1. Introduction
Angiotensin converting enzyme (ACE) is a membrane-bound dipeptidyl carboxypeptidase ectoenzyme that is expressed both peripherally and in the central nervous system. ACE is mainly responsible for the production of angiotensin II, a potent vasoconstrictor, and the inactivation of the potent vasodilator bradykinin. Because of its broad-ranging effects on vascular homeostasis, ACE has become a candidate for association studies with common diseases. The ACE gene maps to chromosome 17q23, spans 21 kb, and comprises 26 exons and 25 introns (Hubert et al., Reference Hubert, Houot, Corvol and Soubrier1991); the GenBank accession number is AC002345 or AF118569 (www.ncbi.nlm.nih.gov). To date, 259 polymorphisms have been reported in the ACE gene (www.ncbi.nlm.nih.gov), with the I/D polymorphism, first reported by Rigat et al. (Reference Rigat, Hubert, Alhenc-Gelas, Cambien, Corvol and Soubrier1990), attracting the most interest. This I/D polymorphism is defined by the presence (insertion; I) or absence (deletion; D) of a 287 base pair (bp) Alu repeat sequence in intron 16 (Rieder et al., 1999). The ACE I/D polymorphism has been linked to various functional effects, for example the DD genotype being associated with high plasma ACE levels in addition to numerous diseases. One of the most extensively studied associations is with cardiovascular diseases, including myocardial infarction (Cambien et al., Reference Cambien, Poirier, Lecerf, Evans, Cambou, Arveiler, Luc, Bard, Bara and Ricard1992; Nakai et al., Reference Nakai, Itoh, Miura, Hotta, Musha, Itoh, Miyakawa, Iwasaki and Hiramori1994), left ventricular hypertrophy and dysfunction (Schunkert et al., Reference Schunkert, Hense, Holmer, Stender, Perz, Keil, Lorell and Riegger1994), dilated cardiomyopathy (Raynolds et al., Reference Raynolds, Bristow, Bush, Abraham, Lowes, Zisman, Taft and Perryman1993; Harn et al., Reference Harn, Chang, Ho, Liu, Jeng, Lin and Jent-Wei1995) hypertrophic cardiomyopathy (Marian et al., Reference Marian, Yu, Workman, Greve and Roberts1993), carotid thickening (Castellano et al., Reference Castellano, Muiesan, Rizzoni, Beschi, Pasini, Cinelli, Salvetti, Porteri, Bettoni and Kreutz1995; Kauma et al., Reference Kauma, Paivansalo, Savolainen, Rantala, Kiema, Lilja, Reunanen and Kesaniemi1996), venous thrombosis (Philipp et al., Reference Philipp, Dilley, Saidi, Evatt, Austin, Zawadsky, Harwood, Ellingsen, Barnhart, Phillips and Hooper1998), nephropathy (Schmidt & Ritz, Reference Schmidt and Ritz1997) and coronary restenosis after stent implantation (Amant et al., Reference Amant, Bauters, Bodart, Lablanche, Grollier, Danchin, Hamon, Richard, Helbecque, McFadden, Amouyel and Bertrand1997). Currently, the best evidence for an association with the ACE I/D polymorphism is with arterial hypertension in men (Fornage et al., Reference Fornage, Amos, Kardia, Sing, Turner and Boerwinkle1988; Higaki et al., Reference Higaki, Baba, Katsuya, Sato, Ishikawa, Mannami, Ogata and Ogihara2000); one study of male carriers of the DD genotype showed a 1·6-fold increase in risk (O'Donnell et al., Reference O'Donnell, Lindpaintner, Larson, Rao, Ordovas, Schaefer, Myers and Levy1998). It has also been suggested that the ACE I/D polymorphism may contribute to an individual's susceptibility to affective disorders and the onset of action of antidepressant therapies (Arinami et al., Reference Arinami, Li, Mitsushio, Itokawa, Hamaguchi and Toru1996; Baghai et al., Reference Baghai, Schule, Zwanzger, Minov, Schwarz, de Jonge, Rupprecht and Bondy2001, 2004, 2005; Gard et al., Reference Gard2004; Saab et al., Reference Saab, Gard, Yeoman, Mfarrej, El-Moalem and Ingram2007b). The ACE DD genotype was also found to be a significant risk factor for children with congenital renal malformations going on to develop progressive renal failure (Hohenfellner et al., Reference Hohenfellner, Wingen, Nauroth, Wuhl, Mehls and Schaefer2001). Also, it was found that in patients with lupus nephritis, the ACE I/D genotype was associated with the severity of the disease and a poor prognosis (Guan et al., Reference Guan, Liu and Chen1997).
