. Although they did not breed in our created habitat, released tadpoles survived and metamorphosed, and the habitat was actively selected by dispersing juveniles after metamorphosis. The created habitat may therefore be unsuitable for breeding but may facilitate persistence in the short term. Furthermore, there were multiple breeding events among other amphibian species in the trial plot, indicating that the created site can support amphibian reproduction, albeit perhaps not for this species. Future research should examine the factors influencing the likelihood and success of L. aurea reproduction.
Factors influencing L. aurea abundance included salinity, tadpole and insect diversity, water temperature, season, and wind speed. The negative relationship between water salinity and abundance is probably attributable to the lethal effects of high salinity levels on amphibians because of their water-permeable skin. Abundance was also negatively related to tadpole diversity, possibly because of increased competition from other amphibian species. The presence of other tadpoles may also have prevented breeding, and should be investigated further. The greater abundance of L. aurea in ponds with higher aquatic insect diversity is probably related to a greater variety of prey items, and resource availability (Hamer et al., Reference Hamer, Lane and Mahony2002; Bower et al., Reference Bower, Pickett, Garnham, DeBoo, McCurry and Mengerink2014; Klop-Toker et al., Reference Klop-Toker, Valdez, Stockwell, Fardell, Clulow, Clulow and Mahony2016), and concurs with previous research finding a positive relationship between invertebrate diversity and L. aurea abundance, as well as reducing the likelihood of extinction at an occupied site (Valdez et al., Reference Valdez, Stockwell, Klop-Toker, Clulow, Clulow and Mahony2015). Although air temperature was not significant in our models, water temperature was positively correlated with abundance, possibly because water temperature is more indicative of the weather on a given day. Whereas daily air temperature fluctuates widely, water temperature remains more constant over time because of the thermal inertia of water. This also corresponds with abundance being lowest in the cold winter months, when L. aurea individuals typically seek shelter and overwinter (Hamer et al., Reference Hamer, Lane and Mahony2003). High wind speed was negatively correlated with abundance, as it increases water loss in amphibians (Heyer et al., Reference Heyer, Donnelly, Foster and McDiarmid2014). Thus, to optimize resource use we suggest surveys should be undertaken on nights with higher daytime temperature and low wind.
We found the current habitat template for L. aurea individuals (Pyke & White, Reference Pyke and White1996) was suitable for their growth and survival to adulthood. However, although we placed terracotta tiles and tins as recommended, they were used extensively by predators and therefore it may be advisable to exclude them from future habitat creation and restoration initiatives. Additionally, although a considerable amount of data was generated, this study required significant effort and resources. Less intensive monitoring might have been more cost-effective and resulted in similar conclusions. However, optimal resource use in conservation management is always context dependent and requires balancing the value of gathering more information against its cost (Canessa et al., Reference Canessa, Guillera-Arroita, Lahoz-Monfort, Southwell, Armstrong and Chadès2015). We recommend releasing large numbers of L. aurea closer to those found in nature to compensate for natural predation, disease, and density-dependent effects. Low release numbers have been implicated in the failures of previous management plans (White & Pyke, Reference White and Pyke2008b), and increasing the number of individuals released, especially for a species with low survival and high turnover rates, could ensure successful management outcomes. The biggest limitation was that a breeding event did not occur, and the long-term persistence of L. aurea in the created habitat remains uncertain. Understanding the factors that contribute to successful L. aurea reproduction should be the focus of future research. We also suggest consideration be given to staged releases over multiple years to improve the likelihood of persistence and compensate for lack of breeding, die-offs, and differences in maturation between the sexes.
Achieving successful conservation outcomes requires recognizing how a species responds to active environmental intervention. Although development is rarely delayed whilst research is conducted to assess whether a proposed management plan can meet its objectives, such data are critical to ensure a greater probability of success. Individual trial studies and monitoring prior to large-scale implementation can help determine whether a proposed project is likely to achieve the desired results. The findings from such evidence-based research can also inform future management strategies by identifying potential issues and optimizing resources. This knowledge can lead to optimum conservation strategies to provide equivalent ecological gains elsewhere and prevent further species decline.
Acknowledgements
We thank Matt Edgar, Joe Gibbs and Mitch Cromarty, who assisted with data collection, Kim Colyvas for statistical consulting, Mathew DeBoo for genetic analysis, John Gould for proofreading, and the reviewers for their constructive feedback. This work was funded by BHP Billiton and conducted under Scientific License No. SL 100529 and ethics number A-2010-145.
Author contributions
JV was responsible for data collection, analysis, and writing the article. KKT assisted in data collection. MS, SC, MM and JC assisted in the research design and obtaining grant funding. All co-authors reviewed drafts of the article.
Biographical sketches
Jose Valdez is interested in ecology, conservation and behaviour. Kaya Klop-Toker is interested in wildlife ecology and conservation. Michelle Stockwell’s work is conservation focused and involves projects with applied outcomes for population management and threat mitigation. Loren Fardell’s research focuses on conservation management. Simon Clulow works in a variety of fields, including ecology, conservation, evolution and reproduction in terrestrial vertebrates. John Clulow focuses on conservation and reproductive biology. Michael Mahony focuses on environmental science and management.