Skeletal muscle proteins turn over regularly to the extent that 1–2 % of proteins are synthesised and broken down daily(Reference Welle, Thornton and Statt1). The turnover of proteins involves the ongoing processes of protein synthesis and breakdown, and it is well known that prolonged exercise depresses muscle protein synthesis(Reference Davis and Karl2, Reference Dohm, Kasperek and Tapscott3).
Previous studies have demonstrated that ingestion of a meal containing protein immediately following exercise stimulates protein synthesis in skeletal muscles(Reference Gautsch, Anthony and Kimball4, Reference Williamson, Kubica and Kimball5). In addition, leucine alone stimulates muscle protein synthesis to the same extent as a complete protein or complete mixture of amino acids. This effect is due to the dose-dependent activation of several factors involved in the initiation of mRNA translation, and is mediated primarily through the mammalian target of rapamycin (mTOR) signalling pathway. This pathway includes ribosomal protein S6 kinase (S6K1) and eukaryotic initiation factor 4E-binding protein 1 (4E-BP1)(Reference Crozier, Kimball and Emmert6, Reference Anthony, Lang and Crozier7).
Several researchers(Reference Anthony, McDaniel and Knoll8–Reference Tang, Moore and Kujbida10) have investigated the effect of different protein sources such as whey, soya, casein and wheat on muscle protein synthesis and mTOR signalling. These studies have demonstrated that whey protein stimulates muscle protein synthesis and initiates translation to a greater extent than the other protein sources. This finding led Norton et al. (Reference Norton, Layman and Bunpo9) to suggest that the leucine content of a meal was proportional to the peak activation of muscle protein synthesis. These results indicated that the greater effect of whey protein on muscle protein synthesis was due to its high leucine content. Katsanos et al. (Reference Katsanos, Chinkes and Paddon-Jones11) also suggested that accrual of muscle protein in elderly subjects was greater following ingestion of whey protein than ingestion of its constituent essential amino acid content. Although there is a large volume of evidence on the effect of leucine and leucine-rich intact whey protein on muscle protein synthesis and mTOR signalling, there is only limited information on the effects on these processes of enzymatically hydrolysed whey protein (whey protein hydrolysates; WPH) which contain mostly peptides.
In a previous study, we demonstrated that ingestion of carbohydrate (CHO) plus WPH after glycogen-depleting exercise caused a significantly greater increase in glycogen content than either whey protein or branched-chain amino acids (BCAA)(Reference Morifuji, Kanda and Koga12). That study also indicated that bioactive peptides in WPH caused a greater increase in muscle glycogen synthesis than BCAA. We therefore hypothesised that WPH, which contains bioactive peptides, may cause greater recovery of muscle protein synthesis after endurance exercise. In the present study, we examined whether ingestion of WPH caused a greater increase in muscle protein synthesis after endurance exercise compared with a mixture of amino acids. We also examined whether WPH stimulated mTOR signalling in the same way as leucine.
Experimental methods
Animals
Male Sprague–Dawley rats with body weights of approximately 150 g (CLEA Japan, Inc.) were used in the study. The animals were maintained at 23 ± 2°C, with lights on from 08.00 to 20.00 hours and off from 20.00 to 08.00 hours, and had free access to food (protein 23·6 %, fat 5·3 %, CHO 54·4 %, ash 6·1 %, fibre 2·9 % and moisture 7·7 %; MF; Oriental Yeast Company Limited) and water. The study was approved by the Animal Committee of the Food Science Research Laboratory, Meiji Company Limited, with animals receiving care according to the guidelines laid down by this committee.
Experimental protocol
The animals were acclimatised to swimming exercise by 3 d of pre-training. At 1 d before the experiment, the animals were fed 5 g of a restricted diet (MF; Oriental Yeast Company Limited). On the day of the experiment, the animals either remained in their cages (sedentary controls, n 8) or swam for 2 h, with four rats swimming simultaneously in a barrel filled to a depth of 50 cm, allowing an average surface area of 400 cm2 for each animal. Water temperature was maintained at a constant 35°C during the swimming protocol. Immediately following exercise, one group of rats was killed (exercise controls, n 8) and the other groups (n 8 per group) were given oral administration of one of three isoenergetic test solutions using a sonde. These solutions contained 44 kJ in a 4 ml test dose that represented approximately 15 % of daily energy needs, as determined previously(Reference Gautsch, Anthony and Kimball4), and included either CHO in the form of 50 % glucose plus 50 % sucrose (CHO), a mixed meal containing approximately 18 % amino acid mixture (AA), or a mixed meal containing approximately 23 % WPH (Meiji Company Limited) (Tables 1 and 2). Each test solution was isoenergetic (1100 kJ/100 ml). The AA and WPH meals contained equal amounts of protein, with the protein content of these preparations being measured by the Kjeldahl method(Reference Bradstreet13). The average chain length of the peptides was calculated as the ratio of total N:amino N in the protein samples, which showed that whey protein hydrolysate had an average peptide length of 3·64. The amino acid composition of AA was equivalent to that of WPH and was prepared by mixing pure crystalline l-amino acids (Table 3).
