Immunoglobulins are a group of specialised bioactive proteins, present in blood plasma, serum and other tissue fluids, including the milk of all mammals(Reference Späth1). Numerous studies have reported benefits from including animal plasma that contains immunoglobulins in the post-weaning diet of production animals, with improvements observed in feed intake, growth rate and intestinal organ growth(Reference Pierce, Cromwell and Lindemann2, Reference Campbell, Polo and Russell3). The beneficial effect of animal plasma seems to be more pronounced in animals during a pathogen challenge(Reference Coffey and Cromwell4). Lallès et al. (Reference Lallès, Bosi and Janczyk5) reported that the beneficial effects of animal plasma were mediated mainly by its immunoglobulin fraction. The observed effects of the immunoglobulin fraction on growth in animals might be due to a greater availability of nutrients and energy for growth, consequent upon a lower degree of immune cell activation and an altered integrity and structure of the intestinal mucosa(Reference Fiocchi6).
Orally administered human immunoglobulins have been used as a prophylactic treatment for children born prematurely with necrotising enterocolitis(Reference Eibl, Wolf and Furnkranz7). Immunoglobulins have also been used therapeutically for medical intervention of Campylobacter jejuni (Reference Hammarström, Smith and Hammarström8), Clostridium difficile (Reference Tjellström, Stenhammar and Eriksson9), Helicobacter pylori, rotavirus and cryptosporidial infection. Reports have shown that orally administered immunoglobulins retain activity after digestion in the gastrointestinal tract(10). We recently demonstrated in a rat model that oral administration of purified intact ovine (extracted from lamb's blood) serum immunoglobulins (and not the heat-denatured fraction: autoclaved ovine serum immunoglobulins) was effective in improving growth performance(Reference Balan, Han and Rutherfurd11) and led to a higher degree of immunological activity as evidenced by increased phagocytic activity, lymphocyte proliferation, cytokine production and intestinal immunoglobulin levels(Reference Balan, Han and Rutherfurd-Markwick12). Feeding ovine serum immunoglobulins also resulted in an increase in gut mucin protein concentrations(Reference Balan, Han and Singh13) and supported the growth of beneficial lactic acid bacteria and prevented the growth of pathogenic bacteria in the gut (Balan(10); Balan et al. (Reference Balan, Han and Rutherfurd-Markwick14); P Balan, unpublished results) in both normal and Salmonella enteritidis-challenged rats(10, Reference Balan, Han and Rutherfurd-Markwick14). We have also demonstrated that immunoglobulins can survive gut digestion and that intact ovine immunoglobulins can be detected in the luminal contents, from the stomach through to the colon, of rats fed the ovine immunoglobulin diet(10). Pérez-Bosque et al. (Reference Pérez-Bosque, Miró and Polo15) reported that Staphylococcus aureus enterotoxin B (SEB) increased the release of pro-inflammatory mediators in the rat intestinal mucosa challenged with SEB. Animal plasma supplementation prevented the increase in pro-inflammatory mediators such as interferon-γ (IFNγ), IL-6 and leukotriene-B4 induced by SEB. They also reported that animal plasma supplementation increased anti-inflammatory mediators such as IL-10 and transforming growth factor-β concentrations in intestinal mucosa. These results suggest that immunoglobulin supplements can modulate the gut immune system (gut-associated lymphoid tissue) at the mucosal level during infection.
Our earlier reports have shown that ovine immunoglobulins modulated selected immune indices in serum and in the intestinal digesta of normal and pathogen-challenged rodents(10, Reference Balan, Han and Rutherfurd-Markwick12, Reference Balan, Han and Rutherfurd-Markwick14). We hypothesise that oral ovine immunoglobulin supplementation may prevent the release of mucosal pro-inflammatory mediators during intestinal challenge. Mucosal mediators such as cytokines, immunoglobulins and mucin are important physiological regulators of mucosal function. We therefore investigated the effects of orally administered ovine immunoglobulins on various indices of intestinal mucosal inflammation, such as the pro-inflammatory cytokines (IFNγ and TNFα), anti-inflammatory cytokines (IL-4 and IL-10), immunoglobulins (total IgA, total IgG, total IgE and anti-Salmonella (ASE) IgA, IgG), myeloperoxidase (MPO) activity and mucin in rats challenged with S. enteritidis. Comparison was made between rats fed a basal casein-based control diet containing no immunoglobulins (BD; unchallenged) and S. enteritidis-challenged rats fed either the BD or a casein-based diet supplemented with either freeze-dried ovine immunoglobulins (FDOI) or autoclaved freeze-dried ovine immunoglobulins (AOI). The latter constituted a negative control.
