Introduction
The wide diversity of rice germplasm enables it to be cropped over a wide geographical distribution globally across temperatures ranging from as cool as 15°C to exceeding 40°C (Zhang et al., Reference Zhang, Su, Li, Chen and Zhu2005; Wassman et al., Reference Wassman, Jagadish, Sumfleth, Pathak, Howell, Ismail, Serraj, Redona, Singh and Heuer2009). Nonetheless, temperature, particularly extreme values, affects the rate at which crops develop from sowing to maturity, and their yield, which limits their range of cultivation (Evans, Reference Evans1993). In rice (Oryza sativa L.), duration to maturity, and so crop adaptation, is affected by temperature, photoperiod and possibly diurnal temperature amplitude, with considerable variation amongst genotypes in responsiveness to these environmental variables (Collinson et al., Reference Collinson, Ellis, Summerfield and Roberts1992; Yin et al., Reference Yin, Kropff and Ellis1996). Seed set in rice is vulnerable to low temperatures (12–20°C) during the reproductive phase or to high day (35–38.5°C) or night (30–35°C) temperatures in the early reproductive period up to and including anthesis, with considerable variation amongst genotypes in resilience to such temperature stress (Yoshida, Reference Yoshida1981; Jagadish et al., Reference Jagadish, Craufurd and Wheeler2008; Coast et al., Reference Coast, Ellis, Murdoch, Quiñones and Jagadish2015; Martínez-Eixarch and Ellis, Reference Martínez-Eixarch and Ellis2015). In addition to effects on yield, rice seed quality is also affected by high temperature during seed development, particularly during histodifferentiation and early seed-filling (Ellis et al., Reference Ellis, Hong and Jackson1993; Ellis and Hong, Reference Ellis and Hong1994; Ellis, Reference Ellis2011; Martínez-Eixarch and Ellis, Reference Martínez-Eixarch and Ellis2015).
Seed quality is important for the long-term conservation of germplasm as it affects seed storage longevity in genebanks (Hay and Smith, Reference Hay, Smith, Smith, Linington, Dickie, Pritchard and Probert2003). Rice seed quality – such as ability to germinate, desiccation tolerance and survival period under air-dry storage – begins to be acquired early in seed development and, in favourable environments, continues to improve in planta until late in maturation drying (Ellis et al., Reference Ellis, Hong and Jackson1993; Ellis and Hong, Reference Ellis and Hong1994; Ellis, Reference Ellis2011). Moreover, rice seeds, which were metabolically active at harvest (≥16.2% moisture content), improved considerably (up to 3-fold in more moist samples) in subsequent air-dry longevity when subjected to initial, intermittent (8 h day–1) high-temperature (45°C) air-drying ex planta in comparison with immediate post-harvest drying at 15°C and 15% relative humidity (RH) (Whitehouse et al., Reference Whitehouse, Hay and Ellis2015). This latter drying regime is within the multi-species range of 5–20°C with 10–25% RH recommended for genebanks (FAO, 2013).
The importance of seed drying in order to extend subsequent seed storage longevity in genebanks is well recognized (Cromarty et al., Reference Cromarty, Ellis and Roberts1982; FAO, 1994, 2013; Rao et al., Reference Rao, Hanson, Dulloo, Ghosh, Nowell and Larinde2006), but high temperatures are usually avoided to reduce the risk of seed deterioration, especially when seeds have a high moisture content (Nellist, Reference Nellist and Hebblethwaite1980; Cromarty et al., Reference Cromarty, Ellis and Roberts1982; McDonald and Copeland, Reference McDonald and Copeland1997). Heated-air seed drying practices recognize safe drying temperatures (maximum value avoiding damage to ability to germinate) which vary with initial moisture content, design of drier, or whether air or seed temperature is specified (Nellist, Reference Nellist and Hebblethwaite1980). However, much of the research involving heated-air seed drying used rewetted mature, dry seeds (Nellist, Reference Nellist and Hebblethwaite1980). Compared with many other species, safe drying temperatures reported for rice seeds are comparatively high: for example, 48.9°C at 20% moisture content or above, 51.7°C at 15–20% moisture content, and as high as 60°C for initial seed moisture content of 15% or below (Lewis, Reference Lewis1950). Harrington (Reference Harrington and Kozlowski1972) suggested a cooler limit of 45°C for drying all cereal seeds, whilst Yamashita (Reference Yamashita and Yamashita1993) recommended an air temperature of only 40°C for rice seeds with ≥24% moisture content. Similarly, Jittanit et al. (Reference Jittanit, Srzednicki and Driscoll2010) reported that rice seeds dried at 40°C for 50–250 minutes showed >85% germination, whereas germination was adversely affected at ≥60°C. They also reported less damage from drying in a batch than a fluidized-bed dryer at any one temperature. With traditional sun drying, on the other hand, temperatures can reach up to 52°C and if carried out carefully rice seed viability can be maintained well thereafter (Regalado and Brena, Reference Regalado and Brena2006).
