1. Introduction
Technological advancements, cost reductions, and increasing policy targets for renewable energy continue to drive the growth of wind energy development (International Energy Agency, 2019). By the end of 2020, 743 gigawatts (GW) of wind power capacity were installed worldwide, with approximately 707 GW from land-based wind (LBW) energy and 35 GW from offshore wind (OSW) energy (Global Wind Energy Council, 2021). The United Nations Intergovernmental Panel on Climate Change (IPCC) reported that cutting global carbon emissions in half by 2030 will be required to limit warming by 1.5°C (IPCC, Reference IPCC, Masson-Delmotte, Zhai, Pörtner, Roberts, Skea, Shukla, Pirani, Moufouma-Okia, Péan, Pidcock, Connors, Matthews, Chen, Zhou, Gomis, Lonnoy, Maycock, Tignor and Waterfield2018). To meet the IPCC requirements, the International Renewable Energy Agency (IRENA) estimates that nearly 2015 GW of wind-generated electricity will be needed by 2030, or a three-fold increase for LBW and 10-fold increase for OSW (IRENA, 2019). Despite the benefits of wind energy, concerns for the direct and indirect environmental effects persist, including habitat alteration and mortality for certain species (e.g. Reference Adams, Kelsey, Felis and Pereksta2016 Friedenberg and Frick, Reference Friedenberg and Frick2021; Maxwell et al., Reference Maxwell, Kershaw, Locke, Conners, Dawson, Aylesworth, Loomis and Johnson2022). The interactions between wind energy and the environment can reduce renewable energy generation through project delays or abandonment, and changes to normal operations (Allison et al., Reference Allison, Diffendorfer, Baerwald, Beston, Drake, Hale, Hein, Huso, Loss, Lovich, Strickland, Williams and Winder2019). Balancing the benefits and concerns of wind deployment requires scientifically based, cost-effective monitoring and mitigation strategies that meet energy, economic, and conservation goals. The global nature of the wind industry, combined with the understanding that many affected species cross jurisdictional boundaries, highlight the need to collaborate at the international level (https://iea-wind.org/task34/).
In response to these global concerns, the International Energy Agency (IEA) Wind Technical Collaborative Program initiated Task 34 – Working Together to Resolve Environmental Effects of Wind Energy or WREN (https://tethys.pnnl.gov/about-wren; Sinclair et al., Reference Sinclair, Copping, May, Bennet, Warnas, Perron, Elmqvist and DeGeorge2018). Representatives of 13 countries from North America and Europe participate in WREN with the goal of informing the global community on the most pressing challenges and opportunities related to the environmental effects of wind deployment. The efforts conducted within WREN focus on (1) expanding international collaboration and knowledge transfer among government agencies, private industry, research institutions, and nongovernmental organizations, and (2) disseminating information on the state of the science for monitoring and mitigation practices (Sinclair et al., Reference Sinclair, Copping, May, Bennet, Warnas, Perron, Elmqvist and DeGeorge2018). To guide strategic planning for the next 3 years, WREN identified the need to collect feedback from the international community on environmental priorities and conducted the study discussed herein.