Materials and methods
Preparation and quantification of ovine serum immunoglobulins
An ovine immunoglobulin fraction was prepared by ammonium sulfate precipitation of fresh lamb's blood as described previously by Balan et al. (Reference Balan, Han and Rutherfurd11). The final product was freeze-dried and a portion was inactivated by autoclaving (121°C; 15 min) to produce the AOI. The inactivation of immunoglobulins in the AOI fraction was confirmed by Circular Dichroism (Chirascan™ Circular Dichroism Spectrophotometers; Applied Photophysics) based on the method of Vermeer & Norde(Reference Vermeer and Norde16). The IgG content of the ovine immunoglobulin fraction was determined by direct ELISA using a rabbit anti-sheep IgG (AbD Serotec) as described by Balan et al. (Reference Balan, Han and Rutherfurd11). The respective protein and IgG concentrations of the FDOI material were 94·0 and 73·0 %.
Experimental diets
Three semi-synthetic wheat starch-based diets were formulated: (1) a basal casein-based control diet containing no immunoglobulins (BD; control); (2) the basal casein-based diet supplemented with FDOI; and (3) the basal casein-based diet supplemented with AOI (negative control). The ingredient composition and determined nutrient composition of the diets are shown in Table 1. All diets were formulated to exceed the recommended nutrient requirements for the growing rat(17). The FDOI and AOI fractions were included in the respective diets at a concentration approximately equal to the amount of spray-dried porcine plasma IgG used in previous studies(Reference Pierce, Cromwell and Lindemann2, Reference Balan, Han and Rutherfurd11) where performance responses were observed.
Table 1 Ingredient compositions and determined nutrient and energy contents of the control and test diets
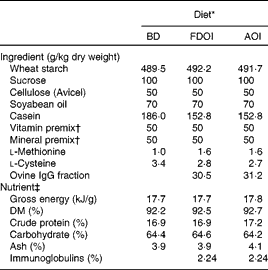
BD, basal diet; FDOI, freeze-dried ovine immunoglobulins; AOI, autoclaved ovine immunoglobulins.
* All diets were formulated to meet or exceed the nutrient requirements of the growing rat.
† The vitamin and mineral premixes were age-appropriate for the growing rat.
‡ Values for nutrients were determined except for carbohydrate, which was calculated by difference, and the immunoglobulin content, which was estimated based on the known levels of the FDOI.
Animal study
The present study was approved by the Massey University Animal Ethics Committee (MUAEC 09/29) and procedures complied with the New Zealand Code of Recommendations and Minimum Standards for the Care and Use of Animals for Scientific Purposes (New Zealand Animal Welfare Advisory Committee, 1995). A total of sixty Sprague–Dawley male rats (aged 8 weeks) were housed individually in stainless-steel cages at 22 ± 2°C with a 12 h light–12 h dark cycle in a single room under PC2 (physical containment level 2) conditions with high-efficiency particulate arresting (HEPA) filtered air, in the Small Animal Production Unit, Massey University, Palmerston North. The animals had unrestricted access to water at all times. During week 1, the rats were acclimatised to the cages and received the BD ad libitum. At the end of the acclimatisation period the rats were randomly allocated to the four experimental groups such that there were fifteen rats per group. The treatment and control groups were as follows: (1) non-challenged rats fed the basal control diet (BD); (2) rats challenged with S. enteritidis and fed the BD; (3) rats challenged with S. enteritidis and fed the FDOI-containing diet; and (4) rats challenged with S. enteritidis and fed the AOI-containing diet (negative control). The rats received the respective diets ad libitum for an 18 d experimental period. On day 15, the rats in groups 2, 3 and 4 were orally administered with a single dose of S. enteritidis ATCC 13 076 (1 ml of saline solution containing 3 % sodium bicarbonate with 1 × 107 viable S. enteritidis). S. enteritidis (strain 1891, originally from the UK) was purchased from the New Zealand Reference Culture Collection – Environmental Science and Research. Before use, S. enteritidis was subcultured three times in LB (Luria–Bertani) broth (Difco). The exact viable count of S. enteritidis inoculum was determined by plating on brilliant green agar (Oxoid). Rats in group 1 (unchallenged, BD) received 1 ml of saline solution without S. enteritidis.
