Human energy balance and obesity
Forty per cent of the world's population of 7·7 billion people are chronically over or undernourished in roughly equal proportions(1). This suggests that at global, national, community and individual level energy balance (EB) remains a key societal challenge. It is important to consider EB from a multi-disciplinary perspective. The causes and consequences of energy imbalance operate at the societal, policy, public health and individual level(Reference Shrimpton and Rokx2). Addressing this challenge necessarily requires consideration of the physiological systems believed to regulate EB, their impact on human behaviour and their interaction with the environment in which such putative regulatory systems operate. This paper focuses on physiological and behavioural responses to negative EB and the potential role for longer-term weight management interventions.
Obesity is a chronic relapsing condition with multiple co-morbidities. Such complex conditions have a large behavioural component to their development and maintenance. Management requires concerted action through multi-component interventions aimed at both prevention and treatment(Reference Craig, Dieppe and Macintyre3). To date no one organisation, approach or sector has made a significant impact on long-term obesity prevalence trends. Evidence-based interventions and commercial programmes for weight loss (WL) are widely available(Reference Gudzune, Doshi and Mehta4,Reference Vakil, Chaudhry and Doshi5) . In developed countries approximately 42 % of adults report trying to lose weight and 23 % report trying to maintain weight annually(Reference Santos, Sniehotta and Marques6). Eighty per cent of those who achieve clinically significant WL fail to sustain that WL over a period of 12 months or more(Reference Wing and Phelan7). Factors responsible for weight regain include physiological resistance to WL(Reference Stubbs, Hopkins and Finlayson8) (see later), the obesogenic environment(Reference Swinburn, Sacks and Hall9), individual experience of stress and life events and emotional eating(Reference Sainsbury, Evans and Pedersen10), and a general lack of knowledge on the part of the general public on how to effectively manage EB behaviours (dietary intake and physical activity), perhaps exacerbated by the extensive use of popular rather evidence-based weight management practices. Thus, the development of obesity and resistance to WL is due to an interaction between physiology and behaviour in a food, lifestyle and marketing environment that facilitates over-consumption of energy and low levels of physical activity energy expenditure (EE)(Reference Swinburn, Sacks and Hall9). Many marketed solutions for WL tend not to comprehensively implement evidence-based components of behaviour change interventions for weight management(Reference Gudzune, Doshi and Mehta4,Reference Johnston, Kanters and Bandayrel11) . The actual evidence for effective components of weight management (i.e. WL and WL maintenance (WLM)) programmes is limited(Reference Sniehotta, Simpson and Greaves12). Obesity research and practice tend to focus on separate domains of physiology and health on the one hand, and behaviour change solutions on the other. This segmented approach may limit the effectiveness of longer-term WL interventions.
This paper considers (i) how energy deficits influence physiology and behaviour, (ii) how those responses may shape our ability to develop evidence-based practices to help people manage long-term EB and (iii) the types of evidence needed to inform more effective and personalised interventions for longer-term weight management.
Physiological and behavioural responses to energy deficits
Human responses to WL are dynamic and complex. WL follows exponential decay curves in three phases: a relatively short phase 1, a slower, more-steady rate of WL in phase 2, which (if WL unfortunately continues) is followed by phase 3, a shorter, pre-mortal phase(Reference Heymsfield, Thomas and Nguyen13,Reference Heymsfield, Gonzalez and Shen14) . Phase 1 is accompanied by rapid decrease in weight, depletion and change in the composition of fat-free mass (FFM), which stabilises to a slower rate of loss after 4–6 weeks (maximally 12) depending on factors such as initial body composition, extent of energy deficit, degree of exercise and sex of subjects(Reference Heymsfield, Thomas and Nguyen13,Reference Heymsfield, Gonzalez and Shen14) . Phase 2 is characterised by a decrease in the rate of FFM loss and an increase in the rate of mobilisation of body fat(Reference Heymsfield, Thomas and Nguyen13). The majority of voluntary WL interventions operate across phase 1 and into phase 2 of WL suggesting that dynamic changes in multiple physiological systems operate to oppose initial WL by reducing EE and defending the loss of FFM from further rapid loss(Reference Heymsfield, Thomas and Nguyen13). These physiological changes may also be associated with compensatory changes in eating and physical activity behaviours(Reference Stubbs, Hopkins and Finlayson8). The effectiveness of behaviour change interventions for weight management could potentially be improved by considering those interventions in the quantitative context of EB physiology (Fig. 1).

Fig. 1. (Colour online) Schematic diagram illustrating how initial body composition, rate and extent of weight loss can affect the proportion of fat (green curve) and fat free mass (red curve) lost (per cent fat free mass loss is greater at higher rate, extent and lower initial fat mass), the impact of these changes in body structure on physiological functions (fat mass, green lines; fat free mass, red lines) and their likely impact on energy balance (EB) behaviours. Behaviour change interventions attempt to override the compensatory EB behaviours that resist weight loss. Physiological and behavioural responses occur throughout the process of weight loss and are likely to escalate on going from stages one through two of weight loss. Note: Proportionate body composition changes are given for overweight subjects assigned to energetic restriction and very low-energy diets, achieving 10–14 % weight loss from Heymsfield et al.(Reference Heymsfield, Thomas and Nguyen13). The proportion of fat and fat-free mass lost would vary with initial body composition, rate and extent of weight loss.
Physiological models of EB regulation and weight management interventions should account for a number of salient features of the way human EB behaves, namely the inherent asymmetry of EB regulation, which underlies (i) the tendency for most human subjects to gain weight under modern environmental conditions, (ii) to regain weight in response to weight management attempts and (iii) the dynamic physiological responses to energy deficits and their potential impact on behaviour. Some integrative functional body composition models of EB regulation are beginning to unravel the dynamic relationships between body structure, physiological function and the way these interactions influence human behaviour and health(Reference Stubbs, Hopkins and Finlayson8).