Table 1 Macronutrient composition of the test solutions

CHO, carbohydrate; AA, amino acid mixture; WPH, whey protein hydrolysate.
Table 2 Macronutrient composition of the whey protein hydrolysates (WPH)
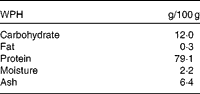
Table 3 Composition of the amino acid mixture
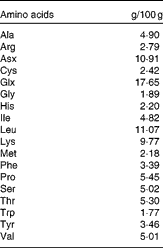
At 1 h after administration, the remaining rats were killed under diethyl ether anaesthesia, and the triceps muscle excised and stored at − 80°C until further use. The decision to investigate muscle recovery 1 h after exercise was based on previous work in rodents, which showed that re-alimentation following exercise caused an increase in muscle protein synthesis within 1 h after refeeding(Reference Gautsch, Anthony and Kimball4).
Administration of metabolic tracer
At 15 min before killing, a bolus dose (45 mg/kg body weight, 22·5 mg/ml) of 2H-labelled phenylalanine ([2H5]Phe; Cambridge Isotope Laboratories) was injected via the tail vein for measurement of the fractional protein synthesis rate (FSR). At 15 min after the injection, the triceps muscle was excised and frozen rapidly. The elapsed time from injection until freezing was recorded as the actual time for incorporation of the labelled amino acid into the protein.
Plasma measurements
Plasma insulin was measured using a commercial ELISA kit for rat insulin (Mercodia AB) and plasma free amino acids by HPLC, with pre-column 6-aminoquinolyl-N-hydroxysuccinimidyl carbamate derivatisation(Reference Morifuji, Ishizaka and Baba14). Plasma glucose was measured by enzymatic methods using a commercial kit (Wako Pure Chemical Industries Limited).
Measurement of protein synthesis
The rate of protein synthesis in individual tissues was measured by the incorporation of injected [2H5]Phe into muscle proteins. Determination of [2H5]Phe enrichment in plasma and hydrolysed muscle protein samples was carried out using a modification of the procedure, described by Bark et al. (Reference Bark, McNurlan and Lang15), that incorporated LC/MS/MS systems (TQD; Waters Corporation) performed on a 2·1 × 50 mm column with a particle size of 1·7 μm (ACQUITY UPLC BEH C18; Waters Corporation). Mobile phase A consisted of 0·05 % trifluoroacetic acid in Milli-Q water, while the mobile phase B consisted of 0·05 % trifluoroacetic acid in acetonitrile. The initial eluent composition was 100 % A, followed by an increase to 40 % B for 9·0 min, 80 % for 1·0 min and then reduction to 100 % A for 3·0 min. The total running time was 12·0 min, eluent flow was 0·3 ml/min and column temperature was set at 40°C. UV trace was recorded at 215 nm, with the analytes detected using electrospray ionisation in the positive mode. Multiple reaction monitoring was performed using characteristic fragmentation ions (m/z 166·19>120·10) for Phe and (m/z 171·19>125·10) for [2H5]Phe. The parameters for the LC/MS/MS analysis were as follows: capillary voltage, 3000 V; source temperature, 120°C; desolvation temperature, 400°C; desolvation gas flow, 849 litres/h; cone gas flow, 48 litres/h; cone voltage and collision energy, 25 V and 15 eV, respectively. FSR, defined as the percentage of tissue protein renewed each day, were calculated according to the formula:

where t is the time interval between injection and cooling of the tissue sample, expressed in d, and E b and E a are the enrichments of [2H5]Phe in hydrolysed tissue protein and in muscle free amino acids, respectively.