Many investigations of high-temperature drying in rice relate to milling quality. Drying at high temperatures creates intra-kernel moisture content gradients that can lead to fissure formation and reduced milling yield if seeds cool before tempering (Chen et al., Reference Chen, Siebenmorgen and Marks1997; Cnossen and Siebenmorgen, Reference Cnossen and Siebenmorgen2000, Reference Cnossen and Siebenmorgen2002; Schluterman and Siebenmorgen, Reference Schluterman and Siebenmorgen2007). Hence for milling rice, Lewis (Reference Lewis1950) recommended cooler temperatures than for seeds: 40.6°C at 20% or more, 46.1°C at 15–20%, and 51.7°C for initial seed moisture contents of 15% or below. More recently, two-stage drying (initial high temperature and high velocity followed by ambient conditions with reduced air flow) has been used to dry high-moisture content seeds to reduce damage to milling yield (Jittanit et al., Reference Jittanit, Srzednicki and Driscoll2010; and references therein). The beneficial high-temperature procedure for rice seed longevity reported by Whitehouse et al. (Reference Whitehouse, Hay and Ellis2015), as above, was in fact a multi-stage treatment: an initial diurnal cycle (8/16 h, 45°C/15°C for 1–6 days) followed by a final period at 15°C (15% RH).
We report here an investigation of the high-temperature limits for seed longevity when drying rice seeds harvested at different moisture contents and days after anthesis (DAA) in order to explore whether maturing rice seeds, which are still metabolically active at harvest, benefit from high-temperature exposure due to continued development or a stress-related response ex planta.
Materials and methods
Plant material
Seeds of an aromatic variety of rice from the International Rice Genebank Collection, accession IRGC 117265 (McNally et al., Reference McNally, Child, Bohnert, Davidson, Zhao, Ulat, Zeller, Clark, Hoen, Bureau, Stokowski, Ballinger, Frazer, Cox, Padhukasahasram, Bustamante, Weigel, Mackill, Bruskiewich, Ratsch, Buell, Leung and Leach2009), and of a commonly grown indica variety, ‘Macassane’, were planted for harvest in the 2015 dry season (DS) and 2016 wet season (WS), respectively (Fig. 1A,C). Seeds were sampled either from the International Rice Genebank (IRG) active collection (4°C) (IRGC 117265; https://doi.org/10.18730/1PG6J) or the storage facility (20°C and 30% RH) at the upland site (‘Macassane’) and held at 50°C for 5 days to break dormancy. Staggered sowing of seeds from IRGC 117265 was conducted to enable simultaneous harvests at 25, 35 and 45 days after 50% anthesis (DAA) on two separate occasions, 13 days apart, to achieve two seed lots per stage of maturity differing in harvest moisture content (Table 1 and Fig. 1A). For ‘Macassane’, all seeds were sown on 7 June 2016 and harvested on three separate dates at 34, 36 and 38 DAA (Table 1 and Fig. 1C). All seedlings, from both varieties, were raised in a seed bed before being transplanted to plots on the International Rice Research Institute (IRRI) Ziegler Experimental Station (ZES) (14° 9′ 3.5742″ N, 121° 15′ 54.504″ W) and normal rice production practices and plant protection measures were followed. Seeds were harvested in April 2015 and October 2016 for IRGC 117265 and ‘Macassane’, respectively (Table 1 and Fig. 1A,C).