It has been argued that the asymmetry of EB regulation in many mammalian species is due to an evolutionary history characterised by alternations between periods of feast with those of famine. Environmental uncertainty in the food supply would result in physiological and behavioural programmes that favoured the deposition of reserves in the adipose tissue when good quality food was readily available(Reference Mela and Rogers15,Reference Stubbs and Tolkamp16) . In other words, the capacity for overconsumption and a tendency to gain weight in the face of nutritional abundance appears to be a strategy for survival and reproduction that evolved in resource-limiting environments(Reference Stubbs and Tolkamp16). Such design characteristics of human eating behaviour would only become maladaptive in environments where the nutritional supply is superabundant, energy-dense and hyperpalatable. Human subjects have manufactured and currently live under such environmental conditions.
Physiological resistance to weight loss
Body composition
The composition of WL is not constant as WL proceeds(Reference Heymsfield, Thomas and Nguyen13,Reference Heymsfield, Gonzalez and Shen14,Reference Thomas, Schoeller and Redman17–Reference Hall, Sanghvi and Göbel22) . The rapid depletion of FFM in phase 1 of WL is associated with rapid glycogen losses, initial (relatively large) losses of nitrogen (i.e. protein) and associated body water(Reference Heymsfield, Thomas and Nguyen13). The rate of these dynamic changes in FFM tends to stabilise beyond 4–6 weeks such that glycogen is depleted, protein and water losses decelerate dramatically and the proportion of fat mass (FM) contributing to WL increases as WL progresses(Reference Heymsfield, Thomas and Nguyen13). Hence, both the proportion of body WL as FFM decelerates and the contribution of glycogen and water to FFM loss decrease throughout phase 1(Reference Heymsfield, Thomas and Nguyen13). Furthermore, the source of protein losses changes from phase 1 to phase 2, largely coming from peripheral organs in phase 1 and shifting to skeletal mass in phase 2(Reference Heymsfield, Thomas and Nguyen13). These changes are associated with reductions in energy requirements and increases in the energy density of tissues lost that collectively tend to reduce the overall rate of WL, for a given energy deficit, beyond 4–6 weeks. During phase 2 of WL the proportionate contribution of FFM to WL decreases and the contribution of FM to WL increases along exponential decay curves that are heavily influenced by initial body fatness at the start of WL, the severity of the energy deficit, physical exercise, macronutrient composition of the diet, sex and some hormones and drugs(Reference Heymsfield, Gonzalez and Shen14). It appears that visceral adipose tissue may be preferentially mobilised in the earlier stages of FM loss(Reference Chaston and Dixon23,Reference Hall and Hallgreen24) . In essence, a greater FM operates as a buffer that defends the loss of FFM during WL(Reference Hall25). The dynamic changes in body composition within phase 1 and the shift in tissues mobilised across phase 2 of WL account for the exponential decay curve of body weight during a prolonged energy deficit(Reference Heymsfield, Thomas and Nguyen13). These changes affect both EE (metabolic and behavioural changes) and energy intake (EI; behaviour) and have been mathematically modelled(Reference Heymsfield, Thomas and Nguyen13,Reference Heymsfield, Gonzalez and Shen14,Reference Thomas, Schoeller and Redman17–Reference Hall, Sanghvi and Göbel22) . However, more is currently known about some components of EE than EI and more is known about physiology than behaviour.
Energy expenditure
As WL proceeds energy requirements decrease in line with a decrease in metabolically active tissues and to some extent beyond that predicted by changes in metabolically active tissue, indicating some degree of metabolic adaptation(Reference Hall26,Reference Hall27) . Such changes are most evident in extreme WL. There is limited data on the effect of extreme energy deficits on change in body weight, composition and EE (and its components). The seminal Minnesota semi-starvation study examined the effect of 24-weeks semi-starvation on total EE and (by calculation) its components. The effects of a 40 % WL in initially lean men (comprised 70 % fat loss; 18–20 % FFM loss) on both EE and subsequent EI were amongst the most dramatic compensatory changes in EB components ever recorded(Reference Keys, Brožek and Henschel28).
During prolonged energy deficits (i.e. phase 2 of WL) most weight that is lost is in the form of reduced FM but FFM also decreases with WL, depending on initial body composition and the rate of WL(Reference Hall25). Decreases in weight and FFM also reduce spontaneous (volitional) physical activity EE(Reference Keys, Brožek and Henschel28,Reference Redman, Heilbronn and Martin29) , RMR and total daily EE(Reference Redman, Heilbronn and Martin29,Reference Leibel, Rosenbaum and Hirsch30–Reference Schwartz, Kuk and Lamothe32) , potentially increase muscular efficiency(Reference Goldsmith, Joanisse and Gallagher33) and decrease the energy cost of weight-bearing activities(Reference Redman, Heilbronn and Martin29). The adaptations may persist for 12 months or longer after WL(Reference Fothergill, Guo and Howard34,Reference Rosenbaum, Hirsch and Gallagher35) . These effects have been found in obese(Reference Fothergill, Guo and Howard34,Reference Rosenbaum, Hirsch and Gallagher35) and slightly overweight subjects losing 10–13 % of their weight(Reference Redman, Heilbronn and Martin29,Reference Martin, Heilbronn and de Jonge31) . The estimated maximum change of RMR/kg FFM under such conditions is about 5–10 %(Reference Westerterp36). Extreme energy deficits in the Minnesota study led to a 45 % drop in estimated total daily EE (Table 1) and in the Biggest Loser study reductions of total daily EE of 21 % were estimated by the end of the 30-week competition(Reference Fothergill, Guo and Howard34). In the latter study 37·9 % loss of an initial mean body weight of 149·2 kg, induced by energy restriction and very large increases in physical activity, led to a reduction of RMR independent of changes in body composition of 2109 kJ/d (504 kcal/d)(Reference Johannsen, Knuth and Huizenga37). Interestingly, a near doubling of physical activity (kJ/kg/d) was insufficient to offset the substantial decrease in total daily EE due to changes in body size and metabolic adaptation(Reference Fothergill, Guo and Howard34,Reference Johannsen, Knuth and Huizenga37,Reference Hall38) . The estimated change in whole body EE under conditions of 10–20 % therapeutic WL appears to be in the region of about 15 % or about 126 kJ/kg weight lost/d (30 kcal/kg weight lost/d)(Reference Polidori, Sanghvi and Seeley21,Reference Leibel, Rosenbaum and Hirsch30) . Thus, both physiological and behavioural components of EE significantly change in response to moderate or extreme, prolonged energy deficits in a way that tends to oppose further WL.
