The domestic dogs (Canis familiaris) and man share a long history of co-existence that intensified over time to ‘man's best friend’ and a ‘family member’ we keep in many of our homes today. When man lived as nomadic hunter-gatherers, encampments likely attracted carnivorous grey wolves (Canis lupus), the dog's direct ancestors, to scavenge kills or opportunistically take wounded animals that escaped the hunt( Reference Clutton-Brock and Serpell 1 , Reference Driscoll, Macdonald and O'Brien 2 ). After the transition from the Paleolithic to Neolithic Era between 13 000 and 17 000 years ago, when man became sedentary and started agriculture in the Fertile Crescent of the Near or Middle East, a new food niche emerged consisting of human-derived vegetal and animal food waste items( Reference Driscoll and MacDonald 3 ). Wolves opportunistically took advantage of this niche, gradually becoming accustomed to human contact and, over generations, with the multiple domestication and/or interbreeding events with their wild counterparts, the domesticated dog evolved( Reference Driscoll, Macdonald and O'Brien 2 , Reference VonHoldt, Pollinger and Lohmueller 4 ). Breeding efforts during the last 3000–4000 years and, in particular, over the past two centuries have resulted in the remarkable morphological and behavioural diversity of dogs we know today( Reference Driscoll, Macdonald and O'Brien 2 , Reference Driscoll and MacDonald 3 ). The majority of the morphological diversity among dog breeds has a simple genetic basis dominated by less than four quantitative trait loci( Reference Boyko, Quignon and Li 5 ). Although most modern-day dogs no longer look like wolves, they can still interbreed and produce fertile offspring( Reference Vilà and Wayne 6 ) making dogs a subspecies of wolves. Considering the relatively short time span in which domestication occurred and the close genetic relationship, the dog's genome would still predominantly be the product of the environmental selective pressures imposed upon its ancestor, the wolf. Recent evidence shows that three genes (AMY2B, MGAM and SGLT1) involved in starch digestion and glucose uptake were the target of selection during domestication( Reference Axelsson, Ratnakumar and Arendt 7 ). The AMY2B copy number expansion is, however, not fixed across all dog breeds. The Saluki, an ancient breed originating from the Fertile Crescent, showed twenty-nine copies. Ancient breeds such as the Dingo and Siberian Husky show no or limited the expansion (three to four copies), which suggests that these breeds arose alongside hunter-gatherers rather than agriculturists( Reference Freedman, Gronau and Schweizer 8 ). These recent studies also show that other metabolic traits observed in dogs, like capacity to synthesise sufficient amounts of essential nutrients such as niacin, taurine and arginine, were unaffected by domestication. Dogs typically differ in these traits from carnivorous cats (Felis catus) and, in this respect, resemble omnivorous man, pigs and rats. Similar to the ‘metabolic idiosyncrasies’ of cats, their carnivorous nature is also reflected in recent studies focusing on the macronutrient selection by adult cats( Reference Hewson-Hughes, Hewson-Hughes and Colyer 9 , Reference Hewson-Hughes, Hewson-Hughes and Colyer 10 ). Cats choose a protein–fat–carbohydrate profile consisting of 52:36:12 % by energy, which closely matches with the macronutrient profile of free-roaming cats being 52:46:2 % by energy( Reference Plantinga, Bosch and Hendriks 11 ).
The scientific confirmation of the absence of identical or similar specialised metabolic pathways in dogs has led many scientists to question the once-firm carnivorous classification of our domesticated dogs. This ‘omnivorous dog dogma’ has developed over the past 40 years and has found its way into authoritative scientific reference books( 12 , Reference Hand, Thatcher and Remillard 13 ), nutritional concepts in pet nutrition and as a general view. The apparent contradiction between the dogs’ lack of carnivorous traits similar to cats and their ancestral carnivorous ecology has, hitherto, been left unexplained. Here, we present an explanation for the origin of the lack of similar ‘idiosyncratic’ metabolic adaptations of dogs compared to cats by taking into account the foraging ecology and nutrient intake of modern wolves. A literature review of studies reporting data on diet compositions based on stomach contents and scat analyses was used to calculate the nutrient intake of modern wolves.
Dietary and nutrient profiles consumed by wolves
An overview of twenty-six studies reporting fifty diet compositions of wolves in their natural habitat and based on 31 276 wolf scat and stomach analyses is shown in Table 1. The various diet compositions reported in the literature show that wolves in their natural habitat consume a diet predominantly composed of ungulates but supplemented with smaller mammals such as beavers, hares and rodents (Table 2). In Europe, the major dietary items consumed were wild boar, moose, roe deer and red deer. Beavers (Castor fiber) were overall a less common prey animal but contributed 12·6 % of total percentage of weight in one study conducted in the summer in Latvia( Reference Andersone and Ozolinš 14 ). Various other non-ungulate species were consumed by wolves in Europe but contributed overall little ( < 7·8 %) to the total biomass consumed. Other types of animals consumed were birds, reptiles, insects and fish. In North-America, wolves preyed predominantly on moose, white-tailed deer and beaver (Castor canadensis). Diets also contained various medium-size and small prey animals, with the snowshoe hare (Lepus americanus) being the most common but their contribution to the total amount of biomass consumed was low ( ≤ 2·5 %). Darimont et al. ( Reference Darimont, Price and Winchester 15 ) found that considerable amounts of mustelidae were consumed by wolves in Western Canada. Other types of animals consumed were birds, insects, intertidal organisms and fish.