Chemical analysis
Dietary DM, ash, crude protein, total fat and gross energy contents of the diet (Table 1) were determined according to methods described by AOAC(18). The carbohydrate fraction was determined by difference using the formula (100 − (moisture+ether extract+ash+crude protein) = carbohydrate).
Post-mortem procedure
On day 18 of the experiment, the rats were anaesthetised by intraperitoneal injection (0·1 ml/100 g live weight) of a mixture containing two parts acepromazine maleate BP (2 g/l), five parts ketamine (100 g/l), one part xylazine (100 g/l) and two parts sterile water. Rats were then killed by exsanguination. The entire small intestine from the pylorus to the ileo-caecal valve was removed, dissected free of mesenteric attachments, with the duodenum (upper 12 % from the pylorus end, not used in the present study) being isolated and with the rest being divided into two parts (equal in length), designated to approximate the jejunum (centre of the small intestine) and the ileum (anterior to the ileo-caecal valve). Deng et al. (Reference Deng, Cheng and Wang19) reported that the jejunum, ileum, caecum and colon are the primary sites of invasion in normal mice after oral infection with S. enteritidis. The entire jejunum and ileum were collected and washed intraluminally with PBS. The number of Peyer's patches was counted in the entire ileal segment and recorded. The same procedure was followed for the caecum and colon. The intestinal segments were cut longitudinally, and the mucosa scraped using glass slides. The mucus from each segment was suspended in 5 ml PBS containing 1 % protease inhibitor (Sigma) and centrifuged (HERAEUS, Fresco 17; Thermo Electron Corp.) at 5000 g for 30 min at 4°C to remove debris and the clear supernatant fraction was stored at − 80°C for further analysis. The mesenteric lymph nodes (MLN) and pancreas were also removed and weighed. The remaining portion of the animal was weighed as the empty body weight. Relative weights of the pancreas were expressed relative to empty body weight.
Analysis of mucosal cytokines, IgA, IgG, IgE, and Salmonella-specific mucosal IgA and IgG
The concentrations of pro-inflammatory cytokines (INFγ and TNFα), anti-inflammatory cytokines (IL-4 and IL-10), rat IgA, IgG, IgE, and Salmonella-specific IgA and IgG in the intestinal mucosa were measured by sandwich ELISA as previously described(Reference Balan, Han and Rutherfurd-Markwick14).
Determination of myeloperoxidase activity
MPO activity was measured as an indicator of neutrophil infiltration (intestinal inflammation) in intestinal mucosal samples using a Myeloperoxidase Colorimetric Activity Assay Kit (Biovision) according to the manufacturer's instructions. One unit of MPO is defined as the amount of MPO that hydrolyses the substrate and generates taurine chloramine to consume 1·0 μmol trinitrobenzene (TNB) per min at 25°C.
Quantitative analysis of mucin
Mucin concentrations in the intestinal mucosa were measured by an enzyme-linked lectin assay using wheat germ agglutinin (Sigma) as the lectin(Reference Balan, Han and Singh13, Reference Balan, Han and Rutherfurd20). The concentration of mucin in samples was calculated with reference to a porcine gastric mucin (Sigma) standard curve. Data were expressed as μg or mg mucin/l of supernatant fraction of mucosa suspended in a PBS solution.
Statistical analysis
For most variables there were fifteen rats per treatment, but in some cases a lower (n 8) sample size was used. Data were checked for normality and results expressed as mean values with their standard errors. Data were analysed using a one-way ANOVA using the General Linear Models procedure of SAS/PROC GLM (SAS version 9.1; SAS Institute Inc.) and means were compared using Tukey's test. Statistical significance was accepted at P< 0·05.