Table 1. Estimated changes in total energy expenditure and its components in response to prolonged semi-starvation in the Minnesota study.

The decrease in basal metabolic rate was 65 % due to change in metabolically active tissues and 35 % due to metabolic adaptation. The decrease in physical activity was 36 % due to the energy cost of physical activity and 64 % due to a change in physical activity behaviour. The total estimated decrease in daily energy expenditure after 6 months semi-starvation was 45 % of baseline daily energy expenditure.
Appetite and energy intake
While EE and its components change in response to energy imbalances in a quantitatively important manner, it is likely that compensatory changes in EI have a greater capacity to produce relatively large alterations in EB and body composition. Indeed, much of weight regain subsequent to WL is due to increases in EI rather than decreases in EE(Reference Thomas, Martin and Redman19,Reference Polidori, Sanghvi and Seeley21) .
In order to understand responses to therapeutic WL, extreme examples of semi-starvation provide exaggerated responses in which physiological mechanisms and behaviour changes can be more clearly observed. During the Minnesota study thirty-two lean men (mean per cent body fat 22 %) were underfed by about 40 % relative to their normal EI for 24 weeks. For the next 12 weeks they were incrementally refed, but by the end of this period they were still in a deficit of about 25 % for FM and 12–15 % for FFM. During the final 8 weeks, twelve subjects had ad libitum access to a range of foods, with EI initially increasing to 160 % of requirements and gradually subsiding to pre-WL levels. The cessation of post-WL hyperphagia coincided with a massive overshoot of FM, and repletion of FFM to baseline levels(Reference Keys, Brožek and Henschel28). These (limited) data suggest that there is an integrated response from FM and FFM that is associated with hyperphagia, subsequent to semi-starvation.
Very high levels of ad libitum EI in response to prior semi-starvation have been reported by Widdowson in twelve German men in Wuppertal immediately after the Second World War. These subjects (mean weight 59·3 kg) consumed about 25104 kJ/d (6000 kcal/d) over an observation period of 8 weeks, in which they had unlimited access to food, gaining 10·3 kg in weight(Reference Widdowson39). Similar levels of hyperphagia have been reported in a study of concentration camp (Sandbostel) prisoners, who consumed about 33472 kJ/d (8000 kcal/d)(Reference Murray40) and increased their weight from 56 to 61 kg over 22 d. In both studies, the rate of weight gain decelerated as the observation period proceeded.
It is important to question whether such compensatory physiological and behavioural changes are relevant to therapeutic WL in initially overweight or obese people. The work by Leibel et al., Redman et al. and Muller et al. suggest that for EE this is the case(Reference Hall27,Reference Redman, Heilbronn and Martin29–Reference Schwartz, Kuk and Lamothe32,Reference Fothergill, Guo and Howard34,Reference Rosenbaum, Hirsch and Gallagher35,Reference Müller, Enderle and Pourhassan41) . Energy deficits under conditions relevant to therapeutic WL also affect appetite control. Doucet and Cameron document how 25 % energy restriction typical of WL programmes leads to a substantial elevation of appetite over 15 weeks(Reference Doucet and Cameron42). Sumithran et al. have shown that a 13·5 kg (14 %) WL in fifty obese subjects through very low-energy diet led to an elevation in subjective appetite during a standardised test-meal protocol, immediately after WL and after 12 months where WL was maintained at 8·2 %. Thus, WL leads to increased hunger under standardised test-meal conditions 12 months later(Reference Sumithran, Prendergast and Delbridge43). Nymo et al. also showed an increase in hunger over 12 months after 15 % WL induced by a very low-energy diet. Interestingly, this was accompanied by an increase in fullness, which may be due to gastric contraction(Reference Nymo, Coutinho and Eknes44). Anton et al. found an increase in subjective sensations of hunger or appetite in the fasted state in the first 2 months of energy restriction, which then stabilised between 3 and 6 months in the CALERIE study(Reference Anton, Han and York45). Increases in motivation to eat in response to WL are not always apparent e.g.(Reference Andriessen, Christensen and Nielsen46). A review by Hintze et al. argues that on balance, the literature (primarily in women) suggests that WL (i) tends to elevate fasting and post-prandial subjective/self-reported appetite, (ii) alters appetite-related peptides in a manner that can be interpreted as broadly consistent with a WL-induced elevation of appetite and (iii) shows some evidence of increased food cravings and hedonic responsivity (liking and wanting)(Reference Hintze, Mahmoodianfard and Auguste47). Recently, Polidori et al. used a previously validated mathematical model(Reference Sanghvi, Redman and Martin48) to estimate long-term changes in EI from body weight (assuming no change in physical activity EE) during a year-long placebo-controlled trial in 153 people treated with Canagliflozin, a sodium glucose transport inhibitor that operates peripherally to increase urinary glucose production. Canagliflozin increases urinary glucose output by about 90 g/d (producing an energy deficit equivalent to about 1506 kJ/d (360 kcal/d), that was independent of EE, central appetite control mechanisms or cognition and therefore represented a truly covert energy deficit(Reference Polidori, Sanghvi and Seeley21). The study found that EI compensation (about 418 kJ/kg/d/kg of WL (100 kcal/kg/d/kg of WL)) was proportionate to WL and appears to be about three times greater than estimated compensation of estimated EE in response to 10–20 % WL (about 126 kJ/kg/d (30 kcal/kg/d))(Reference Leibel, Rosenbaum and Hirsch30). This study and the modelling of responses to WL pharmacotherapy(Reference Hall, Sanghvi and Göbel22) suggests that prolonged WL attempts lead to a prolonged and exponential increase in appetite (using estimated EI as a proxy) in proportion to the weight that is lost(Reference Polidori, Sanghvi and Seeley21). Reports of changes in peripheral appetite peptides in response to 10–20 % WL produce results that can be interpreted as being consistent with WL increasing homeostatic increases in appetite(Reference Sumithran, Prendergast and Delbridge43,Reference Nymo, Coutinho and Eknes44,Reference Hintze, Mahmoodianfard and Auguste47,Reference Iepsen, Lundgren and Holst49,Reference Sainsbury, Wood and Seimon50) .