Horizon scanning is a systematic examination of emerging issues, involving potential threats and opportunities, and can be useful in developing strategies to address complex situations in science and decision-making (Esmail et al., Reference Esmail, Wintle, t Sas-Rolfes, Athanas, Beale, Bending, Dai, Fabinyi, Gluszek, Haenlein, Harrington, Hinsley, Kariuki, Lam, Markus, Paudel, Shukhova, Sutherland, Verissimo and Milner-Gulland2020; Sutherland et al., Reference Sutherland, Fleishman, Clout, Gibbons, Lickorish, Peck, Pretty, Spalding and Ockendon2019). There is no one-size-fits-all model for scans, and the time horizon can be short, medium, or long term (Cuhls et al., Reference Cuhls, Erdmann, Warnke, Toivanen, Toivanen, van der Giessen and Seiffert2015). However, a commonality across horizon scan studies is that organizers identify the objectives, then solicit and collate suggested questions or emerging issues from a large, diverse group of individuals, such as through the Delphi technique or other survey methods (Mukherjee et al., Reference Mukherjee, Huge, Sutherland, McNeill, Van Opstal, Dahdouh-Guebas and Koedam2015; Sutherland et al., Reference Sutherland, Fleishman, Mascia, Pretty and Rudd2011, Reference Sutherland, Dias, Dicks, Doran, Entwistle, Fleishman, Gibbons, Hails, Hughes, Hughes and Kelman2020). WREN carried out this assessment, using an approach modeled on horizon scans, to gather information from international stakeholders on key issues related to the environmental effects of both LBW and OSW development. The goal was to identify priority environmental issues associated with wind energy development within a 5-to-10-year time horizon.
2. Methods
2.1 Project scope
The vision for this project was to conduct a systematic (i.e. repeatable, inclusive, and transparent) assessment using feedback from the global wind energy and wildlife conservation community to identify priority environmental issues for LBW and OSW development. Given the global nature of the project, the intent was to elicit feedback from countries where wind energy development is already underway or being planned. The project's target was to achieve a prioritized list of LBW and OSW environmental issues, with specificity by stressor and receptor, and preferably by global regions.
2.2 Delphi method
This study used a horizon scan-like technique and adapted the Delphi method, which is widely used for identifying priorities with expert knowledge to inform ecological and biological conservation research (e.g. Sutherland, Reference Sutherland2006). The Delphi method ‘is a structured, anonymous and iterative questionnaire of a panel of “experts” or participants’ (Mukherjee et al., Reference Mukherjee, Huge, Sutherland, McNeill, Van Opstal, Dahdouh-Guebas and Koedam2015). The method is used for various purposes including gathering information on complex topics from a range of experts and geographic regions.