Figure 1. Illustration of the experimental design showing the factorial combination of different seed production and seed drying treatments for rice accession IRGC 117265 (A and B) and cv. ‘Macassane’ (C and D). Seeds were sown (S) for harvest (H) in either the 2015 dry season (DS) or the 2016 wet season (WS). In graphs B and D the dotted lines represent the drying room (15°C/15% RH) and the continuous black lines represent alternative drying conditions. The continuous grey lines show the duration seeds were held in an air-tight box at room temperature (21.5°C).
Table 1. Dates of sowing and harvest and the seed moisture content (MC) and equilibrium relative humidity (eRH) at harvest of rice accession IRGC 117265 and cv. ‘Macassane’ harvested 25–45 or 34–38 days after 50% anthesis (DAA), respectively
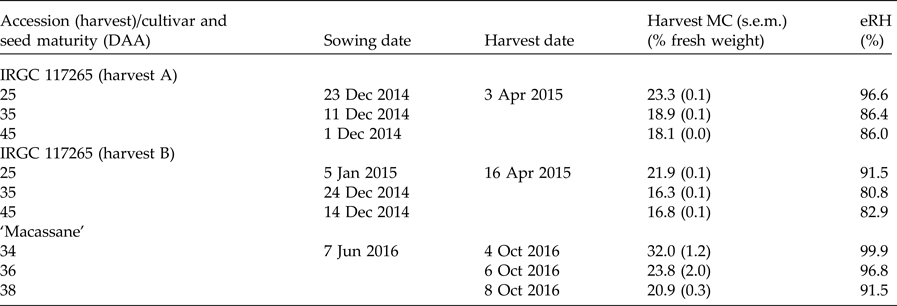
Immediately after harvest, the seeds of each variety were threshed and blown to remove debris and half-filled grains before they were transported to the laboratory where the seeds underwent manual cleaning – discarding any empty, damaged and/or diseased seeds – and the initial temperature, equilibrium relative humidity (eRH; %) and the moisture content (MC; % fresh weight) were measured. The eRH was measured at room temperature (21.5°C) using an AW-D10 water activity station in conjunction with a HygroLab 3 display unit (Rotronic South East Asia, Singapore). Seed MC (fresh weight basis) was determined using three 5 g samples from each seed lot (harvest × DAA) using the high-constant-temperature oven method (ISTA, 2013).
Seed drying over saturated MgCl2 (IRGC 117265)
Seeds of IRGC 117265 at each maturity stage and from A and B harvests were divided into 9 × 200 g samples and placed into 0.2 × 0.33 m (length × width) nylon mesh bags (1 mm diameter holes) in which they were stored inside sealed 0.6 × 0.3 × 0.132 m (length × width × height) electrical enclosure boxes (ENSTO, Porvoo, Finland) at room temperature (21.5°C) overnight to limit drying until the treatments began the following morning (08.00 h). A sample from each maturity stage was transferred directly to the genebank drying room maintained at 15°C and 15% RH (Fig. 1B). As the drying room conditions comply with the current genebank standards (FAO, 2013), the treatments dried throughout therein provided a control, i.e. the baseline against which the effects of other drying treatments were compared. To provide the other drying environments, the remaining seed samples were placed over a 700 ml super-saturated solution of MgCl2 in sealed electrical enclosure boxes, producing an RH of approximately 30%, and transferred either to the drying room (15°C) or to incubators at 30, 45 or 60°C (Fig. 1B). Within these drying environments, there was no airflow through the seeds; rather the drying process was passive with the desiccant absorbing moisture from the seeds, without affecting equilibrium RH within the container, and the temperature driving the evaporation of moisture from the seeds to their surroundings. Seed samples were exposed to intermittent (Int; 8 h day–1) and continuous (Cont; 24 h) drying treatments for 3 days at each temperature regime before final drying for 11 days in the drying room (i.e. equilibrating to 15°C/15% RH; expected MC 6–7%). During the non-active drying phase (Int; 16.00–08.00 h) seeds were sealed in empty (without MgCl2) electrical enclosure boxes at 21.5°C until the following morning (Fig. 1B). The change in weight and eRH of samples was monitored daily, at 16.00 h for intermittently dried seeds and at 08.00 h for continuously dried seeds, until samples were transferred to the drying room where this was subsequently recorded every 3 days. The dried seeds were then sealed inside 0.16 × 0.24 m (length × width) laminated aluminium foil packets (Moore and Buckle, Saint Helens, UK) and stored at 2–4°C until experimental storage began.