Results
The effect of administration of S. enteritidis to the rats was acute. Most of the rats from the BD- and AOI-supplemented diet groups displayed symptoms of S. enteritidis infection such as nasal discharge and red crusts around the nostrils after 3 d of challenge. The study culminated after 3 d of challenge for ethical reasons. After challenge with S. enteritidis over 3 d, there was a marked decrease in body weight and feed conversion efficiency (P< 0·05). In contrast, feed intake and water intake did not vary (P>0·05) across the groups fed the different diets. Although body weight declined, there is no information on the composition of the body weight loss. These results were discussed fully elsewhere(10, Reference Balan, Han and Rutherfurd20).
Analysis of mucosal cytokines
S. enteritidis administration resulted in significantly (P< 0·05) higher pro-inflammatory IFNγ, TNFα and lower anti-inflammatory IL-4, IL-10 mucosal concentrations for the challenged BD-fed rats compared with the unchallenged BD-fed rats in the jejunal, ileal, caecal and colonic mucosa. This effect was completely negated in the challenged rats receiving the FDOI-based diet. Challenged rats receiving the AOI diet were found to have similar (P>0·05) pro- and anti-inflammatory cytokine concentrations when compared with the challenged rats fed the BD (Table 2).
Table 2 Intestinal mucosal interferon-γ (INFγ), IL-4, IL-10 and TNFα concentrations in unchallenged rats fed the basal diet (BD) and rats challenged with Salmonella for 3 d and fed the BD, freeze-dried ovine immunoglobulin (FDOI) diet or autoclaved ovine immunoglobulin (AOI) diet (Mean values with their standard errors; n 15)
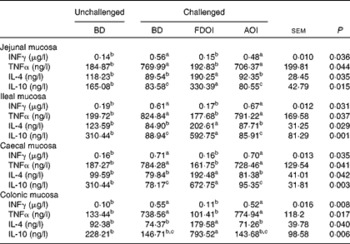
a,b,c Mean values within a row with unlike superscript letters were significantly different (P< 0·05).
Analysis of mucosal immunoglobulins
Mucosal rat IgA (total) concentrations were significantly (P< 0·05) greater in the challenged BD- and AOI-fed rats compared with the unchallenged BD-fed rats in all the segments (jejunal, ileal, caecal and colonic). This effect was not evident in the challenged rats fed the FDOI diet. Consumption of the different diets (BD, FDOI and AOI) did not affect mucosal IgG (in jejunum, ileum and caecum, P= 0·25–0·31) concentrations. However, in the colon, rats challenged and fed either the BD or AOI diet had significantly (P< 0·05) higher mucosal IgG than for the unchallenged rats receiving the BD. There was no significant (P>0·05) difference between the unchallenged BD-fed rats and the challenged FDOI-fed rats. Mucosal IgE levels were not affected (P= 0·27–0·34) by treatment (Table 3).
Table 3 Intestinal mucosal IgA, IgG and IgE concentrations in unchallenged rats fed the basal diet (BD) and rats challenged with Salmonella for 3 d and fed the BD, freeze-dried ovine immunoglobulin (FDOI) diet or autoclaved ovine immunoglobulin (AOI) diet (Mean values with their standard errors; n 15)
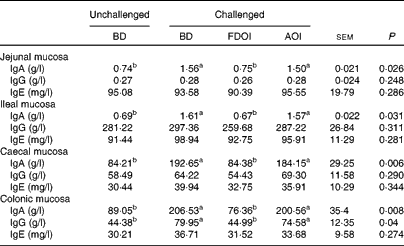
a,b Mean values within a row with unlike superscript letters were significantly different (P< 0·05).
Quantitative analysis of mucosal anti-Salmonella immunoglobulins
In all intestinal mucosal segments (jejunal, ileal, caecal and colonic), ASE IgA levels were significantly (P< 0·05) enhanced for rats (challenged with S. enteritidis) fed the FDOI diet compared with the other groups. A similar trend was evident for the levels of ASE IgG in the mucosal segments (except in jejunum and ileum) where the challenged rats fed the FDOI diet had significantly (P< 0·05) higher levels of ASE IgG compared with the other groups (Table 4).