Table 1 Overview of the considered studies presenting diet compositions expressed as percentage of weight for inclusion in the calculations to determine the nutrient composition of wild wolf diets
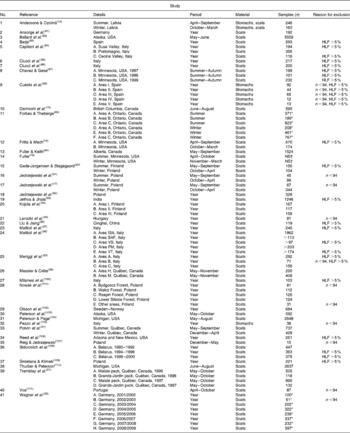
HLF, human-linked foods; A–H, study details with regard to area and/or year.
* Number of scats is not specified in the original manuscript; number of scats after personal communication with authors (i.e. G Forbes and C Wagner).
† Number of scats is not specified in the original manuscript. Considering the large number of total scats (n 2386), it was assumed to be larger than ninety-four for both seasons.
Table 2 Data of dietary profiles of wild wolves found in the literature (% of weight)
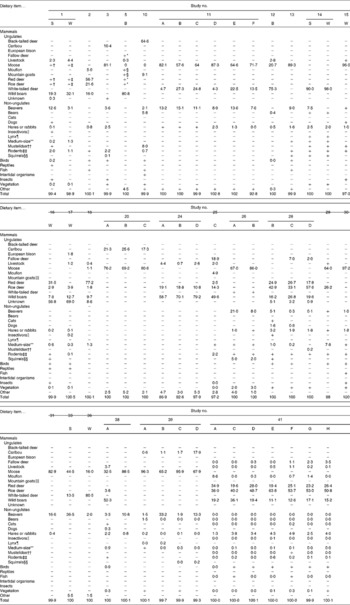
S, summer diet; W, winter diet; A–H, study details with regard to area and/or year (see Table 1); − , food item was not mentioned; +, food item was present but not clearly quantified.
* Comprise together 14·2 % of total biomass consumed; for further calculations, percentage of weight (PW) was equally divided across these species.
† Comprise together 62·2 % of total biomass consumed; for further calculations, PW was equally divided across these species.
‡ Comprise together 56·8 % of total biomass consumed; for further calculations, PW was equally divided across these species.
§ Comprise together 0·2 % of total biomass consumed; for further calculations, PW was equally divided across these species.
∥ Also including shrews (Sorex araneus) and hedgehogs (Erinaceus spp.).
¶ Includes bobcats (Lynx rufus) and Canadian lynx (Lynx canadensis).
** Including foxes (Vulpes spp.), porcupines (Erethizon dorsatum), raccoons (Procyon lotor), raccoon dogs (Nyctereutes procyonoides) and striped skunks (Mephitis mephitis).
†† Including badgers (Meles meles and Taxidea taxus), ermines (Mustela erminea), ferrets (Mustela putorius), martens (Martes spp.), minks (Mustela lutreola and Neovison vison), otters (Lutra lutra and Lontra canadensis) and weasels (Mustela nivalis).
‡‡ Including various species of the rodent family Cricetidae, e.g. microtins or voles (Microtus spp. and Myodes spp.), mice (Apodemus spp.) and muskrat (Ondatra zibethicus).
§§ Also including marmots (Marmota spp.).
∥∥ Also including chamois (Rupicapra spp.).