Seed drying over silica gel (‘Macassane’)
Seeds of ‘Macassane’ harvested at 34, 36 and 38 DAA were divided into 25 × 200 g samples and placed into nylon mesh bags (as described above) (Fig. 1D). A sample was immediately transferred to the drying room (15°C with 15% RH) and the remaining samples were stored inside individual 0.3 × 0.3 × 0.132 m (length × width × height) electrical enclosure boxes containing 400 g of (previously dried) silica gel (granular 0.2–1 mm; Sigma-Aldrich, Singapore). A box was transferred to either the genebank drying room (15°C) or to incubators at 30, 45 and 60°C at 10 min intervals (time taken to measure sample eRH; done to ensure all samples were dried for the same period) until there were a total of six boxes at each temperature (Fig. 1D). The change in weight and eRH (as described above) was monitored for each sample after 2, 4, 8, 12, 16, 24, 72, 144, 216, 288 and 336 h. A sample was removed and transferred from each high-temperature regime after 2, 4, 8, 12, 16 and 24 h to the drying room for final drying (Fig. 1D). At 14 days after harvest, samples from all drying treatments were removed, final weight and eRH of each recorded, before they were sealed inside laminated aluminium foil packets and stored as described above.
Seed storage
Seed samples were removed from temporary cold storage (2–4°C) and equilibrated to room temperature (21.5°C) before opening. Each sample was split into 29 × 5 g subsamples which were placed into 30 mm diameter open Petri dishes in a VC3 0034-M climate chamber (Vötsch Industrietechnik, Germany) set at 60% RH and 21.5°C for 4–5 days. Once equilibrium had been reached (approximately 10.9% MC), four of the 5 g subsamples from each treatment combination were removed to measure seed eRH; three of these were then used to determine MC and the fourth to estimate initial ability to germinate. The remaining subsamples (25) were each sealed inside individual aluminium foil packets (0.12 × 0.08 m, length × width) before being placed in an incubator at 45°C. One packet per treatment combination was removed from storage at 45°C at 3-day intervals up to 54 days to test ability to germinate. Moisture content determinations, using three additional 5 g packets of seeds, were made at the mid-point and end of storage. These analyses confirmed that seed storage moisture content was stable during experimental storage.
Seed germination
Ability to germinate was estimated with four replicates of 30 seeds, sown on two layers of Whatman no. 1 paper wetted with 7.5 ml distilled water in 90 mm diameter Petri dishes and incubated at constant 30°C (12 h light and 12 h dark cycle). Germination was scored after 3, 5, 7 and 14 days; seeds were scored as germinated when the radicle had emerged by at least 2 mm. After the test, mouldy seeds were discarded and non-germinated, hard seeds were dehulled and tested for an additional 7 days before final scoring.