Western blotting
Muscle supernatants were subjected to Western blotting as described previously(Reference Morifuji, Kanda and Koga16). Phosphorylation of mTOR at Ser2448 was detected using rabbit anti-phospho-mTOR (Ser2448; Cell Signaling Technology) and expressed as a ratio of total mTOR expression, determined using anti-mTOR (Cell Signaling Technology). Phosphorylation of 4E-BP1 at Thr37/46 was detected using rabbit anti-phospho-4E-BP1 (Thr37/46; Cell Signaling Technology) and expressed as a ratio of total 4E-BP1 expression, determined using anti-4E-BP1 (Cell Signaling Technology). Phosphorylation of S6K1 at Thr389 and (Thr421/Ser424) was detected using rabbit anti-phospho-S6K1 (Thr389; Cell Signaling Technology) and rabbit anti-phospho-S6K1 (Thr421/Ser424; Santa Cruz Biotechnology), respectively. Phosphorylation of S6K1 was then expressed as a ratio of total S6K1 expression, determined using anti-S6K1 (Santa Cruz Biotechnology).
Statistical analysis
Data are expressed as means with their standard errors. All the statistical analyses were performed using SPSS for Windows, version 14.0J (SPSS Japan). Comparison of data was carried out using one-way ANOVA, and when a significant difference in a variable was detected, the groups were compared using Tukey's post hoc analysis. Differences between the groups were considered to be statistically significant at P< 0·05. Associations between the variables were analysed using Pearson's correlation coefficients. Differences between the groups were considered to be statistically significant at P< 0·05.
Results
Fractional rates of protein synthesis
A 2 h period of swimming (exercise controls) reduced FSR by 42 % compared with the sedentary control group. Feeding of CHO alone did not promote the recovery of FSR. In contrast, supplements containing whey-based protein reversed the decrease in FSR. Furthermore, WPH caused significant increases in FSR compared with AA or CHO (Fig. 1).
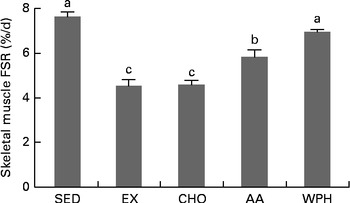
Fig. 1 Fractional rates of protein synthesis (FSR) in rats administered diets that differed in molecular forms of dietary whey-based protein, measured 1 h after swimming activity. Values are means (n 8), with their standard errors represented by vertical bars. a,b,cMean values with unlike letters were significantly different (P <0·05; Tukey's post hoc analysis). SED, sedentary control; EX, exercise control; CHO, carbohydrate; AA, amino acid mixture; WPH, whey protein hydrolysate.
Plasma insulin and amino acid levels
Plasma insulin levels were higher in the WPH group than in the CHO group (Table 4). However, there was no significant difference in insulin levels between the AA and WPH groups. Ingestion of whey-based protein caused a greater increase in plasma concentrations of leucine, total amino acids, essential amino acids and BCAA compared with CHO (Table 4), whereas there was no significant difference in these levels between the AA and WPH groups. Plasma glucose levels did not differ between the groups.
Table 4 Plasma amino acid and insulin levels of rats administered diets that differed in the molecular forms of dietary whey-based protein, measured 1 h after swimming (Mean values with their standard errors)
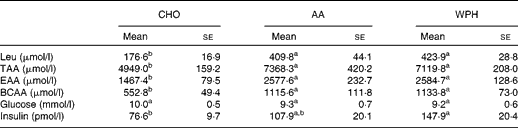
CHO, carbohydrate; AA, amino acid mixture; WPH, whey protein hydrolysate; TAA, total amino acids; EAA, essential amino acids; BCAA, branched-chain amino acids.
a,bMean values within a row with unlike superscript letters were significantly different (P< 0·05; Tukey's post hoc analysis).
Phosphorylated mammalian target of rapamycin, eukaryotic initiation factor 4E-binding protein 1 and ribosomal protein S6 kinase levels
Post-exercise ingestion of glucose plus WPH caused a significant increase in phosphorylated mTOR levels compared with AA or CHO (Fig. 2(A)). The WAA group also showed a significant increase in phosphorylated mTOR compared with the CHO group.