Plant material was identified in scats in several studies, but the contribution of plant material to the total biomass consumed was not always calculated. For example, Fritts & Mech( Reference Fritts and Mech 16 ) did not include grass in the food analyses calculation as it was not considered a food item, although it could have been ingested intentionally by wolves. For example, Jedrzejewski et al. ( Reference Jedrzejewski, Jedrzejewska and Okarma 17 ) detected grasses and sedges in 32·6 % of all faecal samples. Most often, the grass was present in well-ordered bundles, and in some cases (n 5), it occupied more than 50 % and up to 100 % of the scat by relative volume( Reference Jedrzejewski, Jedrzejewska and Okarma 17 ). It has been hypothesised that consumed grass may act as a scouring agent against intestinal parasites such as roundworms and tapeworms (see Peterson( Reference Peterson 18 )). Those studies that did include plant material in their food analyses reported values between 0·1 and 3 % of the total percentage of weight. Identifiable fruit items found in scats included blueberries (Vaccinium spp.)( Reference Fritts and Mech 16 , Reference Fuller 19 – Reference Tremblay, Jolicoeur and Lemieux 21 ), strawberries (Fragaria spp.)( Reference Fritts and Mech 16 ) and raspberries (Rubus spp.)( Reference Fuller 19 , Reference Wagner, Holzapfel and Kluth 22 ), other berries (Juniperus communis and Vitis vinifera)( Reference Meriggi, Brangi and Matteucci 23 ), nuts (Juglans regia, Corylus avellana, Fagus sylvatica and Castanea sativa)( Reference Meriggi, Brangi and Matteucci 23 ) and other fruits (Rosa spp., Malus spp., Pyrus spp., Prunus spp., Rubus spp. and Sorbus spp.)( Reference Wagner, Holzapfel and Kluth 22 , Reference Meriggi, Brangi and Matteucci 23 ). Corn (Zea mays) was also detected in scats by Wagner et al. ( Reference Wagner, Holzapfel and Kluth 22 ). The fruit items contain some energy in the form of carbohydrates (see online supplementary Table S4) but are, compared to the other dietary items, considerably lower in most other nutrients making their contribution to the overall nutrient intake of wolves negligible. Furthermore, it has been suggested that fruit items are primarily consumed by pups( Reference Fritts and Mech 16 , Reference Tremblay, Jolicoeur and Lemieux 21 ). The consumption of fruit items may underlay the tasting capacity of dogs that cats lack. Dogs have Type D units of taste receptors that respond to a small number of ‘fruity-sweet’ compounds( Reference Bradshaw 24 ). Consumption of vegetable matter is also observed in other carnivores such as polar bears (Ursus maritimus)( Reference Derocher, Andriashek and Stirling 25 , Reference Iversen, Aars and Haug 26 ) and crocodilians( Reference Platt, Elsey and Liu 27 ). Contrary to popular belief, wolves do not consume the (partly fermented) vegetable matter in the rumen of ungulates( Reference Peterson 18 , Reference Fuller 19 , Reference Bump, Peterson and Vucetich 28 – Reference Mech 33 ). During removal, however, the rumen can be punctured and its contents spilled( Reference Peterson, Ciucci, Mech and Boitani 29 ) of which some can be consumed along with other body tissues( Reference Stahler, Smith and Guernsey 30 ). Furthermore, the rumen lining and the intestinal wall can be consumed( Reference Peterson, Ciucci, Mech and Boitani 29 , Reference Stahler, Smith and Guernsey 30 ). Based on these studies reporting data on the foraging ecology, wolves can be considered true carnivores in their nature with vegetal matter being a minor to negligible component of their overall diet.
For the calculation of the nutrient profiles, the data on the dietary items were combined with data on the nutrient composition of each dietary item (see online supplementary material). Similar approaches as used here have been applied for the evaluation of nutrient profiles of the diet of free-ranging cats( Reference Plantinga, Bosch and Hendriks 11 ), badgers( Reference Remonti, Balestrieri and Prigioni 34 ), kiwi birds (Apteryx mantelli)( Reference Potter, Hendriks and Lentle 35 ), and that of the hunter-gatherers or Palaeolithic diet of human subjects( Reference Eaton, Eaton and Konner 36 – Reference Eaton 40 ). The mean dietary DM content was 38·6 % with crude protein contributing the largest part to the DM content (mean 67·2 % of DM) followed by EE (24·9 % of DM) and then ash (6·4 % of DM) (Fig. 1). The dietary content of N-free extract (NFE) was the lowest and contributed 1·4 % to DM. The mean energy content was 2085 kJ/100 g DM and ranged from 2004 to 2244 kJ/100 g DM. The mean Ca content was 1·30 g/100 g DM and varied between 0·83 and 2·04 g/100 g DM. The ratio between Ca and P varied between 0·83 and 1·30 and was on average 1·05. Mean values for Na and K contents were 0·28 and 0·99 g/100 g DM, respectively, and showed little variation. Mean dietary contents of Cu, Zn and Fe were 0·66, 10·8 and 27·3 mg/100 g DM, respectively. Dietary Mg varied between 76 and 109 mg/100 g DM with a mean value of 91 mg/100 g DM.