Statistical analysis
Seed survival curves were fitted by probit analysis using GenStat for Windows, version 17 (VSN International Ltd, Hemel Hempsted, UK). As seeds from both varieties typically show dormancy, a probit model combining loss of dormancy (during early storage) with loss of viability (Eqn 1) was applied using the FITNONLINEAR directive in GenStat:

where g is the ability to germinate in normal equivalent deviates (NED), K d is the initial proportion of non-dormant seeds (NED), β 1 is the probit rate of loss in dormancy and p is the storage period (days); K i and σ are as in the Ellis and Roberts (Reference Ellis and Roberts1980) viability equation:

where v is the viability (NED) of a seed lot stored for period, p, K i is the initial viability (NED) and σ (days) is the standard deviation of the normal distribution of seed deaths in time. The time for viability to fall to 50% (p 50, product of K i and σ) was also estimated and used as a measure of longevity. For those seed lots that showed a reduced initial viability and a systematic pattern of residuals when only fitting Eqn (1), the ‘control mortality’ parameter (‘immunity’ in GenStat), which estimates the proportion of ‘non-responding’ seeds (i.e. dead or empty) within the population (Mead and Gray, Reference Mead and Gray1999), was included in the probit analysis. Probit analysis was carried out for all seed lots simultaneously, fitting the full model (different estimates for all parameters).
Results
Seed drying rate
The harvest eRH of all the seed lots was high (80.8–99.9%; Table 1). For each seed production, the moisture content of seeds harvested at the lowest DAA was highest (21.9–32.0%). Moisture content reduced with increasing maturity, but sometimes the intermediate sample provided the lowest value: ‘Macassane’ seeds at 34 DAA were the most moist and IRGC 117265 seeds at 35 DAA (harvest B) the driest (16.3%). All drying treatments, including the control, provided the characteristic negative logarithmic decline, with progressively more rapid moisture loss as temperature increased from 15 to 60°C (Figs 2A–D and 3A). Later harvests, with lower initial MC, showed slower drying rates at all temperatures; this was most apparent at 15°C. Most of this effect of later harvest date affected moisture loss over the first day only. The pattern of drying of seeds in the drying room was similar to 45°C/30% RH over the first 3 days of continuous drying at all maturity stages (Fig. 2C,D), whereas seeds dried faster at all temperatures over silica gel compared with the drying room (Fig. 3A).

Figure 2. Seed drying curves (A, B, C, D) at 15 (●), 30 (▲), 45 (♦) or 60°C (▼) over saturated MgCl2 (30% RH), either intermittently (8 h day–1; A, B) or continuously (24 h day–1; C, D), for rice accession IRGC 117265 harvested on 3 April 2015 (harvest A) or 16 April 2015 (harvest B) after 25, 35 or 45 days after 50% anthesis (DAA). After these initial treatments seeds were dried further in the drying room (15°C/15% RH, 11 days). Interrupted lines show loss in moisture content for seeds dried throughout in the drying room (DR) control (15°C/15% RH; ○). Loss in moisture content during drying was estimated based on the initial determination and subsequent change in sample weight. Seed longevity at 45°C and 60% RH (p 50; days ± s.e.m.) after each drying treatment, provided by the best-fit model for each sample (DAA × temperature) for intermittent (E, F) or continuous drying (G, H), is also shown. For clarity, the control values (open circles joined by broken lines) in E and F are repeated in G and H, respectively.

Figure 3. Seed drying curves (A) at 15 (●), 30 (▲), 45 (♦) or 60°C (▼) over silica gel for rice cv. ‘Macassane’ seeds harvested at 34, 36 and 38 days after 50% anthesis (DAA) during 2016 wet season (WS). Interrupted lines show loss in moisture content for seeds dried throughout in the drying room (DR) control (15°C/15% RH; ○). Loss in moisture content during drying was estimated based on the initial determination and subsequent change in sample weight. Seed longevity (B) during storage at 45°C and 60% RH (p 50; days ± s.e.m.) after each drying treatment (DAA × temperature × drying duration), from both harvests, was estimated from the best-fit model for each sample.
Subsequent seed storage longevity
Significant differences in survival curves (P < 0.05) were apparent between some, but not all, seed lots harvested at different maturity stages and amongst drying treatments at each maturity stage. Therefore, longevity derived from the best-fit survival curves for each treatment combination are shown (Tables S1 and S2).