WL influences body structure, which in turn affects EB physiology (the composition of tissues mobilised and EE) and behaviours (physical activity and EI), in a way that attempts to restore body weight to pre-WL levels. Extreme WL leads to pronounced decreases in components of EE and increases in appetite and EI that tend to ameliorate as body weight and composition are restored. Similar responses occur in relation to therapeutic WL, but they are more muted. Appetitive responses to therapeutic WL are not always easy to detect using psychometric or physiological measures at fixed timepoints, probably because participants already have an excess FM (which acts as an energy buffer) and because the rate and extent of WL are less pronounced. Nevertheless, mathematical modelling of changes in EI tracked over weeks and months suggest that there are compensatory increases in EI contribute substantially to weight regain.
Physiological and behavioural responses to weight loss: implications for weight management
WL invokes physiological changes on the intake and expenditure side of the EB equation that predispose those engaged in WL attempts to weight regain. The National Institutes of Health working group on maintenance of WL has produced a conceptual framework to articulate how such changes undermine longer-term WLM through changes in (i) appetite and eating behaviour, (ii) components of EE and (iii) the motivational cost of adherence to weight management programmes v. the rewards of continued WL attempts(Reference MacLean, Wing and Davidson51). These changes tend to oppose or undermine sustained behavioural attempts at reducing EI and increasing EE. This is the physiological challenge for behavioural interventions aimed at achieving WLM, subsequent to WL attempts. As weight is progressively lost evidence suggests that physiological signalling systems actively oppose further WL through compensatory decreases in activity behaviours and increases in appetite and EI(Reference Polidori, Sanghvi and Seeley21,Reference Keys, Brožek and Henschel28,Reference Leibel, Rosenbaum and Hirsch30,Reference Schwartz, Kuk and Lamothe32,Reference Rosenbaum, Hirsch and Gallagher35,Reference Hintze, Mahmoodianfard and Auguste47,Reference MacLean, Wing and Davidson51–Reference Greenway53) . It appears that the strength of such signalling systems increases on going from the modest amounts typically seen during voluntary WL to the large amounts characteristic of semi-starvation(Reference Stubbs, Hopkins and Finlayson8,Reference Keys, Brožek and Henschel28,Reference Widdowson39,Reference Murray40) . Thus, while WL is achievable in the short-to-medium term, there is a need to measure and understand the physiological resistance to WL and its impact on compensatory EB behaviours. This may inform behavioural strategies to prevent weight regain in the longer-term. However, EB behaviours are notoriously difficult to measure and the use of self-report dietary intake and physical activity estimates have been heavily criticised as methodologically flawed(Reference Dhurandhar, Schoeller and Brown54). Almost all dietary intake and many physical activity measures have a large subjective component; they are self-reported by the subjects. A consensus statement of the Energy Balance Assessment Working Group highlights the flawed nature of self-report dietary intake methods and that the potential inaccuracies of self-report data make findings in many studies questionable, incorrect or misleading(Reference Dhurandhar, Schoeller and Brown54). One answer to this problem will be to develop tracking technologies for estimation of EE from physical activity and energy storage from the tracking of body weight over time, so that mathematical models can be refined to resolve the EB equation, enabling errors and uncertainty associated with self-report measures of EB behaviours to be appreciated.
While relatively few plausible physiological systems have been identified that may protect individuals from overconsumption, the asymmetry of EB regulation is a major factor that should be considered when designing behavioural interventions for longer-term weight management (i.e. WL and prevention of weight regain). This might seem like an obvious notion, but it is apparent that the majority of longer-term WL interventions decrease in intensity as the intervention progresses (i.e. greater intervention intensity is focused on the WL rather than the WLM phase). However, the progression of a weight control intervention is associated with decreased adherence, a lapse in the control of EB behaviours, increased dropout and hence weight regain, and as such it is more logical for intervention dose to be maintained or increase, rather than decrease, over time. Evidence supports an extended care approach, in which obesity is treated as a chronic disease requiring continuous therapist contact to prevent weight regain(Reference Ross Middleton, Patidar and Perri55). It is perhaps useful to consider the challenges that the physiology of EB regulation presents for behaviour change interventions for WL and WLM.
In simplistic terms the key challenges for longer-term weight management involve (i) engaging a significant proportion of the population in evidence-based behavioural approaches to WL, (ii) adapting behaviour change attempts to navigate around the physiological resistance to WL that undermines maintenance of WL, (iii) providing behavioural solutions to facilitate coping with lapses in the control of EB behaviours and relapses in weight and (iv) finding methods to scale evidence-based solutions to longer-term weight management across the general population. These challenges are individually considerable and collectively daunting. The behavioural evidence for longer-term weight management is less robust than one might expect. The quantification of EB behaviours is inexact, making lapses difficult to detect. Several weight management solutions are scaled across the general population, with limited effect and (excluding drugs and surgery) the mechanisms of action remain unclear.
The effectiveness of behaviour change interventions for weight management
Weight loss
There is now considerable evidence documenting the effects of non-commercial and commercial behavioural, pharmacological or surgical interventions on initial WL(Reference Gudzune, Doshi and Mehta4,Reference Vakil, Chaudhry and Doshi5,Reference Johnston, Kanters and Bandayrel11,Reference Anton, Han and York45,Reference Franz, VanWormer and Crain56–Reference Vakil, Doshi and Mehta66) . Diet and lifestyle interventions produce mean WL <5 kg after 2–4 years, which is disappointing compared to pharmacological therapies (mean WL 5–10 kg over 1–2 years) and surgery (mean WL 25–75 kg after 2–4 years)(Reference Douketis, Macie and Thabane67). All of these approaches are subject to some degree of longer-term weight regain(Reference Franz, VanWormer and Crain56,Reference Magro, Geloneze and Delfini68) .