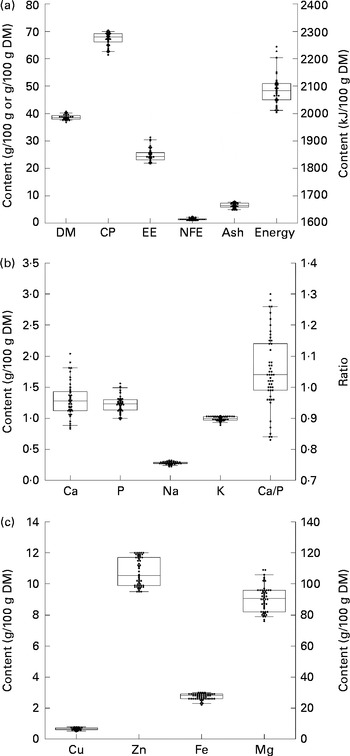
Fig. 1 Calculated macronutrient (a), micronutrient (b) and trace mineral (c) composition of the natural diet (n 43) of wild wolves. The upper and lower hinges represent the 75th and 25th percentiles of the dataset. The band within the box represents the median. The whiskers extend to the 5 % and 95 % CI. For (a), the calculated means are: DM, 38·6 (sem 0·1) g/100 g; crude protein (CP), 67·2 (sem 0·3) g/100 g DM; ethereal extract (EE), 24·9 (sem 0·3) g/100 g DM; nitrogen-free extract (NFE), 1·4 (sem 0·0) g/100 g DM; ash, 6·4 (sem 0·1) g/100 g DM; and energy, 2085 (sem 8) kJ/100 g DM. For (b), the calculated means are: calcium, 1·30 (sem 0·04) g/100 g DM; phosphorus, 1·23 (sem 0·02) g/100 g DM; sodium, 0·28 (sem 0·00) g/100 g DM; potassium, 0·99 (sem 0·01) g/100 g DM; and calcium/phosphorus, 1·05 (sem 0·02) g/100 g DM. For (c) the calculated means are: copper, 0·66 (sem 0·01) mg/100 g DM; zinc, 10·8 (sem 0·13) mg/100 g DM; iron, 27·3 (sem 0·3) mg/100 g DM; and magnesium, 91 (sem 1) mg/100 g DM.
As the nutrient digestibility of most dietary items has not been described in the literature yet, it was not possible to take nutrient bioavailability into account. Considering the digestibility of the macronutrients, proteins are expected to vary most with the more digestible proteins being present in soft tissues (e.g. liver and large muscles) and poorly digestible proteins in bone and hide. The latter are consumed in the later stages of prey consumption( Reference Wilmers, Crabtree and Smith 32 ) or during scavenging( Reference Stahler, Smith and Guernsey 30 ). With the organs and muscles contributing to the majority of the edible proportion of ungulate prey, most proteins may be considered to be well digestible and amino acids to be available for metabolism. Bone is one of the major nutrient sources of Ca and, to a lesser extent, P and Mg, whereas other body tissues provide more than 95 % of Na, K, Cu, Zn and Fe. For these nutrients, bioavailability is dependent not only on the dietary source but also on the nutritional status of the animal. When nutrient supply is limiting or excessive, absorption is up- or down-regulated by homeostatic mechanisms( Reference Fairweather-Tait, Hurrell and Van Dael 41 ). In general, the bioavailability of micronutrients and trace elements in soft animal tissues such as muscle and liver is higher than in vegetal sources( Reference Nohr and Biesalski 42 ). Altogether, the nutrient profiles described provide the hitherto best estimates available for wild wolves but with the limitation that the bioavailability of these nutrients remains to be determined.
The selected protein–fat–carbohydrate profile of wolves in the present study (54:45:1 % by energy) is different from that in dogs, i.e. 30:63:7 % by energy( Reference Hewson-Hughes, Hewson-Hughes and Colyer 10 ). Furthermore, profiles were similar between the five dog breeds studied (i.e. papillon, miniature schnauzer, cocker spaniel, Labrador retriever and St Bernard), indicating that the rapid divergence among dog breeds over the past 200 years was not substantially reflected in the macronutrient priorities among the modern-day breeds of dogs( Reference Hewson-Hughes, Hewson-Hughes and Colyer 10 ). Hewson-Hughes et al. ( Reference Hewson-Hughes, Hewson-Hughes and Colyer 10 ) attributed the lipid selection of dogs to the early domestication period when dogs became ‘adapted’ to a human-associated diet. During this period, such a diet would have consisted of human-derived vegetal and animal food waste items( Reference Driscoll and MacDonald 3 ). Furthermore, commensal species such as rodents may have been a food source for early dogs, as it is (a small) part of many diets for wolves (see Table 2). It is beyond the scope of the present study to consider possible waste items from human settlements, but it would be unlikely that hunter-gatherers disposed such lipid-rich food items when famines and seasonal food shortages were common in those agricultural societies( Reference Prentice 43 , Reference Prentice, Rayco-Solon and Moore 44 ) as the intake of energy from lipids would have been vital for survival. The preference of dogs for lipid-rich diets may thus be a trait that has evolved during the evolution of its ancestor the wolf rather than during early dog domestication.