Table 4 Intestinal mucosal anti-Salmonella (ASE) IgA and ASE IgG concentrations (absorbance) in rats challenged with Salmonella for 3 d and fed the basal diet (BD), freeze-dried ovine immunoglobulin (FDOI) diet or autoclaved ovine immunoglobulin (AOI) diet (Mean values with their standard errors; n 15)
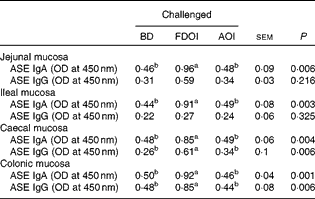
OD, optical density.
a,b Mean values within a row with unlike superscript letters were significantly different (P< 0·05).
Determination of myeloperoxidase activity
Challenging rats with S. enteritidis resulted in significantly (P< 0·05) higher MPO activity in all of the intestinal mucosal segments for the rats receiving the BD compared with the unchallenged BD-fed rats. There was no significant (P>0·05) difference between the challenged AOI-fed rats and the challenged BD-fed rats. This effect was not seen in the challenged rats fed the FDOI diet. MPO activity in the challenged rats receiving the FDOI diet did not differ from those from the unchallenged rats (Table 5).
Table 5 Intestinal mucosal myeloperoxidase (MPO) activity (U/l*) for the unchallenged rats fed the basal diet (BD) and rats challenged with Salmonella for 3 d and fed the BD, freeze-dried ovine immunoglobulin (FDOI) diet or autoclaved ovine immunoglobulin (AOI) diet (Mean values with their standard errors; n 15)
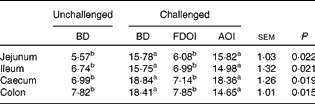
a,b Mean values within a row with unlike superscript letters were significantly different (P< 0·05).
* One unit of MPO is defined as the amount of MPO that hydrolyses the substrate and generates taurine chloramine to consume 1·0 μmol trinitrobenzene (TNB) per min at 25°C.
Mean organ, mesenteric lymph node weights and number of ileal Peyer's patches
Mean pancreas weight relative to empty body weight and MLN weights and the number of ileal Peyer's patches for the rats on the final day (day 18) of the study are shown in Table 6. Challenging with S. enteritidis resulted in significantly (P< 0·05) higher MLN and pancreas weights for the rats receiving the BD. Moreover, pancreas and MLN weights in the challenged rats receiving the AOI diet were not significantly (P>0·05) different from the challenged rats fed the BD. In contrast, the mean pancreas and MLN weights in the rats challenged but receiving the FDOI diet were not significantly (P>0·05) different from the unchallenged rats receiving the BD, but were significantly (P< 0·05) different from the challenged BD- and AOI diet-fed rats. A similar trend was observed for the number of ileal Peyer's patches.
Table 6 Mean mesenteric lymph node (MLN) weights and relative pancreas weights and number of ileal Peyer's patches (n 8) for the unchallenged rats fed the basal diet (BD) and rats challenged with Salmonella for 3 d and fed the BD, freeze-dried ovine immunoglobulin (FDOI) diet or autoclaved ovine immunoglobulin (AOI) diet (Mean values with their standard errors; n 15)
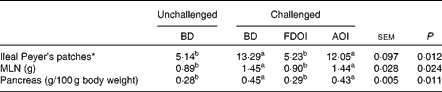
a,b Mean values within a row with unlike superscript letters were significantly different (P< 0·05).
* n 8.
Quantitative analysis of mucin
Data for the amount of mucin in the intestinal mucosal scrapings are shown in Table 7. In the jejunum, ileum, caecum and colon, intestinal mucosal mucin protein content was significantly (P< 0·05) higher for the unchallenged rats fed the BD than for the rats challenged and receiving either the BD or AOI diet. However, in all of the segments, mucosal mucin protein content was significantly (P< 0·05) higher for the challenged rats fed the FDOI diet than for the challenged rats fed either the BD or AOI diet, but was not different (P>0·05) from the unchallenged rats fed the BD.