In both experiments, low-temperature drying (15°C) resulted in seeds with shorter longevity compared with high-temperature drying (Figs 2E–H and 3B). Nonetheless, drying seeds over MgCl2 at 15°C still led to an improvement in their subsequent storage longevity compared with drying at lower RH (15% RH) in the drying room (Fig. 2E–H). In ‘Macassane’, however, relative improvement between the drying room and silica gel treatments at 15°C depended on the duration of drying (Fig. 3B). Drying seeds in the drying room throughout (shown by broken lines) resulted in progressively greater longevity from 25 to 45 DAA in harvest A of IRGC 117265 (Fig. 2E,G), a small increase from 34 to 36 DAA but similar longevity at 36 and 38 DAA in ‘Macassane’ (Fig. 3B), or an increase from 25 to 35 DAA but then decline from 35 to 45 DAA in harvest B of IRGC 117265 (Fig. 2F,H).
In both varieties at all harvests, the higher the temperature of drying from 15 to 45°C the greater the longevity (Figs 2E–H and 3B). In ‘Macassane’, this was also the case with further increase in temperature from 45 to 60°C (Fig. 3B), whereas 60°C provided poorer, or sometimes similar, longevity compared with 45°C in IRGC 117265, but was always greater compared with drying at 15 or 30°C (Fig. 2E–H). There was a tendency for the more mature seed at harvest to show a slightly smaller benefit to longevity from high-temperature drying.
Within a temperature regime, the longevity of seeds of IRGC 117265 exposed to high temperature intermittently was broadly similar to the equivalent continuous treatment (Fig. 2E–H). At 30 and 45°C, continuous high-temperature drying provided slightly greater longevity than intermittent drying, but vice versa at 15 and 60°C (Fig. 2E–H).
The longevity of seeds of ‘Macassane’ dried over silica gel was determined for samples drawn after successive periods of drying. This showed that at the warmer temperatures of 45 and 60°C, and to a lesser extent at 30°C, the early periods of drying provided greater enhancement of longevity than the later periods (Fig. 3B). Comparing 60°C with progressively cooler temperatures, the warmer regimes continued to provide a benefit to longevity later in drying than was observed in cooler conditions.
Discussion
The moisture content of mature seeds at harvest depends on the ambient temperature and relative humidity and will affect the subsequent rate of viability loss if seeds are not dried ex planta. In tropical climates, RH conditions rarely fall below 80% and so seeds are harvested at moisture contents too high for safe storage, especially in the wet season. The genebank standards recommend drying seeds immediately after harvest to between 3 and 7% MC before long-term storage (FAO, 2013), because the rate of ageing is minimized at these values (Ellis et al., Reference Ellis, Hong and Roberts1989, Reference Ellis, Hong and Roberts1992; Ellis and Hong, Reference Ellis and Hong2006). However, the tolerance of orthodox seeds to desiccation and storage depends on the stage of maturity and the drying conditions, especially the rate of drying (Hay and Probert, Reference Hay and Probert1995).
In this experiment, all rice seeds were metabolically active (MC > 16.2%; Whitehouse et al., Reference Whitehouse, Hay and Ellis2015) and assumed to have reached mass maturity before harvest (Kameswara Rao and Jackson, Reference Kameswara Rao and Jackson1996a,Reference Kameswara Rao and Jacksonb). Hence they were still within the desiccation phase of seed development where seed quality traits can still be accrued (Galau et al., Reference Galau, Kjetill and Hughes1991; Angelovici et al., Reference Angelovici, Galili, Fernie and Fait2010; Chatelain et al., Reference Chatelain, Hundertmark, Leprince, Le Gall, Satour, Deligny-Pennick, Rogniaux and Buitink2012). In accordance with this, and similar to other studies (Ellis et al., Reference Ellis, Hong and Jackson1993; Kameswara Rao and Jackson, Reference Kameswara Rao and Jackson1996a,Reference Kameswara Rao and Jacksonb; Hay and Smith, Reference Hay, Smith, Smith, Linington, Dickie, Pritchard and Probert2003; Ellis, Reference Ellis2011), the longevity of seeds dried in the drying room (control; broken lines) increased the later the seeds were harvested i.e. 25–35 DAA (Fig. 2E,G) until, depending on the environmental conditions, longevity plateaued (Fig. 3B) or declined (Fig. 2 F,H). However, as previously shown by Whitehouse et al. (Reference Whitehouse, Hay and Ellis2015), longevity continued to increase ex planta when seeds from both cultivars and all harvests were exposed to initial drying at temperatures >15°C (Figs 2 and 3). The longevity of seeds dried intermittently or continuously for 3 days over MgCl2 (30% RH) continued to increase with drying at 30 to 45°C (Fig. 2E–H), with a further increase seen from 45 to 60°C in ‘Macassane’ seeds dried for 1 day over silica (Fig. 3B). The latter corresponds with the report that mature rice seeds can survive drying temperatures as high as 60°C (Lewis, Reference Lewis1950). As reported by Whitehouse et al. (Reference Whitehouse, Hay and Ellis2015), these results provide further confirmation that the quality of the seeds cannot be accurately predicted post mass maturity with respect to developmental time (DAA), as the pre-harvest environment (ambient temperature and humidity conditions, as well as the seed production environment) can restrict the seeds’ progression through development and through maturation drying in particular.