Fluctuating food availability
Wolves are carnivores that cope with distinct periods of feast and famine, which is different from the ecology of wildcats. These profound fluctuations in food availability have resulted in different coping strategies by wolves. As indicated earlier, wolves hunt in packs on large ungulates and opportunistically scavenge a varied but essentially animal-based diet. During periods of abundant prey availability, wolves ingest large amounts of highly nutritious animal tissues, with a feast meal weight of up to 22 % of their body weight (BW), and a preferential consumption of internal organs such as liver( Reference Stahler, Smith and Guernsey 30 ). During prolonged periods of low prey availability, a pack of wolves may go days without catching large prey during which time they consume smaller prey and some leftovers of old prey( Reference Mech 33 ). Wolves have been observed to scavenge on bone and hide for even up to 10 weeks( Reference Stahler, Smith and Guernsey 30 ). Wolves also consume prey parts they cached for later consumption( Reference Mech and Boitani 45 – Reference Phillips, Danilchuk and Ryon 47 ). When food is available, wolves can quickly recover again from weight loss during fasting. Captive (sedentary) wolves fasted for 10 d lost 7–8 % of their BW, which was replenished after 2 d of consuming white-tailed deer meat with amounts between 15 and 19 % of their BW per d( Reference Kreeger, DelGiudice and Mech 48 ). Also dogs can resist prolonged periods of famine. An adult Scotch collie named ‘Oscar’ has the longest fast on record; after an astonishing 117 d and weighing only 37 % of its initial BW, the fast was stopped( Reference Howe, Mattill and Hawk 49 ). In contrast, wildcats (Felis silvestris), the domestic cat's ancestor( Reference Driscoll, Menotti-Raymond and Roca 50 ), are predominantly solitary and hunt individually catching a variety of mainly small mammals and birds weighing only approximately 1 % of their BW( Reference Pearre and Maass 51 ). Wildcats, therefore, require multiple small prey items per d and thrive in habitats abundant in prey year-round.
During times of low food availability, dogs have been shown to very effectively utilise body fat resources for energy purposes. de Bruijne & van den Brom( Reference de Bruijne and van den Brom 52 ) found that peripheral utilisation of ketone bodies in fasting dogs was very efficient. It was estimated that the contribution of ketone bodies to the daily energy requirement of dogs increased from 7 % in the overnight-fasted state to 13 % after 10 d of starvation. Also, the capacity to decrease metabolic losses and to endogenously synthesise essential nutrients for ongoing metabolic processes will be vital for survival and, as such, has been conserved throughout evolution. Wolves efficiently conserve body proteins by down-regulating enzymes involved in amino acid catabolism to cope with famine( Reference Kreeger, Mech and Boitani 53 ). Such protein sparing capacity is also observed in other carnivores that face prolonged periods of famine such as polar bears (U. maritimus)( Reference Derocher, Nelson and Stirling 54 ), Antarctic fur seal (Arctocephalus gazella) pups( Reference Arnould, Green and Rawlins 55 ) and chicks of king penguins (Aptenodytes patagonica)( Reference Cherel and Le Maho 56 ). Cats, on the contrary, are less capable of conserving protein as they maintain high activities of amino acid catabolising enzymes for gluconeogenesis( Reference Morris 57 ). This difference becomes apparent when fed a diet without protein; adult dogs produce half as much urinary urea as cats (116 v. 243 mg/kg0.75 per d)( Reference Hendriks, Moughan and Tarttelin 58 ). The feline feeding ecology with regular nutrient intake relaxed selection pressures for conserving certain metabolic pathways, which is reflected by low enzymatic synthesis capacity of cats for a number of nutrients (e.g. niacin, taurine, arginine and arachidonic acid)( Reference Morris 57 ). It can be hypothesised that other large carnivores with a feast-or-famine lifestyle such as polar bears, cougars (Puma concolor), lions (Panthera leo) or pinnipeds also have capacities to synthesise essential nutrients such as arginine and niacin or may have developed other metabolic strategies that are key for their survival.
As mentioned earlier, the strong preference for lipids shown by dogs( Reference Hewson-Hughes, Hewson-Hughes and Colyer 10 ) could also be linked to the feeding ecology of wolves. Preferential lipid intake by wolves at times of prey abundance increases adipose tissue that serves as an energy store for periods of low prey abundance. The importance of lipid intake may also be reflected in the preservation of post-carnassial molars in wolves (and dogs) during evolution. These molars are used to crush large bones( Reference Biknevicius, Van Valkenburg and Gittleman 59 ) and provide access to the lipid-rich marrow (see online supplementary Table S3). The extent of seasonal fasting in nature may also explain why mink (Mustela vison), which preys on small animals such as wildcats and encounter periods of famine( Reference Mustonen, Puukka and Pyykonen 60 ), selects an intermediate diet containing 35 % protein and 50 % lipid by energy( Reference Mayntz, Nielsen and Sørensen 61 ). This latter observation, however, requires further study as it may merely reflect the selection for macronutrients as in their normal diet fed over generations( Reference Mayntz, Nielsen and Sørensen 61 ). Macronutrient profiling of other carnivorous species varying in lifestyle might provide insight if profiles can be linked to the extent of seasonal fasting in nature.