Behavioural programmes have central elements of dietary restriction, behavioural counselling/support and physical activity advice (see later)(Reference Loveman, Frampton and Shepherd69). But programmes vary in modes of delivery, settings and implementation strategies(Reference Jensen, Ryan and Apovian70). Macronutrient composition of the diet seems relatively unimportant in terms of its impact on WL(Reference Johnston, Kanters and Bandayrel11,Reference Jensen, Ryan and Apovian70) . A lower energy dense diet may be more efficacious for appetite control(Reference Buckland, Camidge and Croden71,Reference Ello-Martin, Roe and Ledikwe72) , which may improve adherence during WL attempts. Evidence that high fibre or high protein diets may enhance satiety is inconsistent(Reference Benelam73,Reference Slavin and Green74) . Whether these are nutrient-specific effects that are completely independent of energy density is less clear(Reference Benelam73).
Many evaluations of WL programmes now involve 1-year follow up(Reference Gudzune, Doshi and Mehta4,Reference Johnston, Kanters and Bandayrel11,Reference Franz, VanWormer and Crain56,Reference Jolly, Lewis and Beach59,Reference Douketis, Macie and Thabane67) . Many trials of such programmes are characterised by high attrition, relatively short duration, lack of blinding and tend to report best-case scenarios (e.g. completer analyses or last observation carried forward analyses)(Reference Gudzune, Doshi and Mehta4). For completers of non-surgical WL clinical trials mean WL of 5–9 % occur, plateau at about 6–12 months and gradually creep upwards between 24 and 48 months (where data are available)(Reference Franz, VanWormer and Crain56). In real-life settings a greater number of potential participants will not actually engage with the programme and outcomes may be more variable. Commercial programmes tend to produce slightly but significantly greater WL than non-commercial (e.g. primary care setting) programmes(Reference Jolly, Lewis and Beach59). Performance of commercial weight management programmes is broadly comparable (Reference Johnston, Kanters and Bandayrel11,Reference Vakil, Doshi and Mehta66) . In the USA few community-based programmes meet the American College of Cardiology/American Heart Association Task Force on Practice Guidelines and The Obesity Society guideline for the management of overweight and obesity in adults(Reference Vakil, Doshi and Mehta66). Very low-energy diets induce faster and greater WL but also faster and greater weight regain(Reference Gudzune, Doshi and Mehta4,Reference Franz, VanWormer and Crain56) . Several analyses suggest that initial WL and programme attendance are important predictors of weight outcomes(Reference Astrup and Rössner75–Reference Stotland and Larocque79). However, it seems that in real-life programmes (rather than clinical trials) average attendance is limited to 7–8 weeks and over a 12-month time window the vast majority of participants (>90 %) drop out of such programmes(Reference Martin, Talamini and Johnson80). Thus, for many, these attempts are short in duration (days–weeks) and result in cessation of the behaviour changes that initiated the WL attempt and/or lead to subsequent weight regain.
Weight loss maintenance
There is debate as to whether WLM represents a separate behavioural phase to WL, requiring a different skills-set or whether it involves a continuation of the skills developed for WL in the first place(Reference Stubbs and Lavin81). There is evidence that some of the behaviours that lead to WL are continued during WLM, and that some additional behaviours are recruited during the period of WLM(Reference Sciamanna, Kiernan and Rolls82). For this reason, it is useful to consider longer-term weight control as a dynamic interaction between behavioural strategies to lose weight, maintain that loss and the physiological (and environmental) resistance to WL, which creates a tonic pull on EB behaviours that can undermine the behaviours that achieved initial WL. It is more appropriate to view the transition from WL to WLM as a dynamic process. Those who lose weight are at high risk of weight regain. Given the limited effectiveness of diet and lifestyle programmes for WL most people who have engaged in an initial WL attempt are actually aiming to achieve further WL rather than WLM. Many people attempting to maintain their WL therefore experience periods where they re-visit strategies they originally used to lose weight, in order to cope with weight relapse or lose further weight. In this sense, the study of WLM is something of a misnomer and would be better described as the study of weight regain prevention.
Studies aimed specifically at supporting WLM or longer-term weight management after a period of clinically significant WL are relatively few and have been systematically reviewed by Middleton, Ddouketis, Curioni, Neve and Ramage(Reference Ross Middleton, Patidar and Perri55,Reference Douketis, Macie and Thabane67,Reference Curioni and Lourenco83–Reference Ramage, Farmer and Apps Eccles85) and more recently and extensively by Dombrowski et al.(Reference Dombrowski, Knittle and Avenell86) and Varkevisser(Reference Varkevisser, van Stralen and Kroeze87). The majority of such studies usually involve a continuation of interventions that are used for initial WL i.e. diet/lifestyle interventions (primarily diet) and/or use of pharmacological treatments (e.g. orlistat). While the majority of interventions aimed at producing or maintaining WL are, by design, complex, evidence of their specific behavioural mechanisms of action is limited. Almost all successful WL interventions reviewed by Ramage et al. combined elements of dietary restriction, physical activity advice and behaviour change approaches to support alterations in EB behaviours(Reference Ramage, Farmer and Apps Eccles85). These involved standard or cognitive behavioural therapy, education on behavioural strategies delivered by a behavioural practitioner and self-monitoring (including self-weighing, social support, goal setting, stimulus control, relapse prevention strategies, problem-solving and promoting intrinsic motivation). Successful WLM appears to involve a continuation of some or all of these strategies but it is unclear what differentiates those who maintain from those who regain.
While those who adhere to evidence-based weight management programmes can achieve significant WL, they appear to be the minority of those who initially engage in such programmes. For many, initial engagement in weight management programmes occurs in the backdrop of an obesogenic environment, which is relatively hostile to sustained WL attempts and presents risks for relapse. Many individuals experience frustrations and lapses, adherence to initial behaviour change becomes sporadic, weight control behaviours discontinue leading to weight relapse and regain. Repeated failed attempts at WL may decrease motivation, physical and emotional well-being (Fig. 2).