Saab et al. (Reference Saab2004) have typed the ACE I/D gene polymorphism in the Lebanese population, where the homozygous II genotype accounted for 8% of the sample – an incidence that was found to be atypically low relative to European and East Asian populations. These preliminary results suggested that the ACE II genotype frequency might vary according to a geographic trend, as has been postulated for other Alu insertion polymorphisms (Stoneking et al., Reference Stoneking, Fontius, Clifford, Soodyall, Arcot, Saha, Jenkins, Tahir, Deininger and Batzer1997). The objective of the present study was to determine whether the ACE I/D gene polymorphism frequency did indeed correlate with geographic distance and then identify whether the ACE gene can be considered as a genetic marker for the past demography of human populations.
2. Materials and methods
(i) ACE genotype frequency determination in Lebanese subjects
A total of 570 healthy volunteers were recruited from the Lebanese population. Included were non-obese subjects (body mass index (BMI) <29·5 kg/m2) with no history or clinical evidence of diabetes, cardiovascular problems, hypertension, renal insufficiency and/or depression. All study subjects are of Lebanese origin, and were living in Lebanon at the time of study. Exclusion criteria were set to achieve parity with other studies.
(ii) Sample collection and DNA extraction
Each volunteer was instructed to give a DNA sample from the cheek using a cheek swab. The sample was used for DNA extraction. DNA was extracted using a protocol described by Saab et al. (Reference Saab, Gard, Yeoman, Mfarrej, El-Moalem and Ingram2007b).
(iii) ACE I/D gene polymorphism genotyping
The presence of the insertion/deletion allele in intron 16 of the ACE gene was detected using the method of Rigat et al. (Reference Rigat, Hubert, Alhenc-Gelas, Cambien, Corvol and Soubrier1990) with some modifications (Sery et al., Reference Sery, Vojtova and Zvolsky2001). The sequence of the sense oligonucleotide primer is 5′-CTG GAG ACC ACT CCC ATC CTT TCT-3′ and the antisense primer 5′-GAT GTG GCC ATC ACA TTC GTC AGA T-3′. Polymerase chain reaction was performed in a final volume of 25 μl containing 50 mM KCl, 10 mM Tris-HCl, pH 8·4, 5 U/μl MgCl2, 0·5 mM of each dNTP, 0·6 U Taq DNA polymerase, 0·2 μM of each primer, and 3 μl of DNA solution. PCR products were separated and sized by electrophoresis on a 2·5% agarose gel and visualized directly with ethidium bromide staining. The insertion allele manifested as a 490 bp band, and the deletion allele was visualized as a 190 bp band. Because of the possibility of preferential amplification of the D fragment in relation to the I fragment, resulting in mistyping of I/D as DD genotype, all DD genotypes were confirmed (Odawara et al., Reference Odawara, Matsunuma and Yamashita1997).
(iv) ACE gene geographic mapping
We identified literature, published in English between 1984 and 2006, reporting ACE I/D gene polymorphisms. The extracted data are summarized and tabulated in Table 2. Excluded were studies of a small sample size (<48), studies where the subjects' origins were unknown and where subjects were known to be suffering from a disease.