At times of feast, when large ungulates are killed, wolves in general rapidly open the body cavity and consume the internal organs such as the heart, lungs, liver, spleen and kidneys( Reference Stahler, Smith and Guernsey 30 ). The liver of an ungulate would provide stored vitamin A and potentially glycogen. Dogs transport vitamin A as retinyl esters in the blood and clear blood retinyl through the kidney( Reference Schweigert, Ryder and Rambeck 62 ), which makes them more resistant to hypervitaminosis A and can be considered as functional for wolves. Although it is unclear what proportion of the glycogen stores remains after the chase and catch of the prey, intestinal amylase and hepatic glucokinase activities as present in domestic dogs would be functional for glycogen utilisation. Furthermore, liver contains glycogen that, like starch from plants, can be digested and utilised by the use of intestinal amylase and hepatic glucokinase. The higher activities of these enzymes in dogs than in cats( Reference Kienzle 63 , Reference Washizu, Tanaka and Sako 64 ) may be consistent with a diet periodically high in liver and muscle tissue rather than a diet high in plant starch.
Dogs share numerous typical carnivorous characteristics with cats (see Fig. 2). For example, both species lack salivary amylase, have a short and simple gastrointestinal tract, conjugate bile acids with taurine and are unable to synthesise vitamin D( 12 ). The metabolic adaptations, such as protein sparing and endogenous niacin synthesis capacity, facilitating survival during times of low dietary nutrient availability are similar to those observed in omnivores, and this may be a key factor to explain differences in digestive physiology and metabolism between today's domestic dogs and cats. Omnivores, such as pigs and rats, may not experience similar periods of famine like wolves but more specifically encounter fluctuations in amounts and types of vegetal and animal matter in their diets (e.g. due to season). The omnivorous pig and rat, consuming lower and fluctuating amounts of protein due to seasonal changes in availability of vegetal and animal matter, can reduce urea excretion even further to 70 and 60 mg/kg0·75 per d( Reference Hendriks, Moughan and Tarttelin 58 ), respectively. It can be speculated that the array of metabolic traits shaped by a feast-or-famine lifestyle enabled carnivorous wolves to metabolically thrive on relatively lower-nutritious human food wastes. These wolves were likely already capable of utilising the starch from plants. Glycogen stored in muscles and in particular liver from prey is, after consumption by carnivores, processed similarly using pancreatic amylase, small intestinal GLUT and hepatic glucokinase. Those dogs with genetic mutations in the AMY2B, MGAM and SGLT1 genes were more fit to this new niche opened up by man( Reference Axelsson, Ratnakumar and Arendt 7 ). Furthermore, this niche favoured wolves smaller in body size, which required less energy, and those more tolerant to human proximity( Reference Coppinger and Coppinger 65 ).
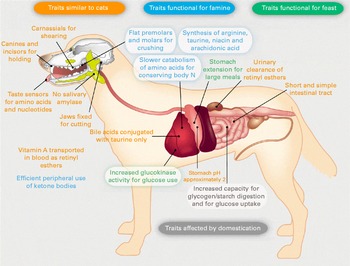
Fig. 2 Omnivorous dog traits revisited. Dogs are classified as omnivores based on traits that are different from carnivorous cats. The authors hypothesise that these ‘omnivorous’ traits, highlighted in white boxes, reflect the typical feast-or-famine lifestyle of the carnivorous dog's ancestor, the wolf. Traits outlined in green and blue are functional for periods of feast and famine, respectively. Dogs share numerous traits with cats, shown in orange. Capacities of traits shown in grey are the target during domestication( Reference Axelsson, Ratnakumar and Arendt 7 ).
Comparison with dog nutrition
The wolf's natural dietary nutrient profile differs in several aspects from the nutrient guidelines and in nutritive characteristics of commercial foods. Table 3 provides the average dietary nutrient profile for wild wolves (based on n 50 diets) reported in the present study (in units/MJ metabolisable energy (ME)) compared to the minimum nutrient requirement of growing dogs and of dogs at maintenance as provided by the National Research Council( 12 ). The physiological minimum nutrient requirement have been accurately determined for several nutrients and can be considered to represent the limit of the adaptation capacity of domestic dogs in relation to dietary nutrient concentrations( Reference Plantinga, Bosch and Hendriks 11 ). Contents of crude protein, Ca, Zn, Fe and Mg in the average wolf diet are well above the set minimum nutrient requirement for these nutrients, although the bioavailability of these nutrients is unknown.
Table 3 Approximated dietary nutrient profiles reported in the literature of wild wolves, profiles as affected by a wolf's ranking and during scavenging, and minimal and recommended allowance (RA) nutrient composition for dogs in growth and at maintenance

ME, metabolisable energy; CP, crude protein; EE, ethereal extract; NFE, N-free extract; NA, not available.