This study employed a modified Delphi method known as the ‘decision Delphi’ method to assess the environmental research issues deemed most important from the global wind energy and environmental community. The decision Delphi is a variation of the classical Delphi approach that focuses more on guiding future planning and prioritization (Hasson & Keeney, Reference Hasson and Keeney2011). The decision Delphi uses an initial open qualitative method and then narrows the process to a consensus. In this study, feedback from two rounds of questionnaires was consolidated, coded, and analyzed to identify environmental priorities associated with wind energy development.
2.3 Flow of study
The stages of the study included two questionnaires with associated analysis of results (stages 1 and 2) and final analysis to determine priority topics (stage 3; Figure 1).
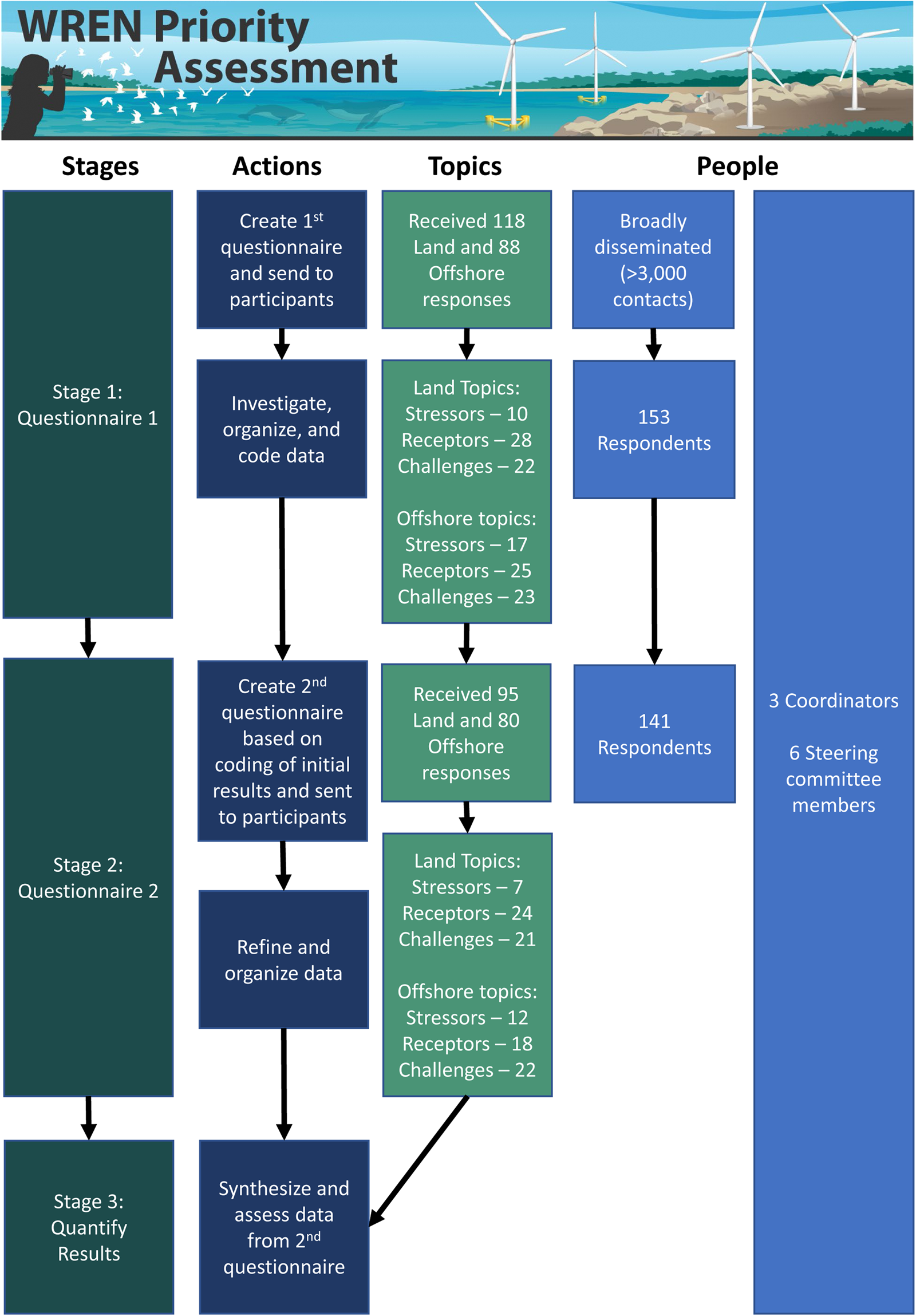
Figure 1. Flow of Working Together to Resolve Environmental Effects of Wind Energy (WREN) Priority Assessment Study. Graphic adapted from Esmail et al. (Reference Esmail, Wintle, t Sas-Rolfes, Athanas, Beale, Bending, Dai, Fabinyi, Gluszek, Haenlein, Harrington, Hinsley, Kariuki, Lam, Markus, Paudel, Shukhova, Sutherland, Verissimo and Milner-Gulland2020).
The study was conducted between January and December 2021. The first questionnaire was developed during January/February 2021 and was open online between March 9th and June 14th, 2021. The second questionnaire was developed during July 2021 and was open online between August 19th and October 26th, 2021. The final analysis (stage 3) was performed during November–December 2021, after the second questionnaire was closed.
2.3.1 Project team and participants
The study was facilitated by a project team that included coordinators and a technical steering committee. The coordinators included three researchers from the National Renewable Energy Laboratory in the United States, and the technical steering committee included representatives from Belgium, France, Norway, Portugal, Sweden, and the U.S. Department of Energy. The team included a broad set of expertise related to wind energy and wildlife, including representation from several countries, different sectors (i.e. government, research institutions, academia, and private industry), and both LBW and OSW expertise across various environmental topics. Questionnaires, coding, and analysis were developed by the coordinators and reviewed by the steering committee.