The exception was the Kuwait sample, which comprises 48 individuals suffering from nephropathy. This sample was included due to the shortage of available samples from the Middle East, but appears to display allele and genotype frequencies consistent with those observed in the region. In addition, the genotypes did not appear to be inconsistent with Hardy–Weinberg expectations.
(v) Statistical analysis
Statistical analyses were performed using SPSS version 12 for Windows. The study samples' allele and genotype frequencies were estimated by the gene counting method. The agreement with Hardy–Weinberg equilibrium of the observed genotypic distribution for the ACE I/D alleles was tested using Fisher exact tests. A P value of <0·05 was considered statistically significant.
Genetic distances were estimated assuming that differences in allele frequency distributions between populations were due to drift. Pairwise distances were calculated as d=ln (1 – F ST) (Weir, Reference Weir1996). Nei's genetic distances (Nei & Feldman, Reference Nei and Feldman1972) were also calculated for the purpose of constructing neighbour-joining trees (Saitou & Nei, Reference Saitou and Nei1987) using the GENDIST and NEIGHBOR programs in PHYLIP 3.65 (Felsenstein, Reference Felsenstein1993) and the genetic data analysis package GDA (Lewis & Zaykin, Reference Lewis and Zaykin2001). In most cases, specific geographic locations relating to the samples were not specified in the literature, so geographic distances were taken as pairwise distances (in kilometres) between capital cities of the country in question. To identify whether any correlation exists between the two matrices (genetic and geographic distances), a Mantel test was performed (10 000 permutations, using the Pearson correlation coefficient) using XLSTAT (Kovach Computing Services, 2007).
3. Results
(i) Subjects' demographic characteristics
A total of 570 Lebanese subjects were included in the study, which aimed to determine the ACE gene I/D polymorphism prevalence in the Lebanese population. The study samples consisted of 51·9% and 48·1% males and females, respectively. The mean age was 28·63 years (range 18–69 years) and the average BMI was 23·04 kg/m2 (range 17·15–28·41 kg/m2).
(ii) ACE genotype distribution in Lebanese and Hardy–Weinberg equilibrium
The detailed distribution of the ACE genotypes in the Lebanese population is depicted in Table 1. The prevalence of the D allele was 73%, and the II genotype accounted for 7·37%. Genotype frequencies were found to be in Hardy–Weinberg equilibrium (P=0·743, Fisher exact test, 10 000 permutations).
Table 1. ACE I/D observed genotypes/allele frequencies in the Lebanese population compared with expected genotypes

(iii) ACE II genotype prevalence among different populations
The ACE allele frequencies of different populations retrieved from the literature, along with that of this study's finding, are depicted in Table 2. The results suggest that the ACE II genotype frequency decreases according to a geographic trend from northern Europe to southern Europe, and on to the Mediterranean region. Moreover, moving geographically eastward, the II genotype prevalence appears to increase progressively. The results of the Mantel test show a reasonable and significant correlation between geographic and genetic distances between the populations (r=0·478984, P<0·0001). On further analysis, it appears that this is largely influenced by II frequency and longitude correlation (R 2=0·727), where the II genotype frequency declines on moving from Europe to the Middle East, followed by an increase moving eastwards to Asia (Fig. 1). There was no meaningful relationship between II genotype frequency and latitude (R 2=0·027). For the correlation with longitude, the quadratic relationship proved to be more significant than the linear relationship (starting with a GLM maximal model: II frequency=β0+β1x 12+β2x 2, where x is the degrees east of Greenwich, UK and the β parameters were estimated by maximum likelihood; removing the quadratic term resulted in a significant difference between the deviance values of the two models (Crawley, Reference Crawley1993), where P=0·0001). The correlation between I allele frequency and longitude gave a slightly weaker relationship than that with the II genotype (R 2=0·54). In brief, the II genotype had an average frequency of 23% in northern Europe, 20% in the UK, 15% in Spain, 14% in north Italy, 12% in south Italy, 7% in Lebanon, 6% in the United Arab Emirates (UAE), 2% in Kuwait, and then an average of 35% in China and 45% in Japan.