Research has shown that the environmental conditions experienced during development and maturation can affect the relative timings of developmental stages (Hirano, Reference Hirano1979; Tu et al., Reference Tu, McDonnell and Dirks1988; Olivares et al., Reference Olivares, Johnston and Calderon2009; Ellis, Reference Ellis2011; Martínez-Eixarch and Ellis, Reference Martínez-Eixarch and Ellis2015). For example, a warm seed production environment reduced the improvement in seed quality development that occurs subsequent to mass maturity in indica varieties of rice as the hotter temperatures enhanced the progression through development, which subsequently resulted in seeds that had not fully acquired maximum quality (Ellis et al., Reference Ellis, Hong and Jackson1993). Continuation of such developmental events can, however, continue ex planta if seeds are held at conditions similar to those they would naturally encounter in situ (Hay and Probert, Reference Hay and Probert1995; Hay, Reference Hay1997; Probert et al., Reference Probert, Adams, Coneybeer, Crawford and Hay2007). Rice seeds typically experience field temperatures between 27 and 30°C and so perhaps it is not surprising that subsequent longevity improved ex planta in response to drying at temperatures >15°C (Figs 2E–H and 3B) However, improvements in seed quality are not infinite and presumably there is a ‘maximum longevity’ that any developing cohort of seeds can attain. A typical developmental response would involve a continual increase in longevity before plateauing, and although increases in longevity would be slower at temperatures cooler than 45–60°C, all seed lots would be expected to eventually reach the same level of longevity. This was not observed in this study; rather, drying rate and subsequent storage longevity both increased with the increase in drying temperature, when seed lots were dried over silica gel (Fig. 3). This suggests that desiccation shock is more likely to account for the variation in longevity, whilst not ruling out a small contribution from continued development as it is likely that the two are connected, i.e. the desiccation shock accelerates the developmental events associated with increase in longevity during maturation drying. However, as some seed lots dried at a similar rate e.g. 15°C/15% RH and 45°C/30% RH (Fig. 2C,D) but with substantial differences in longevity (Fig. 2G,H), and all seed lots generally showed the greatest benefit to longevity within the first 2 h of drying, which was greater at higher temperatures (Fig. 3), there is clearly an effect of temperature rather than desiccation alone.