Outreach for the study involved several existing wind energy-environmental mailing lists, direct networks of the team, and key individuals in the field, based on literature reviews. Mailing lists used included Tethys Blast, Bats and Wind Energy Cooperative, National Wind Coordinating Collaborative, and the U.S. Offshore Wind Energy Synthesis of Environmental Effects Research project.
The original mail distribution encouraged participants to forward the information to their colleagues to increase dissemination. The approach prohibits an exact count of individuals who received an invite to participate; however, a minimum estimate of 3000 individuals were contacted. The second questionnaire was also sent to the entire mailing list and the outreach again encouraged participants to disseminate the form to their professional networks.
2.3.2 Questionnaires
The study approach included an initial questionnaire (stage 1) and a follow-up questionnaire (stage 2) to iteratively generate feedback and provide refinement of identified topics (Figure 1). Both questionnaires were sent to the full distribution list regardless of whether participants filled out the first questionnaire. For each questionnaire, participants were asked to submit answers for LBW, OSW, or both, depending on their expertise. The initial questionnaire asked participants to identify one to five priority environmental issues related to LBW and/or OSW development over the next 5–10 years in their country. IEA definitions were used for grouping countries by regions. Respondents were asked to indicate both a stressor and a receptor for each issue that they identified. Respondents with expertise in multiple countries were encouraged to fill out the questionnaire for each country. Although the identification of top issues was the focus of the initial questionnaire, several other questions were asked to provide greater context for analysis (Supplementary Table S1). When formulating the questionnaire, the coordinators drafted the initial questions that were reviewed by the technical steering committee. Several rounds of feedback from a small test group of 5–10 experts were conducted to ensure the questions were easy to interpret and the mechanics of the online form worked properly. The first questionnaire intentionally posed open-ended questions to avoid any potential influence from the project team.
The second questionnaire provided a refined list of topics identified in the first questionnaire. The questionnaire used a closed-question (i.e. choice from a list) format to facilitate ranking of priorities and allowed respondents to answer using drop-down menus. Participants were asked to identify up to three priorities from this refined list. The questionnaire remained focused on identifying the top environmental issues through the lens of a stressor–receptor relationship, with additional questions to elicit further feedback (Supplementary Table S2). To avoid bias, respondents had the opportunity to highlight topics not included in the refined list before submitting the questionnaire. The submissions were then ranked by the number of total responses received to determine the top issues identified.
2.3.3 Identifying priorities based on expert knowledge
The team's approach to issue identification was based on coding the responses from the first questionnaire. Each coordinator from the team developed individual codes based on the responses (both stressors and receptors) from the first questionnaire for both LBW and OSW. The codes were then consolidated into two final lists that were reviewed and approved by the steering committee. These codes were used as the options for priority environmental issues in the second questionnaire to enable quantifiable prioritization.
The initial questionnaire also asked participants to identify the primary considerations to implementing proven monitoring or mitigation approaches associated with a particular issue, such as societal, financial, political, regulatory, environmental, or other. These responses were also coded and included in the second questionnaire for ranking by participants.
Final questionnaire results were analyzed to identify prioritized stressors, receptors, stressor–receptor relationships, and challenges for both LBW and OSW. Respondents were asked to identify up to three priorities for LBW and/or OSW stressor–receptor relationships. Priority issues were grouped across all respondents without regard for the order in which individual respondents ranked priorities.
2.4 Limitations
The team conducted all outreach through email, and the questionnaires were conducted online. The questionnaire was only available in English which may have influenced responses. The geographic representation of respondents was also limited to the available mailing lists, the team's network of contacts, and willingness of participants from less-represented countries to respond.
3. Results and discussion
3.1 Representation of respondents
The questionnaire yielded responses from multiple regions, spanning a diversity of professional sectors and countries within the wind/environmental community. The first questionnaire received 153 responses across 23 different countries. Most respondents were from Europe (55%) and North America (31%), but responses were also received from Africa, Asia Pacific, Central and South America, and Eurasia (Supplementary Table S3).