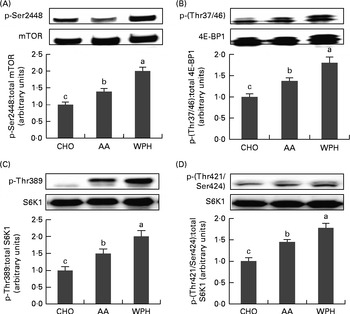
Fig. 2 Skeletal muscle phosphorylated (A) mammalian target of rapamycin (mTOR), (B) eukaryotic initiation factor 4E-binding protein 1 (4E-BP1), ribosomal protein S6 kinase (S6K1) and (C) Thr389 and (D) Thr421/Ser424 levels of rats administered diets that differed in molecular forms of dietary whey-based protein, measured 1 h after swimming. Phosphorylated mTOR was normalised for total mTOR, phosphorylated 4E-BP1 for total 4E-BP1 and phosphorylated S6K1 for total S6K1. Values are means (n 8), with their standard errors represented by vertical bars. a,b,cMean values with unlike letters were significantly different (P< 0·05; Tukey's post hoc analysis). CHO, carbohydrate; AA, amino acid mixture; WPH, whey protein hydrolysate.
The WPH group had greater phosphorylation of 4E-BP1 compared with both the AA and CHO groups (Fig. 2(B)). The AA group also exhibited a significant increase in phosphorylated 4E-BP1 compared with the CHO group.
Phosphorylation of S6K1 at Thr389 and Thr421/Ser424 was greater in the WPH group than in the AA and CHO groups (Fig. 2(C) and (D), respectively). The AA group also demonstrated greater phosphorylation of S6K1 at both these sites compared with the CHO group.
Discussion
In the present study, we compared the effect of different molecular forms of dietary protein on the recovery of muscle protein synthesis and mTOR signalling after endurance exercise. We exercised rats using 2 h of swimming to depress muscle protein synthesis. Recovery of muscle protein synthesis was stimulated by whey-based protein (AA or WPH) supplementation. Furthermore, the WPH group showed significantly higher muscle protein synthesis compared with the AA group. This is the first study to demonstrate that WPH is more effective in the recovery of skeletal muscle protein synthesis than a mixture of whey amino acids, when given as the protein source after exercise. Furthermore, the present results suggest that it is possible that certain bioactive peptides contained in WPH are superior to amino acids for stimulating muscle protein synthesis.
It is well known that BCAA, especially leucine, play an important role in the activation of muscle protein synthesis(Reference Escobar, Frank and Suryawan17, Reference Blomstrand, Eliasson and Karlsson18). Leucine alone or as a supplement has been shown to stimulate muscle protein synthesis after exercise in models of both endurance and resistance exercise(Reference Anthony, Anthony and Layman19, Reference Koopman, Wagenmakers and Manders20), leading Norton et al. (Reference Norton, Layman and Bunpo9) to suggest that peak activation of muscle protein synthesis was proportional to the leucine content of a meal. On the other hand, the amino acid compositions of the two solutions used in our study (WPH and AA) were equivalent, and therefore total BCAA and leucine intake was equal. In addition, plasma amino acid levels did not differ between the two groups, although the effects of the two diets on muscle protein synthesis varied. This result strongly suggests that not only the leucine content in dietary protein but also the molecular form of dietary protein sources may be important determinants of muscle protein synthesis. Furthermore, we conclude that WPH used in the present study contained active components that are superior to leucine itself for increasing muscle protein synthesis. Recently, we demonstrated that BCAA-containing peptides in WPH, such as Ile-Val, Leu-Val, Val-Leu, Ile-Ile, Ile-Leu, Leu-Ile and Leu-Leu, increased the rate of glucose uptake in isolated rat skeletal muscle. This finding suggested that these BCAA-containing peptides are bioactive peptides that promote muscle glycogen synthesis(Reference Morifuji, Koga and Kawanaka21). Furthermore, we have shown that the plasma levels of these peptides were elevated markedly after ingestion of WPH(Reference Morifuji, Ishizaka and Baba14). It is therefore possible that BCAA-containing peptides may act as bioactive peptides in both glycogen and muscle protein synthesis in skeletal muscle.