Table 7 Intestinal mucosal mucin protein concentrations for the unchallenged rats fed the basal diet (BD) and rats challenged with Salmonella for 3 d and fed the BD, freeze-dried ovine immunoglobulin (FDOI) diet or autoclaved ovine immunoglobulin (AOI) diet (Mean values with their standard errors; n 15)
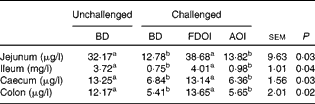
a,b Mean values within a row with unlike superscript letters were significantly different (P< 0·05).
Discussion
In mammals, binding of S. enteritidis to the intestinal mucosa during intestinal infection is known to elicit the production of pro-inflammatory cytokines such as IFNγ, TNF-α (T-helper (Th) 1 cytokines), IL1 and IL-6, chemokines such as IL-8, but to down-regulate Th2 cell cytokines such as IL-4 and IL-10(Reference Eckmann and Kagnoff21, Reference Coburn, Grassl and Finlay22). IFNγ and TNFα have been shown to suppress the invasiveness of Salmonella into the host cells(Reference Degré, Bukholm and Czarniecki23). Generally, Th1 cytokines primarily facilitate cellular immunity against intracellular bacteria and viruses, thereby activating cellular mechanisms that end in phagocyte-dependent inflammation(Reference Corthay24). On the other hand, Th2 cytokines prevent several functions of phagocytic cells, regulate humoral immunity, regulate or suppress inflammation, and promote phagocyte-independent inflammation(Reference Corthay24). The primary antibacterial effect of IFNγ seems to be the suppression of bacterial growth by induction of NO(Reference Makela, Hormaeche and Kaufmann25). In the present study, mucosal IFNγ and TNFα concentrations were significantly lower for the challenged rats fed the FDOI diet when compared with the challenged rats fed the BD, suggesting that the colonisation of foodborne pathogens might be inhibited through enhanced luminal growth suppression of S. enteritidis in the challenged rats fed the FDOI compared with the challenged rats fed the BD or AOI diet. These findings confirm some of our earlier results(10) and those of others(Reference Stoycheva and Murdjeva26) where plasma IFNγ, TNFα, IL-10 and IgA concentrations were significantly lower for challenged rats fed an FDOI diet when compared with challenged rats fed the BD, suggesting that there was a higher translocation of S. enteritidis into the blood of the challenged rats fed the BD than for the challenged rats fed the FDOI diet. Intestinal mucosal IL-4 and IL-10 concentrations were significantly higher for the challenged FDOI-fed rats compared with the other challenged diet groups. This is contradictory to our earlier findings where we showed lower plasma IL-10 levels for the challenged FDOI-fed rats(Reference Balan, Han and Rutherfurd-Markwick14), but in the presently reported study the higher intestinal IL-10 levels may be due to a high enrichment of Lactobacillus strains in the intestinal segments (observed in previous studies) which have been shown to up-regulate intestinal tissue IL-10(Reference Perdigón, Maldonado Galdeano and Valdez27). FDOI prevented the deleterious effects of S. enteritidis on the release of mucosal pro-inflammatory cytokines, suggesting that the effects of immunoglobulin supplements during an inflammatory event involve changes in the mucosal cytokine balance.
Intestinal rat IgA protects mucous membranes by preventing bacteria from attaching to the mucosal surface and works in a process known as immune exclusion, where intestinal IgA binds to the bacteria, pathogen or its antigen, thereby preventing its translocation(Reference Amin, Diebel and Liberati28). In the present study, the feeding of FDOI led to significantly (P< 0·05) lower mucosal IgA concentrations in all of the intestinal segments compared with the challenged rats fed either the BD or AOI diet. This suggests that the pathogen burden was greatly reduced in the rats fed the FDOI. A similar result (but only in colonic mucosa) was observed with rat mucosal IgG concentrations. Conversely, we found higher mucosal ASE IgA and ASE IgG in the challenged FDOI-fed rats, showing that feeding the FDOI diet up-regulated specific humoral immune responses against S. enteritidis, perhaps by increasing either B cell proliferation or differentiation of B cells to secrete ASE IgA and ASE IgG antibodies(10, Reference Balan, Han and Rutherfurd-Markwick14, Reference Jain, Yadav and Sinha29). Rodenburg et al. (Reference Rodenburg, Keijer and Kramer30) using a microarray technique reported that immunity genes in ileal and colonic mucosal segments were up-regulated from day 1 of S. enteritidis challenge, which we have also observed(Reference Balan, Han and Rutherfurd-Markwick14).