In the first questionnaire, the sectors with the most responses included environmental consultancies (25%), research institutions (17%), government agencies (16%), academia (14%), wind farm developers and operators (11%), and nongovernmental organizations (8%). A few responses were also received from various other entities (9%), such as technology providers and turbine manufacturers. Seventy-two percent of respondents had at least 5 years of experience with the environmental effects of wind energy (n = 108). Forty-five percent of respondents had expertise with LBW, 23% with OSW, and 32% with both.
The second questionnaire received 141 responses from across 23 different countries. As in the first iteration, most feedback received was from Europe (60%) and North America (30%), with responses also received from Africa, Asia Pacific, Central and South America, and Eurasia (Supplementary Table S3). This skewed distribution may be caused by a combination of factors, including: (1) lack of contacts with the wind/environmental community that was less represented in this study, (2) locations of historic and current wind energy development, (3) level of research and monitoring effort addressing environmental issues within a region, and (4) distribution of the questionnaire only in English. Representation by professional sector and environmental area of expertise was similar to the first questionnaire. Forty-two percent of respondents had expertise with LBW, 34% with OSW, and 34% with both.
The expertise of respondents ranged from high-level, general topics to specific expertise with individual stressors. Respondents were allowed to identify multiple areas of expertise. The majority of respondents to both questionnaires (58–63%) indicated broad environmental expertise in biology/ecology. Individual stressors most represented by respondents included habitats (41–50%), terrestrial birds (40–50%), and ecosystems (40–47%). Stressor bias associated with respondents' expertise was minimized by analyzing stressor priorities for each receptor group identified, regardless of the total number of votes for a particular receptor group.
3.2 LBW environmental priorities
3.2.1 Initial open-ended, land-based responses