Fig. 1. Plot of ACE II genotype frequencies and coordinates east of Greenwich, UK. For the linear relationship R 2=0·599; for the quadratic, R 2=0·727· The Lebanese population is circled.
Table 2. ACE II genotype frequency in different populations/countries
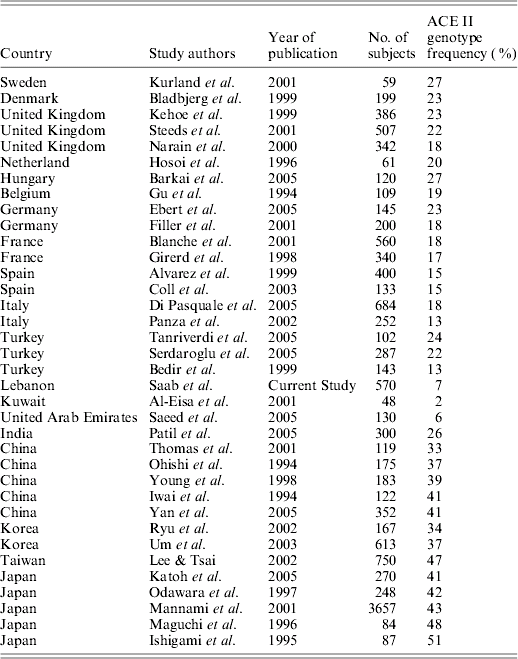
Fig. 2 shows the neighbour-joining tree relating all 36 population samples using Nei's genetic distance (Nei & Feldman, Reference Nei and Feldman1972). Because the ancestral state of the Alu insertion polymorphisms is considered to be the absence of the insertion, the tree could be rooted using a hypothetical outgroup consisting of individuals fixed for the D allele. The multiple population samples of identical country of origin were averaged to condense the tree.

Fig. 2. Neighbour-joining tree of population relationships. The tree is rooted by a hypothetical ancestral population fixed for the ACE D allele. UAE, United Arab Emirates.
4. Discussion
(i) ACE I/D genotype distribution
According to a meta-analysis of 145 studies with 49 959 subjects, the overall prevalence of the D allele was 54·0%. The II, ID and DD genotype frequencies were 22·5%, 47·0% and 30·5%, respectively (Staessen et al., Reference Staessen, Ginocchio, Wang, Saavedra, Soubrier, Vlietinck and Fagard1997). Ethnicity was a major determinant of the D and I allele frequencies as the prevalence of the D allele was 39·1% in Asians, 56·2% in Caucasians and 60·3% in blacks (Staessen et al., Reference Staessen, Ginocchio, Wang, Saavedra, Soubrier, Vlietinck and Fagard1997). In the present study, the D allele had a frequency of 73·42%, which is consistent with the other two Middle Eastern populations (Kuwait and UAE) in being amongst the highest recorded.
(ii) ACE I/D gene polymorphism: a genetic marker
The average ACE II genotype frequency in control subjects in different populations of different countries was thoroughly examined and compared. Nevertheless, we accept that the comparison of the allele and genotype frequencies with other published studies has to be considered with some caution since published data might have been generated using slightly different methodologies, and thus there is a possibility of discrepancies in genotype classification (Ueda et al., Reference Ueda, Heeley, Lees, Elliott and Connell1996) – in particular, since some genotyping methodologies misclassify ID heterozygotes as DD homozygotes. Although such a misclassification can result in deviations from Hardy–Weinberg equilibrium, which was not observed in our study, it was considered prudent to base our analysis on the II genotype frequencies in addition to the I allele frequencies. Indeed, it may be for this reason that the stronger correlation with longitude was observed with the genotype data than the allele frequency scores.