The evidence of infection-induced body weight loss in the challenged rats fed the BD or AOI diet also confirms that the systemic infection was more severe in those groups compared with the challenged rats receiving the FDOI diet(Reference Balan, Han and Rutherfurd20). Neutrophils are key cellular components in the inflammatory response and play a key role in host defence against bacterial infections and disease through oxygen-dependent and -independent bactericidal mechanisms(Reference Elsbach, Weiss, Gallin, Goldstein and Snyderman31). A high leucocyte (WBC) count along with high neutrophil count can also indicate a systemic translocation of S. enteritidis. In our previous study, neutrophils and leucocyte counts were lower (P< 0·05) in S. enteritidis-challenged rats fed the FDOI diet when compared with challenged rats fed either the BD or AOI diet. Consistent with the stimulated translocation of S. enteritidis in the challenged rats fed either the BD or AOI diet in the present study, the mucosal inflammatory response (MPO activity) in the jejunal, ileal, caecal and colonic mucosa was significantly (P< 0·05; about two-fold increase) higher compared with challenged rats fed the FDOI diet (Table 5). MPO is a marker of neutrophil accumulation(Reference Sener, Sehirli and Cetinel32) and inflammation(Reference Nieto, Fernandez and Torres33). The observed inhibition of enhanced intestinal mucosal MPO activity by ovine serum immunoglobulins (FDOI) in the S. enteritidis-challenged rats clearly suggests a potent intestinal anti-inflammatory effect against intestinal injury. Previously, we reported that mucosal inflammation (in jejunal, ileal and colonic segments) was not evident in S. enteritidis-challenged rats fed the FDOI diet, based on measures of gut morphology and histology. However, mucosal inflammation in all of the intestinal segments was notably severe in the BD and AOI groups after Salmonella challenge(Reference Balan, Han and Rutherfurd20).
Entry of the entero-invasive salmonellae into the gut tissues is known to occur by colonising of the intestinal epithelium and Peyer's patches (via M cells), passing through the submucosa and arriving at the MLN via the draining lymphatic vessels. From this point, the blood and other vital organs such as the liver and spleen may be colonised. Any damage to the integrity of the epithelium may initiate an opportunity for early access by the organisms(Reference Islam, Moss and Dai34). In the present study, the number of Peyer's patches was significantly (P< 0·05) lower in the challenged rats fed the FDOI diet when compared with the challenged rats fed either the BD or AOI diet (Table 6). In animal models, a reduced number of Peyer's patches has been linked to a higher resistance to orally acquired infection(Reference Prinz, Huber and Macpherson35). Reports have also shown that pathogenic bacteria like Streptococcus or Salmonella may increase the number of M cells within the Peyer's patches which might increase the bacterial translocation(Reference Meynell, Thomas and James36). Moreover, weights of the MLN and pancreas increased significantly (P< 0·05) in the challenged rats fed either the BD or AOI diet compared with the challenged rats fed the FDOI diet or unchallenged rats fed the BD (Table 6). Some of the present results are similar to the findings of Renner et al. (Reference Renner, Nimeth and Demmelabauer37) where they reported a high incidence of acute pancreatitis in adult patients with Salmonella infection(Reference Tositti, Fabris and Barnes38). This is also supported by findings of severe acute pancreatitis during Salmonellosis, suggesting that serious pancreatic disease may represent a complication of Salmonella infection(Reference Hamaguchi, Okabayashi and Yoneda39).