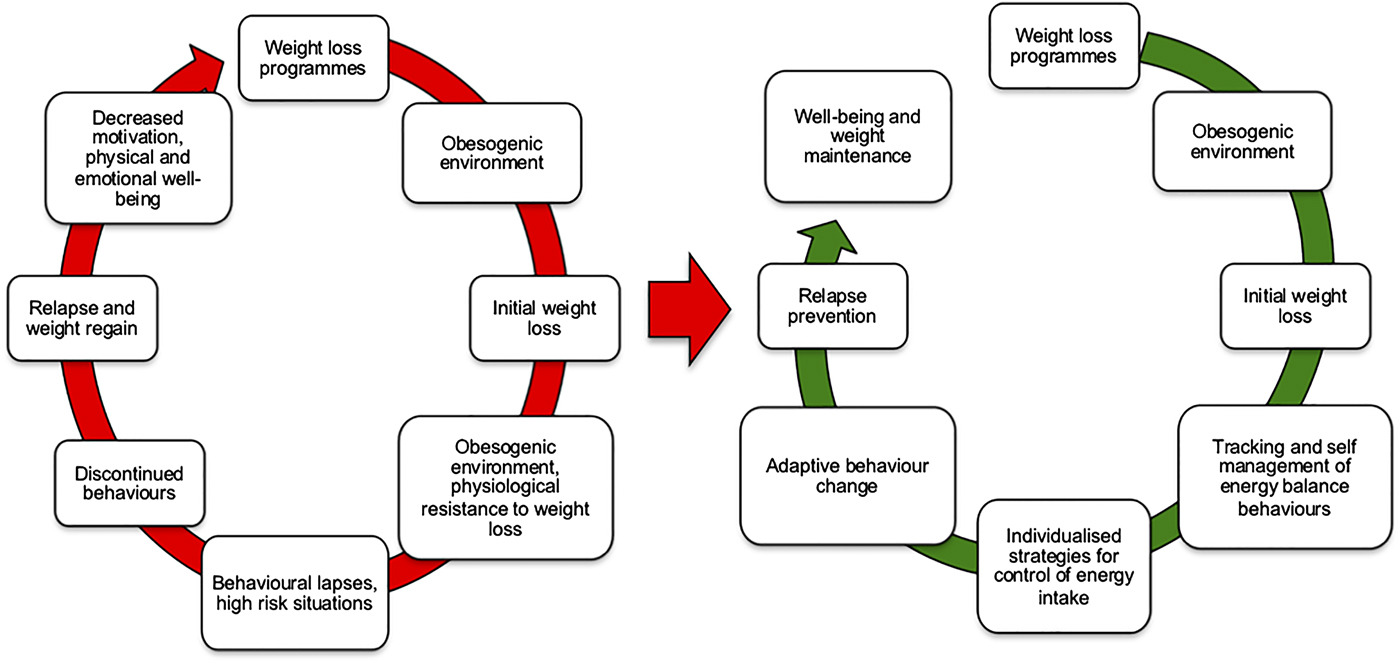
Fig. 2. (Colour online) The cycle of weight loss and weight relapse. The left side indicates the pathways by which weight loss attempts are opposed by both an obesogenic environment and physiological resistance to weight loss. The right side illustrates how better matching behaviour change approaches to tracking of energy balance behaviours may facilitate rapid monitoring and adjustments of energy intake to help avoid weight regain.
Behavioural mechanisms of weight loss maintenance interventions
Behaviour change approaches for WL and WLM should be both theoretically informed and evidence-based in order to understand and target effective intervention components to the needs of specific individuals(Reference Dombrowski, Sniehotta and Avenell88–Reference Michie, Richardson and Johnston91). There is currently more theory than clear evidence. It is unclear what specific behaviour change approaches, modes of delivery, settings and implementation strategies are most effective for sustained change in EB behaviours. Behaviour change approaches for WLM might be undermined by compensatory changes in EE and EI as WL proceeds. This field is still poorly developed because the majority of studies examining predictors of longer-term weight outcomes have either used pre-treatment predictors(Reference Carraça, Santos and Mata92,Reference Teixeira, Going and Houtkooper93) or correlates of WL and maintenance, rather than sequential measures of outcomes throughout the intervention and follow-up periods. Few/no studies have directly linked longitudinal changes in compensatory EB physiology and behaviour to mechanisms of action of behaviour change interventions. The current discussion focuses on the process of WLM given that pre-treatment predictors of WL tend to explain relatively little of subsequent weight outcomes(Reference Varkevisser, van Stralen and Kroeze87,Reference Carraça, Santos and Mata92,Reference Teixeira, Going and Houtkooper93) and WLM studies appear to focus on self-selected, non-representative samples(Reference Butryn, Phelan and Hill94–Reference Thomas, Bond and Phelan97).