Numerous environmental issues were identified during the first stage of the study that were condensed for the second stage. The first questionnaire yielded 118 individual responses for LBW. These were consolidated into a set of stressors (n = 8) and receptors (n = 24) (Figure 2). Examples of stressors identified included attraction, turbine collisions, and displacement. Receptors were identified at various levels of specificity, ranging from broad categories, such as birds and bats, to smaller groups, such as eagles, raptors, grassland nesting birds, grouse, migratory songbirds, and soaring birds (includes storks, vultures, condors, and cranes). Specific bat-related receptors included cave-hibernating and tree-roosting bats.
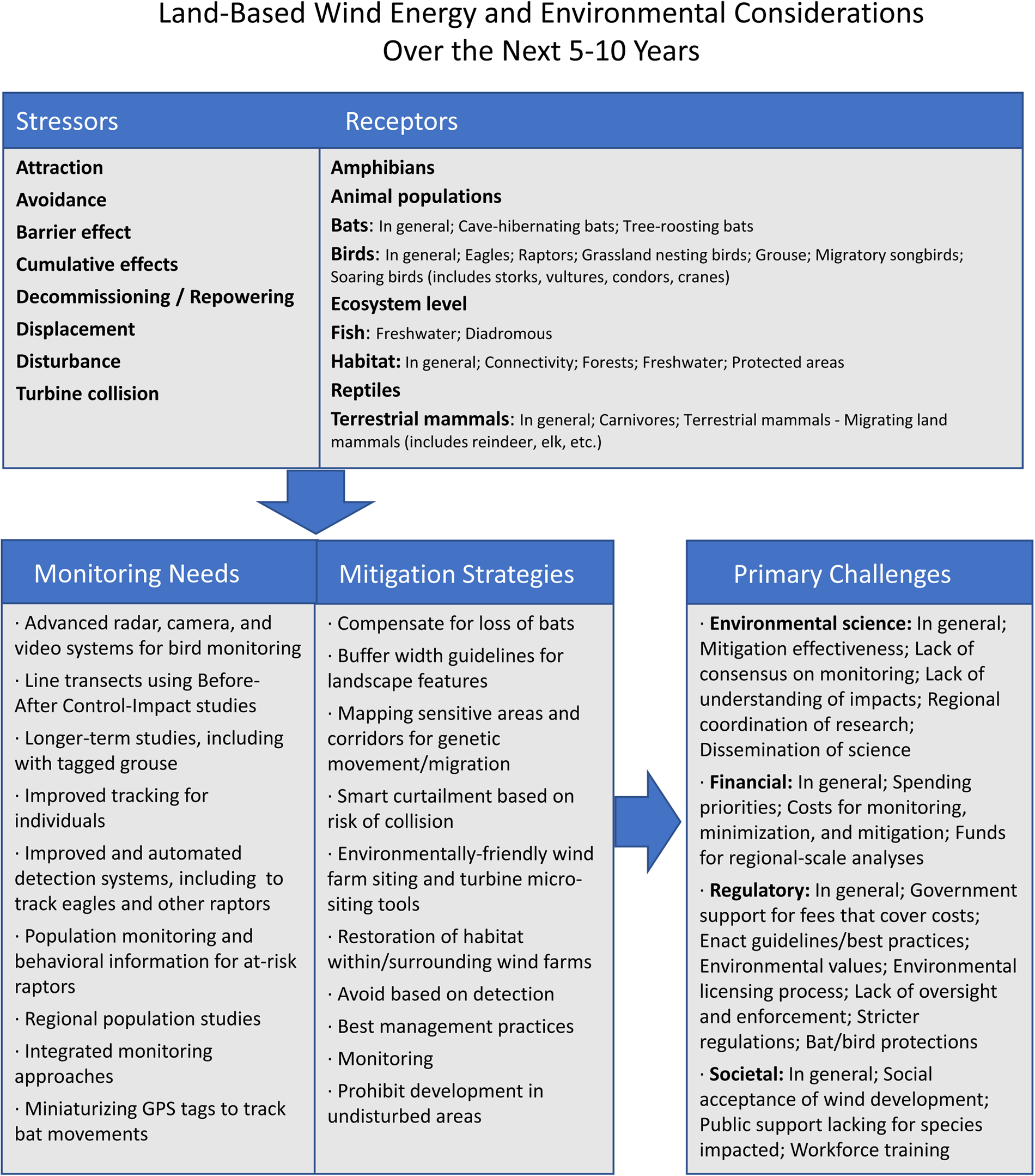
Figure 2. Summary of land-based responses from the first questionnaire, with further grouping of categories (ordered alphabetically; bold font) for inclusion in the second questionnaire.
A range of land-based monitoring needs and mitigation measures were identified during the first stage of the study (Figure 2). Several respondents identified the need for advanced technologies, such as radar, camera systems, and GPS tags for improved monitoring. The need for longer-term studies, including those for grouse species, was also identified. Mitigation measures identified included actions to avoid, minimize, and compensate for environmental impacts. For avoidance, the need to develop environmentally friendly wind farm and turbine siting tools was acknowledged. Minimization measures included the use of smart curtailment strategies. Compensating for mortality, such as for loss of bats, or for habitat loss/alteration was recognized.
3.2.2 Land-based stressors, receptors, and challenges
Following the second questionnaire, the team collated and analyzed the results from all LBW respondents (n = 95). Stressor priorities were analyzed for each receptor group identified by respondents, including birds, bats, ecosystems, and habitat, as well as other receptors identified less often (i.e. animal populations and terrestrial mammals). The land-based stressor with the most votes was turbine collisions (42%), followed by cumulative effects (26%; Figure 3). Within the bird category, raptors (including eagles) and ‘birds in general’ received the most votes with 35% of responses each, followed by soaring birds (14%), grassland nesting birds (7%), migratory songbirds (6%), and grouse (3%). Within the bat category, ‘bats in general’ (77%) and tree-roosting bats (22%) received the most votes. The greatest challenges to monitoring and mitigation approaches were associated with environmental science (36%) and regulation (34%), specifically related to the effectiveness of mitigation measures and costs for monitoring, minimization, and mitigation.