Optimal functioning of the mucosal barrier is vital to prevent bacterial translocation and gut-derived septicaemia(Reference Moncada, Chadee and Blaser40). The decreased intestinal mucosal mucin content observed in the challenged rats fed either the BD or AOI diet compared with the challenged rats receiving the FDOI diet (Table 7) also suggests damage to the gut mucosa or at least potentially compromised mucosal protection. These results are similar to our recent findings(10, Reference Balan, Han and Rutherfurd20) where we reported that rats challenged with S. enteritidis and fed the FDOI diet had significantly (P< 0·05) higher secreted mucin in both ileal and colonic luminal segments. King et al. (Reference King, Morel and Pluske41) also reported that feeding pigs with dried plasma resulted in an increase of gut mucin. Mucin acts as the main structural component of the mucus layer, giving rise to its polymeric, viscoelastic and protective properties. The mesh-like structure of the mucin gel prevents the entry of enteric pathogens and also serves numerous other functions, such as lubrication for the passage of particulate matter; preservation of a hydrated layer over the epithelium; providing a barrier to noxious substances; and forming a permeable gel layer, for the exchange of gases and nutrients, with the underlying epithelium(Reference Laboisse, Jarry and Branka42). Caballero-Franco et al. (Reference Caballero-Franco, Keller and De Simone43) reported that the Lactobacillus species (VSL#3 probiotic formula) were the strongest potentiator of mucin secretion in vitro. In the present study, mucin protein was found to be higher in the jejunal, ileal, caecal and colonic mucosa of the challenged rats fed the FDOI. In our earlier studies, we observed that feeding rats an FDOI-containing diet resulted in an increase in the total Lactobacillus, Lactobacillus johnsonii and L. acidophillus and depletion of enterobacteria in both ileal and colonic luminal segments(10, Reference Balan, Han and Rutherfurd-Markwick14). Thus, feeding the FDOI diet may have resulted in an increase in the number of lactic acid bacteria which may have potentiated the mucosal mucin protein in vivo.
An FDOI diet supported enhanced mucosal immunomodulation when compared with a control diet or with a diet containing AOI (negative control). Given that the immunoglobulin in the AOI diet was inactivated as confirmed by circular dichroism spectral analysis (data not shown), it would suggest that the active immunoglobulin present in the FDOI was responsible for the greater mucosal immunity. An understanding of the detailed mechanistic basis of the effects of FDOI on mucosal immunity awaits future research. However, the results reported in the present study suggest a mechanism by which intact ovine serum immunoglobulins (and not the autoclaved ovine immunoglobulins) may influence the positive effect. Passive inhibition of the adhesion of the pathogenic bacteria to the host intestinal wall may be mediated by antibodies present in FDOI(Reference Han, Boland and Singh44). Lambs are exposed to a much harsher environment than the average human. As a result, it is possible that the animal's immune system is better adjusted to counterattacking the full spectrum of pathogens by producing antibodies against them. It is well known that animal serum contains antibodies that are effective against a variety of pathogens like Escherichia coli, Salmonella, Listeria, C. difficile, rotavirus, and many more(Reference Pierce, Cromwell and Lindemann2, Reference Eibl, Wolf and Furnkranz7, 10, Reference Han, Boland and Singh44). Therefore, intact ovine serum immunoglobulins (FDOI) may provide passive antimicrobial protection by exclusion of opportunistic pathogens, thereby resulting in stronger mucosal immunity. This was not seen with the AOI, as the antibodies present in the AOI fraction were denatured due to heat inactivation.
In conclusion, FDOI selectively modulated the mucosal immune function of rats through enhanced specific immune responses, such as by increasing the production of anti-inflammatory cytokines, anti-Salmonella immunoglobulins and mucin. It also decreased the production of pro-inflammatory cytokines, lowering the MPO activity and non-specific IgA and IgG in the intestinal mucosa. Overall, orally administered FDOI significantly reduced the negative effects of S. enteritidis challenge in the growing rat potentially by passive prevention of attachment and translocation of S. enteritidis. These effects resulted in the rat having an increased resistance to infection. Autoclaving the ovine immunoglobulin fraction leading to denaturation of the immunoglobulins, nullified the immunoglobulin effect.
Acknowledgements
The authors' responsibilities were as follows: P. B. and P. J. M. designed the study; P. B. conducted the research; P. J. M. supervised the research; P. B. analysed and interpreted the results; P. B. wrote the paper; P. B. had primary responsibility for the final content. Both authors read and approved the final manuscript.
P. B. was the recipient of a Riddet Institute doctoral scholarship for his PhD research studies. The authors are grateful to the Riddet Institute, Palmerston North, New Zealand, for providing experimental facilities and financial support for this research project.
The authors declare no conflicts of interest.