Genetic polymorphisms have often been found to show geographic clines, many of which have been put to great use in offering insights into the historical movements of peoples around the globe since at least as far back as Neolithic times (Cavalli-Sforza et al., Reference Cavalli-Sforza and Piazza1993; Barbujani et al., Reference Barbujani, Bertorelle and Chikhi1998). Such interpretations of clines are not without their critics (e.g. Richards & Sykes, Reference Richards and Sykes1998) and care is required not to over-interpret geographic patterns when they emerge. In particular, little confidence can be placed in the timing of population movement. Broader conclusions, such as identifying the origin of a particular polymorphism on the basis of its relative frequency, are less controversial; and this is more so with Alu elements, which are considered to be highly stable polymorphisms, where deletion of newly inserted elements is a rare event (Stoneking et al., Reference Stoneking, Fontius, Clifford, Soodyall, Arcot, Saha, Jenkins, Tahir, Deininger and Batzer1997). Low frequencies of the insertion are therefore indicative of the ancestral state, and African populations tend to have not only the lowest frequency of the insertion (Bayoumi et al., Reference Bayoumi, Simsek, Yahya, Bendict, Al-Hinai, Al-Barwani and Hassan2006) but also the greatest variation in frequency (Stoneking et al., Reference Stoneking, Fontius, Clifford, Soodyall, Arcot, Saha, Jenkins, Tahir, Deininger and Batzer1997). On this basis it would appear that the ALU deletion within the ACE gene was, of the populations studied here, Middle Eastern in origin. Given that the human migration out of Africa is likely to have journeyed through the Middle East before migrating east and west, it is to be expected that the Lebanese population should be ancestral with regard to the ACE polymorphism and to have a relatively lower frequency of the insertion allele; this is borne out in the frequency–coordinate correlation analysis in Fig. 1, where a significant quadratic relationship was observed between both the I allele frequency (not shown) and II genotype frequency and the coordinates east of Greenwich, UK. The picture is less clear in the tree reconstruction in Fig. 2. Although the Middle Eastern populations appear quite distinct from both European and Asian populations, these latter groups are not well resolved, most likely due to the fact that only a single locus has been investigated for these populations.
In the analysis of modern human origins, genetic maps demonstrating allelic clines have been quite revealing. Classical attempts to distinguish distinct ancestries of human subgroups (Cavalli-Sforza et al., Reference Cavalli-Sforza, Menozzi and Piazza1996) have been quite successful in employing classical genetic markers, such as the different gene frequencies of A and B blood antigens. Consequently, ABO blood groups have been used as a genetic marker to differentiate human subgroups, on the basis of their distinct demographic histories. It is also considered that the frequency of an allele is likely to be higher at its place of origin as well as in the region where selective factors favour it. ABO gene frequencies again offer an example of a gene that follows this trend (Cavalli-Sforza et al., Reference Cavalli-Sforza, Menozzi and Piazza1996). The gradient of decreasing frequencies has also been shown with haplotypes V and VI (Lucotte et al., Reference Lucotte, Gérard and Mercier2001). Mapping ACE I/D polymorphism genotype frequencies from both this study and those of other authors on to a geographic map, shows the ACE gene to have a geographic trend of expansion consistent with what is known about the migration of modern Homo sapiens out of Africa, thus qualifying the ACE gene as another useful marker tool for studying prehistoric human demography. It remains to be seen, however, whether disorders associated with the ACE gene show geographic trends corresponding with the major polymorphisms, including the Alu I/D.
5. Conclusion
In summary, in view of the reported associations of ACE gene polymorphisms and different diseases, ACE genotypes were assessed in the Lebanese population. The II genotype frequency was 7·37%. Comparing this study's finding with that of other studies in different populations, the ACE gene can be considered a useful genetic marker for gaining an insight into the historical migrations of human populations, in particular the frequency cline of the wild-type D allele. Future pharmacogenetic studies are likely to reveal the natural selection for this gene's geographic variation and the pharmacological role of this enzyme in different populations.
We acknowledge the Lebanese American University (LAU) for funding the project. We thank Dr Hussam Atat, Mr Bechara Mfarej and LAU Pharm D graduates of 2004 and 2005 for their assistance in the sample collection and laboratory work.