High temperatures are thought to induce stress responses within seeds, similar to that which triggers maturation drying, which is likely to promote the metabolic processes and protective mechanisms associated with desiccation (since they both represent stresses), including synthesis of late embryogenesis abundant (LEA) proteins and heat shock proteins (HSPs), accumulation of raffinose family oligosaccharides, and activation of anti-oxidant defence mechanisms (Vertucci and Farrant, Reference Vertucci, Farrant, Kigel and Galili1995; Kermode, Reference Kermode1997; Bailly et al., Reference Bailly, Leymaries, Lehner, Rousseau, Côme and Corbineau2004; Buitink and Leprince, Reference Buitink and Leprince2008; Leprince and Buitink, Reference Leprince and Buitink2010); high temperature will also increase the rate at which the above occur. This is supported by previous studies which provided evidence that the accumulation of soluble carbohydrates and heat stable proteins during development were associated with desiccation tolerance and potential longevity (Sinniah et al., Reference Sinniah, Ellis and John1998). However, these metabolic pathways and processes involved in the accumulation of longevity can be slowed/impaired when temperatures pass a critical limit (McDonald, Reference McDonald1999; Corbineau et al., Reference Corbineau, Gay-Matheiu, Vinel and Côme2002). Seed temperature during high-temperature drying would have increased towards the air temperature, albeit with some delay due to evaporative cooling as moisture was lost from the seeds; this may explain why seeds still benefit from drying at higher temperatures, up to 45°C, even after 3 days, but also why longevity was greatest when seeds were dried for 1 day at 60°C (Fig. 3B) but not after 3 days (Fig. 2E–H). Furthermore, it is possible that normal energy metabolism and enzyme activity may have been reinstated in metabolically active seeds (>16.2% MC) during the non-drying period, as reported by Corbineau et al. (Reference Corbineau, Gay-Matheiu, Vinel and Côme2002), which may explain the greater longevity observed in seeds dried intermittently at 60°C compared with seeds dried continuously (Fig. 2E–H).
Interestingly, prior to the 1990s, it was common practice to dry rice seeds intended for storage in the IRG at high temperature (45–50°C) and it was only after the publication of the FAO genebank standards (FAO, 1994), which recommended cool temperatures combined with relatively low humidity to dry seeds prior to storage, that the drying room facility was installed and operated at 15°C and 15% RH. The standards were derived based on the low MC limit, i.e. below which there is no further improvement in longevity (Ellis and Hong, Reference Ellis and Hong2006 and references therein), and the drying conditions necessary to achieve this equilibrium MC (without jeopardising seed quality). The FAO wanted a single, simple, safe procedure for diverse species from all locations worldwide and due to the vulnerability of some species to high temperatures, especially when mature seeds were at high MC (Nellist, Reference Nellist and Hebblethwaite1980; Cromarty et al., Reference Cromarty, Ellis and Roberts1982; McDonald and Copeland, Reference McDonald and Copeland1997), low temperature and humidity conditions were adopted (FAO, 1994, 2013). The genebank standards have recently been modified from 10–25°C and 10–15% RH (FAO, 1994) to a lower temperature (5–20°C) and broader humidity (10–25% RH) range (FAO, 2013) that further contradicts the results presented in this study which show drying rice seeds, harvested before maturity, at higher temperatures and at a higher humidity (30% RH) can significantly improve their subsequent storage longevity by more than that which occurred during subsequent development in planta. This could benefit farmers in resource-limited countries, particularly those in wet tropical regions, where it is difficult to dry large volumes of seeds to low MC. The quality of freshly harvested seeds could be maintained better (e.g. to the next growing season) by drying seeds to an intermediate MC using heated air.
To conclude, there is clear evidence that drying freshly harvested, high moisture content rice seeds under low temperature, low humidity conditions is not optimum for subsequent seed storage longevity. Rice seeds showed improvement in longevity in response to drying for a total of 3 days at temperatures greater than 15°C, up to at least 45°C (but drying at 60°C for more than 1 day may damage some seeds). Further studies could involve testing, in independent studies, the beneficial limits of high-temperature drying on rice seeds produced/regenerated in other climatic regions. This could have huge implications on how rice seeds are managed by genebanks globally to ensure maximum longevity when first placed into storage. However, future research should investigate high-temperature drying of fresh moist seeds more widely, addressing other economically important crops, especially those with poor seed storage longevity and where harvesting seeds at a range of maturities and hence, perhaps moisture content, is inevitable.
Financial support
This research received no specific grant from any funding agency, commercial or non-for-profit sectors.
Supplementary material
To view supplementary material for this article, please visit https://doi.org/10.1017/S0960258517000277.