The majority of systematic reviews of WLM interventions find considerable heterogeneity between studies(Reference Dombrowski, Knittle and Avenell86,Reference Varkevisser, van Stralen and Kroeze87,Reference Teixeira, Carraça and Marques98) . Many determinants of WLM are established by association rather than longitudinal change scores. Studies use different theoretical frameworks, constructs and measures(Reference Varkevisser, van Stralen and Kroeze87). A significant proportion report completer analyses e.g.(Reference Franz, VanWormer and Crain56). For many specific predictors of long-term weight outcomes, the evidence is insufficient to reach a strong conclusion(Reference Varkevisser, van Stralen and Kroeze87,Reference Dombrowski, Sniehotta and Avenell88,Reference Teixeira, Carraça and Marques98) . In the past few years evidence that self-regulation of weight control and EB behaviours improves longer-term weight outcomes has grown. Dombrowski et al. have found in forty-five studies that behavioural interventions targeting both diet and physical activity behaviours for WLM are moderately effective (about 1·6 kg difference compared to control/placebo interventions) at promoting WLM over 1 year. There is some evidence of effectiveness over 2 years, and limited evidence relating to weight outcomes beyond 2 years(Reference Varkevisser, van Stralen and Kroeze87,Reference Dombrowski, Sniehotta and Avenell88,Reference Teixeira, Carraça and Marques98) . They found no evidence that mode of intervention delivery (number of intervention components or frequency of contact, internet v. control or face-to-face v. remote delivery of the same intervention), for diet, physical activity or nutritional supplements/food replacements have a greater effect when used as interventions alone. Teixeira et al.(Reference Teixeira, Carraça and Marques98) have systematically reviewed forty-two putative self-regulatory and psychological mechanisms as mediators of longer-term weight outcomes and EB behaviours across thirty-five behavioural interventions. They identified mediators of successful weight outcomes as higher autonomous motivation, self-efficacy/barriers, self-regulatory techniques, flexible restraint and positive body image. Mediators of sustained increases in physical activity were autonomous motivation, self-efficacy and use of self-regulatory skills. No mediators of long-term dietary intake were identified. In this analysis, weight change was empirically measured in twenty-six studies, but physical activity was objectively measured in only four of nineteen studies and dietary intake was objectively measured in none of the eleven studies examining mediators/predictors of dietary intake. Varkevisser et al.(Reference Varkevisser, van Stralen and Kroeze87) have recently systematically reviewed forty-nine studies and evaluated five demographic, fifty-nine behavioural, fifty-one psychological/cognitive and nine social and environmental determinants of weight outcomes in observational, long-term WL and maintenance interventions. They found that aspects of self-regulation of eating, activity and weight control behaviours are effective for WLM, through their impact on change in behaviour during weight management attempts. This is important because pre-treatment predictors explain very little of the variance in WL(Reference Varkevisser, van Stralen and Kroeze87,Reference Carraça, Santos and Mata92,Reference Teixeira, Going and Houtkooper93) .
The limited evidence from these meta-analyses suggests that navigating from initial WL to habitual WLM requires long-term self-management of EB behaviours in the face of physiological resistance to WL. Avoiding slow, gradual and the more pronounced weight regains require behavioural strategies in which relapse coping and WLM become learned skills of self-regulation, action planning, developing self-efficacy, autonomy and motivation(Reference Dombrowski, Avenell and Sniehott99–Reference Larimer, Palmer and Marlatt104) as part of a longer-term process. Core features of modestly more effective WLM interventions include behaviour change techniques that improve self-efficacy in self-monitoring (of weight and behaviour), relapse prevention, goal setting, and action plans for diet and physical activity(Reference Stubbs and Lavin81,Reference Dombrowski, Knittle and Avenell86,Reference Varkevisser, van Stralen and Kroeze87,Reference Michie, Abraham and Whittington90,Reference Teixeira, Carraça and Marques98,Reference Dombrowski, Avenell and Sniehott99,Reference Silva, Markland and Carraça105) . Autonomous self-regulation and intrinsic motivation may augment self-regulatory goals and self-efficacy(Reference Carraça, Santos and Mata92,Reference Teixeira, Mata and Williams101,Reference Silva, Markland and Carraça105,Reference Verstuyf, Patrick and Vansteenkiste106) . However, physical activity and dietary interventions based on current behaviour change theories characteristically achieve relatively small effects on weight outcomes, in the region of about 1·5 kg compared to controls. This is disappointing and there is considerable interest in improving the effectiveness of such interventions. This could be achieved by greater standardisation of predictive constructs and outcome measures that are tracked longitudinally to improve cause–effect models, by providing more accurate objective tracking of EB behaviours, to facilitate self-monitoring and improve analyses of the mechanism of action of behaviour change interventions for weight management. This, in turn, may lead to personalised intervention content and delivery to meet individual needs and improve weight outcomes.
Historically, behaviour change models have focused on social cognition (e.g. beliefs, intentions, attitudes and decisions), emphasising pathways of reasoned action in which pre-decisional motivation leads to the formation of intentions and the implementation of intentions as volitional action(Reference Armitage and Conner107,Reference Sniehotta108) . It is also believed that automatic processes (emotions, desires, habits resulting from associative learning and physiological states) may also have a large impact on behaviour and behaviour change. These processes tend to be relatively rapid, impulsive (less conscious) and habitual in comparison to the slow, deliberative processes of motivation and self-regulation(Reference Gibbons, Houlihan and Gerrard109,Reference Hofmann, Friese and Strack110) . Furthermore, in the context EB behaviours, the development of self-regulatory behaviour change is effortful, particularly in the face of physiological resistance to WL, while unconscious or automatic components of EB behaviours are rapid and effortless(Reference Bargh and Chartrand111). Such processes may have considerable capacity to undermine initial self-regulation of EB behaviours (particularly eating behaviours) in the face of a physiological system that resists longer-term WL(Reference Bargh and Chartrand111). Physiological mediators of homeostatic and hedonic appetitive drives and changes in physical activity that are triggered by WL may feed into such automatic process of behaviour change to undermine self-regulation of EB behaviours. These concepts are depicted in Fig. 3, which is an adaptation of Witkiewitz and Marlett's relapse model(Reference Witkiewitz and Marlatt112). Automatic components of self-regulation may also promote longer-term behaviour change if they are engaged and developed.

Fig. 3. (Colour online) An adaptation of Witkiewitz and Marlett's relapse prevention model(Reference Witkiewitz and Marlatt112) focusing on the factors that influence the relaxation of controlled eating behaviour during a weight loss attempt. Energy intake appears to be a quantitatively more important route for weight regain and is likely to be the most promising target for prevention of weight relapse during weight loss attempts, in the context of tracking energy balance and its components.
Another aspect of automaticity potentially affecting EB behaviours is distress tolerance and emotion regulation. Individuals trying to lose weight can experience increased psychosocial stress and weight-related stigma(Reference Carr and Friedman113–Reference Puhl and Brownell115), which may undermine self-regulatory practices and WL attempts. Repeated attempts at WL followed by weight regain can have a negative emotional impact, leading to self-critical thoughts and negative emotions. For some, ‘comfort eating’ may be a means of coping with these negative experiences, potentially derailing strategies of planned behaviour(Reference Adam and Epel116–Reference Vartanian and Porter120). Contextual–behavioural approaches to WL and WLM suggest that specific skills could be developed to promote self-regulation and to prevent relapse(Reference Carrière, Khoury and Günak121). These include mindfulness and cognitive awareness of automaticity, behavioural commitment with personally-relevant values and acceptance of internal negative or aversive states (e.g. negative emotions or cognitions)(Reference Carrière, Khoury and Günak121–Reference Lillis and Kendra124). Mindfulness and acceptance-based interventions, which are one attempt to address difficulties in emotion regulation, show modest promise in changing obesity-related eating behaviours (e.g. binge eating and emotional eating) and improving weight management(Reference Carrière, Khoury and Günak121). Augmenting current self-regulatory approaches with components that help manage weight-related emotions could potentially lead to more effective WLM.