* Average dietary profiles reported in the literature (n 50, see Table 2).
The recommended allowance set by National Research Council( 12 ) for dietary Ca for growth and Cu for growth and maintenance is higher than that found in the average diet of wolves (Table 3). Furthermore, the average Ca content of the wolf's diet is lower than that found in commercial dry foods, and the wolf's dietary Na and Mg contents are lower than the average for commercial dry and moist dog foods. It should be noted, however, that due to higher energy requirements, the actual daily nutrient intake of wolves is higher than that of the average sedentary pet dog living in a temperate environment. The daily energy requirement of adult wolves (35 kg BW) has been estimated to be 25 025 kJ ME( Reference Peterson, Ciucci, Mech and Boitani 29 ) or 1739 kJ/kg0·75 BW, which is 3·2 times higher than the daily energy requirements for the maintenance of adult dogs (544 kJ/kg0·75 BW)( 12 ). Thus, the actual daily intake of micronutrients and trace elements included in the profile would be higher in wild wolves than in pet dogs fed foods with nutrient contents close to the recommended allowance values and fed the average commercial foods reported in Table 3. Little information is available about how nutrient requirements vary with energy expenditure in dogs( 12 ), but it is reasonable to assume that the increase in energy requirements for physical activity and thermoregulation does not result in an equal increase in reported micronutrients and trace elements. Hence, the wolf's metabolism may be accustomed to high dietary availability of these nutrients.
The nutritive characteristics of commercial foods may differ in several aspects from the wolf's natural dietary nutrient profile, and this may pose physiological and metabolic challenges that dogs need to cope with. The average nutrient composition of commercial high-quality dry extruded (n 93) and moist canned (n 39) dog foods( Reference Debraekeleer, Hand, Thatcher, Remillard and Roudebush 66 ) is presented in Table 3. The average dietary NFE content of dogs fed dry and moist commercial foods is substantially higher (25·8 and 20·0 g/MJ ME, respectively) than the dietary NFE content of wolves (0·7 g/MJ ME). The NFE content in dog foods mainly originates from the starch of cereal grains. These starches are cooked during processing and are, therefore, generally well digested by dogs, with ileal apparent digestibility reaching values of above 99 % for starches in dry extruded diets( Reference Murray, Flickinger and Patil 67 ). For wolves, the NFE intake would be conditionally high and in the form of glycogen, i.e. when a liver of a large prey, containing approximately 10·5 g/MJ ME in a white-tailed deer( Reference McCoullough and Ullrey 68 ) (see also later texts), is consumed. To the authors’ knowledge, the glycaemic index of glycogen is unknown, but wolves are not likely to be exposed to a daily glycaemic load that pet dogs fed commercial dry and wet foods may experience. Although the dog has been shown to have an increased digestive and absorptive capacity to cope with starch-containing foods compared to wolves, the impact of a consistent high amount of absorbed glucose on the dog's health and longevity remains to be determined.
The average content of EE of the wolf's diet was slightly higher than the average content normally observed in commercial foods. The origin of lipids in both diets, however, would also be different resulting in a different fatty acid profile consumed. Dog foods contain lipids from vegetable oil (e.g. soyabean, sunflower and maize) and/or animal origin (e.g. pork fat, beef tallow, poultry fat and fish oil). Lipids from vegetable origin are typically higher in n-6 PUFA than in n-3 PUFA. In addition, with regard to the n-3 PUFA, vegetable oils are relatively rich in α-linolenic acid (18 : 3n-3) whereas those from animal origin are typically high in EPA (20 : 5n-3) and DHA (22 : 6n-3). The PUFA profile lipids derived from animals vary according to diets fed during rearing. The n-6:n-3 ratios in muscle lipids of cattle and chickens raised in captivity range between 6:1 and 19:1( Reference Rule, Broughton and Shellito 69 ) whereas those for wild mule deer and red deer contain a ratio of approximately 2:1 to 3:1( Reference Cordain, Watkins and Florant 70 ), closely matching the ratio of 2:1 of wolf subcutaneous fat( Reference Käkelä and Hyvärinen 71 ). Also, modern aquaculture produces fish that contain less n-3 PUFA than do their wild counterparts, although the n-6:n-3 ratio is still very low (1:6 for cultured and 1:11 for wild salmon (Salmo salar)( Reference Simopoulos 72 )). The vegetable and animal lipid sources commonly used in dog foods result in higher n-6:n-3 ratio than that of lipids from wild animals. Commercial dog foods (n 12) showed an average n-6:n-3 ratio of 8:1, ranging from 5:1 to 17:1( Reference Ahlstrøm, Krogdahl and Vhile 73 ). Furthermore, the average PUFA concentration in the lipid fraction of these dog foods was 24·4 % (range 18·1–43·1 %)( Reference Ahlstrøm, Krogdahl and Vhile 73 ), which is lower than 31·3 and 28·7 % PUFA content in muscle lipids of mule deer and red deer, respectively( Reference Cordain, Watkins and Florant 70 ). Considering the involvement of n-3 PUFA (EPA and DHA) in numerous physiological processes, including the mediation of inflammatory and immune responses, renal functioning, cardiovascular health and neurologic development( Reference Simopoulos 72 , Reference Bauer 74 – Reference Bosch, Beerda and Hendriks 76 ), the fatty acid profiles of our pet dog foods deserves careful (re)consideration. The impact of a relative shortage of n-3 fatty acids in the commercial diet on the functioning immune system of dogs requires further study.