mTOR signalling is a key regulatory factor for muscle protein synthesis and is regulated positively by amino acids, particularly leucine(Reference Drummond, Dreyer and Fry22–Reference Findlay, Yan and Procter24). In the present study, ingestion of WPH caused significantly greater phosphorylation of mTOR levels compared with a mixture of amino acids, despite the plasma levels of amino acids in AA and WPH being similar. Taken together, these results indicated that active components, such as bioactive peptides, contained in WPH have a greater effect on mTOR phosphorylation than leucine degraded from WPH. It is also known that two well-studied downstream targets of mTOR, S6K1 and 4E-BP1, influence translation initiation and elongation(Reference Baar and Esser25, Reference Wang and Proud26). In the present study, we demonstrated that phosphorylation of S6K1 and 4E-BP1 was higher in the WPH group than in the AA group. These results indicate that WPH caused greater phosphorylation of S6K1 and 4E-BP1 via mTOR activation than AA. Karlsson et al. (Reference Karlsson, Nilsson and Nilsson27) also demonstrated that BCAA increase the phosphorylation of S6K1 at Thr421/Ser424 without activating mTOR(Reference Hornberger, Stuppard and Conley28). Taken together, these earlier findings and the present results suggest that certain bioactive peptides in WPH may also activate S6K1 via phosphorylation of Thr421/Ser424 without activating mTOR.
We also showed that plasma levels of dipeptides were elevated markedly after ingestion of WPH(Reference Morifuji, Ishizaka and Baba14). However, the plasma levels of dipeptides were lower than those of plasma amino acids. In the present study, plasma leucine levels in the AA and WPH groups were 409·8 and 423·9 μmol/l, respectively. In contrast, plasma dipeptide levels, which are based on WPH, were extremely low compared with leucine levels. For example, the plasma levels of Ile-Val, Leu-Val, Val-Leu, Ile-Ile, Leu-Ile, Ile-Leu and Leu-Leu were 11·1 (se 1·4), 6·0 (se 0·7), 25·9 (se 3·1), 9·4 (se 1·1), 1·7 (se 0·2), 13·2 (se 1·3) and 34·2 (se 4·3) nmol/l, respectively, in the WPH group (A Kanda, K Nakayama, T Fukasawa, J Koga and K Kawanaka, unpublished results). On the other hand, these dipeptides were not detected in the AA group. Additional studies are therefore required to determine whether these dipeptides caused stimulation of mTOR signalling at lower plasma concentrations than leucine.
Previous studies(Reference Kimball, Farrell and Jefferson29) have indicated that a minimal concentration of plasma insulin is required for both amino acid- and exercise-induced stimulation of translation initiation and protein synthesis in skeletal muscle. In particular, insulin availability is important in mTOR-mediated signalling. We have shown previously that the plasma insulin response during the 0–60 min period was higher for protein hydrolysates than for whole protein(Reference Morifuji, Ishizaka and Baba14). In the present study, the WPH group had higher plasma insulin levels than the AA group, although this difference was not statistically significant. We also observed a significant correlation between insulin and both FSR (r 0·51, P= 0·012) and phosphorylated mTOR levels (r 0·66, P< 0·001). Taken together, these findings suggest that a greater increase in plasma insulin levels caused by WPH may induce a larger increase in FSR via activation of mTOR signalling compared with AA.
It is well known that swimming exercise is a highly stressful activity that results in an increased release of glucocorticoids(Reference Korz and Frey30). The association between glucocorticoid excess and muscle atrophy is well established(Reference Rannels and Jefferson31). Inhibition of protein synthesis has been observed consistently in glucocorticoid-treated animals(Reference Odedra, Bates and Millward32). In addition, the potent synthetic glucocorticoid dexamethasone also prevents amino acid-induced hyperphosphorylation of S6K1 and 4E-BP1, as well as stimulating protein synthesis in muscle(Reference Shah, Anthony and Kimball33). It is therefore possible that WPH caused an increase in muscle protein synthesis not only by activating mTOR signalling, but also by attenuating the effect of glucocorticoids. Further studies are therefore required to investigate the effects of WPH on glucocorticoids.
In conclusion, the present results demonstrate that ingestion of WPH increases muscle protein synthesis to a greater extent than a mixture of amino acids after endurance exercise as a result of greater phosphorylation of mTOR levels. These results indicated that WPH may contain active components, such as bioactive peptides, that are superior to leucine for stimulating mTOR signalling. The findings of the present study therefore provide new insights into the effects of different forms of dietary protein for sports nutrition.
Acknowledgements
No funding was received for the present study. A. K. and K. N. designed the research; A. K., T. F., J. K., M. K., K. K. and M. H. conducted the research; A. K. analysed the data, wrote the paper and had primary responsibility for the final content. All authors read and approved the final manuscript. The authors have no conflicts of interest to declare.