Developing more effective, personalised interventions for longer-term weight management
There is some evidence that aspects of self-regulation and motivation may improve the odds of sustaining changes in EB behaviours and if those changes become automatic in the longer-term the chances of preventing weight regain may improve. However, it is likely that automatic processes (emotions, desires, appetitive drives and habits resulting from associative learning and physiological resistance to WL) are powerful forces that can undermine the relatively transient and fragile attempts at changing EB behaviours during phase 1 and 2 of WL. It is perhaps in this dynamic transition where we need to better understand the interplay between physiology and behaviour to improve longer-term weight management and the prevention of weight regain. Key areas where there is a need to develop new evidence to inform behaviour change interventions are detailed later.
There is an urgent need to understand the relationship between WL-induced EB physiology and compensatory changes in EB behaviour that appear to collectively drive weight regain. At present this endeavour is hampered by the fact that EB behaviours are extremely difficult to measure. Measurement of EI and EE in free-living subjects (with the exception of doubly-labelled water) usually relies on self-report measures that are known to be unreliable(Reference Dhurandhar, Schoeller and Brown54). Furthermore, it is likely that significant components of EB behaviours are automatic and therefore extremely difficult to measure using self-report methods. Given the apparent unreliability of self-report measures of EI and EE, the development of objective tracking technologies would enable better quantification of EI and EE. Numerous tracking devices are available to estimate physical activity but the relationship between such measurements and estimates of EE is uncertain(Reference O'Driscoll, Turicchi and Beaulieu125). Such devices have the advantage of being cost-effective, objective, unobtrusive and capable of considerable data storage and aggregation. However, such devices are limited by their lack of accuracy(Reference O'Driscoll, Turicchi and Beaulieu125). It is important to bridge the gap between the tracking of physical activity and estimates of free-living EE. Accurate tracking of EE and body weight would allow approximate estimates of changes in EB and its components. Such developments would provide a major leap forward in longitudinal estimates of EB behaviours and their relative contribution to altered states of EB. Such methodological developments would potentially provide the quantitative framework on which behaviour change interventions for longer-term self-management of body weight could be based. Objective quantification of EI and EE would help us understand and better use self-reported psychological and behavioural factors. Longer-term weight management interventions should be designed around the known compensatory physiological responses to WL and developing approaches to tracking EB behaviours is a critical gap that needs to be addressed in improving future interventions.
There is currently a gap between detailed, small-scale physiological studies of WL and the larger-scale interventions that seek to understand mechanisms of action of behaviour change approaches(Reference MacLean, Wing and Davidson51). Such behavioural studies tend to ignore both physiological resistance to WL and compensation of EB behaviours. It is important to improve our understanding of the mechanisms by which WL facilitates subsequent weight regain as a context in which behaviour change interventions attempt to operate. The use of mathematical modelling represents a major advance in estimating the contribution of EI and EE to longer-term weight outcomes(Reference Thomas, Schoeller and Redman17–Reference Thomas, Martin and Redman19,Reference Polidori, Sanghvi and Seeley21,Reference Hall, Sanghvi and Göbel22,Reference Hall27) . Current models assume a level of physical activity EE and estimate change in body energy stores from changes in body weight or composition. If two of the three components of the EB equation and known (change in EE and energy stores) the third (EI) can be estimated by difference. These models could be refined if mobile tracking technologies can improve estimates of free-living EE.
There is a need to develop longitudinal interventions using logic models based on theoretically informed, evidence-based intervention content, employing repeat measures of components of EB (e.g. physical activity, weight) tracked over the course of WL attempts, so that cause–effect relationship can be established to better understand the mechanisms of action of behaviour change interventions. Such interventions should also, where possible, examine user interactions with intervention content. Given the evidence reviewed earlier it is likely that such secondary analyses will yield critical information about who responds to certain intervention components and how those components affect EB behaviours, to inform personalised interventions in the future. If the typical effect sizes produced by multi-component interventions are small, personalisation of such interventions, by better matching evidence-based behaviour change content to the specific needs of individuals may improve longer-term weight outcomes.
Greater standardisation of predictive constructs and outcome measures, in clearly defined study populations, tracked longitudinally, would improve cause-effect models that characterise (i) how WL impacts on body structure, function and subsequent EB behaviours, (ii) how behaviour change approaches can overcome physiological resistance to WL and (ii) who is likely to maintain WL or relapse (Fig. 4). Modelling physiological and psychological moderators and mediators of EB-related behaviours is central to understanding and improving longer-term weight and health outcomes in the general population. Improved measurement of EB and associated behaviours may help us bring together research on physiological and behavioural responses to energy deficits, better understand the factors that lead to weight regain and help people navigate more effectively to sustained changes in their weight and health outcomes.

Fig. 4. (Colour online) Illustration of how the National Institutes of Health conceptual framework(Reference MacLean, Wing and Davidson51) for weight loss maintenance can be adapted to develop cause–effect models that characterise how weight loss impacts on body structure, function and energy balance behaviours, how behaviour change approaches can mediate physiological resistance to weight loss and who is likely to maintain or regain weight lost. EE, energy expenditure; EI, energy intake.
Acknowledgements
The authors are grateful to the University of Leeds School of Psychology for matched funding of the PhD scholarships for Ruairi O'Driscoll and Jake Turicchi.
Financial Support
None.
Conflict of Interest
R. J. S. consults for Slimming World UK, through Consulting Leeds, a wholly-owned subsidiary of the University of Leeds. This consultancy played no role in the funding or production of the present paper. The authors have no conflict of interest to declare.
Authorship
All authors contributed to the writing and editing of the paper.