Undigested dietary fractions provide substrates for the microbiota in the distal small and large intestine and the type of substrates can be expected to differ between those of a wolf's diet and dry and moist dog foods. Whole prey and, specifically, lower-quality animal tissues (e.g. hide and bones) provide low digestible or indigestible substances such as cartilage, collagens and glycosaminoglycans, with specific fermentative characteristics( Reference Depauw, Bosch and Hesta 77 ) other than fibres of vegetal origin and indigestible proteins in processed foods. Given the involvement of the intestinal microbial community in host physiology, immune function and behaviour( Reference Sekirov, Russell and Antunes 78 ), the effect of these specific substances on the canine microbial composition and activity and on canine (intestinal) health warrants further investigation.
The concept of ‘natural’ foods that may better match the physiological and metabolic make-up of dogs is comparable to the paradigm that the Palaeolithic hunter-gatherer diet would better fit modern man than our current nutrition. The discordance hypothesis originally described by Eaton & Konner( Reference Eaton and Konner 37 , Reference Konner and Boyd Eaton 79 ) states that the human genome evolved to adapt to conditions that no longer exist, the change from Palaeolithic to current nutrition occurred too rapidly for adequate genetic adaptation and the resulting mismatch helps to cause some common ‘diseases of civilisation’ such as diabetes mellitus, obesity and dental disease( Reference Eaton, Konner and Shostak 80 ). Furthermore, the nutrient intake of modern-day hunter-gatherers is suggested to represent a reference standard for modern human nutrition and a model for defence against these diseases. To what extent the discordance hypothesis may also apply for dogs and to what extent the nutrient profile of the wolf's diet is optimal for pet dogs are subjects for study. The nutrient profile described here originates from a wolf population living under severe physiological and climatic conditions and in which nutrition is a precondition for species survival and procreation. In general, our domestic dogs have a much more sedentary lifestyle, regular meals and a longer lifespan, which may have a significant effect on nutrient requirements and handling. Dogs have adapted to a starch-rich diet (i.e. increased enzymatic capacity to digest starch and increased glucose uptake capacity) and are able to cope with large variations in nutrient intake. There are also situations of reduced adaptation capacity (such as geriatrics and chronic disease) in which the consumption of a diet that requires an adaptable metabolism may place the animal under stress. Nevertheless, the wolves’ feeding ecology and nutrient intake may provide valuable information to further improve our understanding of the origin of the dogs’ digestive physiology and metabolism and possibly provide new leads for optimising individual health and longevity.
Conclusions and implications
Data on the wolf's feeding ecology show that the progenitors of our modern-day dogs were adaptive, true carnivores and not omnivores. During times of feast and famine, wolves would have had to cope with a variable nutrient intake requiring an adaptable metabolism, which is still functional in our modern-day dogs. These traits may also allow wolves to make the transition from carnivory to omnivory during domestication. The nutritive characteristics of commercial foods differ in several aspects from the dog's closest free-living progenitor in terms of dietary nutrient profile, and this may pose physiological and metabolic challenges that dogs need to cope with. The question remains to what extent the approximated nutrient profile also optimally supports health and longevity of domestic dogs with a more sedentary lifestyle and a longer lifespan in a different environment. The present study describing the wolf's dietary nutrient profile may provide an impetus for further research in this area similar to research activities in the field of human nutrition( Reference Konner and Boyd Eaton 79 ). Laboratory, clinical and epidemiological studies would be required in which the nutrient profile and other aspects of a wolf's diet are translated and evaluated for their contribution to the health and longevity in today's pet dogs.
Supplementary material
To view supplementary material for this article, please visit http://dx.doi.org/10.1017/S0007114514002311
Acknowledgements
This research was funded by Wageningen University and Utrecht University. All authors contributed fundamentally to the present manuscript. G. B. contributed to all facets including research design, data collection, calculations and writing the initial manuscript. E. A. H.-P. and W. H. H. contributed to research design, data interpretation and manuscript preparation. The authors thank David L. Mech for his willingness to answer the questions relating to the feeding ecology of wolves. Laura de Vries, Gijs Hulsebosch, Joyce Neroni and Esther Lichtenberg are thanked for their contributions at the beginning of this research. There are no conflicts of interest.