Figure 3. Land-based responses from second questionnaire for environmental stressors identified for each receptor group.
The largest number of LBW responses were received from Europe and North America (Figure 4). For Europe, turbine collision risk was the highest-ranked stressor (48%), followed by cumulative effects (24%). Similarly, for North America, turbine collision risk and cumulative effects were the highest-ranked stressors (39 and 27%, respectively). For Europe, the next highest stressor was avoidance (10%), whereas in the United States, the next highest stressor was displacement (18%). Some responses were also received from other regions, including Asia Pacific, Africa, Central and South America, and Eurasia. For all of these other regions, the highest-ranked stressor was turbine collision. Cumulative effect was the second highest-ranked stressor across all regions, except in Africa where it tied with avoidance and in Central and South America where barrier effect was the second highest-ranked stressor.

Figure 4. Highest ranked land-based environmental stressors by region, ordered by votes (separation by a comma denotes tied votes).
3.2.3 Discussion of land-based priorities
The primary environmental concern for LBW, identified by the study, was turbine collision risk for birds and bats (Figure 3). This is primarily driven by direct mortality caused by collision strikes of raptors and migratory bats (Allison et al., Reference Allison, Diffendorfer, Baerwald, Beston, Drake, Hale, Hein, Huso, Loss, Lovich, Strickland, Williams and Winder2019; Barclay et al., Reference Barclay, Baerwald and Rydell2017; de Lucas & Perrow, Reference De Lucas and Perrow2017). Many of the species impacted have small population sizes and low growth rates (Barclay & Harder, Reference Barclay and Harder2003; Beston et al., Reference Beston, Diffendorfer, Loss and Johnson2016). For raptors, such as golden eagles (Aquila chrysaetos) and white-tailed eagles (Haliaeetus albicilla), there are relatively few collisions compared to other bird species, but this small impact compounded with other stressors, such as electrocution with power lines, can affect population sizes (Allison et al., Reference Allison, Cochrane, Lonsdorf and Sanders-Reed2017; May, Reference May2015). Migratory bats, including hoary bats (Lasiurus cinereus) and noctule bats (Nyctalus noctula), often comprise the highest proportion of fatalities at wind farms (Arnett & Baerwald, Reference Arnett and Baerwald2013; Rydell et al., Reference Rydell, Bach, Dubourg-Savage, Green, Rodrigues and Hedenstrom2010). Although population data for these species are limited, it is possible that the current fatality rate is unsustainable (Friedenberg & Frick, Reference Friedenberg and Frick2021).
Cumulative effect was the second highest-ranked LBW stressor in the study, with application to birds, bats, ecosystems, habitat, and animal populations (Figure 4). By definition, cumulative effects result from the incremental impact of an action when added to other past, present, and reasonably foreseeable future actions (Council on Environmental Quality, 1997). Cumulative effects account for both direct and indirect effects and span across the construction, operational, and decommissioning phases of a wind farm. The effects of wind energy development may also be combined with habitat loss from other land-use practices, invasive species, and climate change, as is the case for many grassland bird species, specifically prairie grouse (LeBeau et al., Reference LeBeau, Howlin, Tredennick and Kosciuch2020). Given the projected growth for LBW over the next decade, cumulative effects assessment will continue to play an important role in development and continued evaluation, and potentially mitigation, will be necessary (May et al., Reference May, Middel, Stokke, Jackson and Verones2020, Reference May, Jackson, Middel, Stokke and Verones2021).
Indirect effects of LBW for all receptors are another concern (Figure 4). These include behavioral responses of an individual or species to the presence of wind turbines or farms. Behavioral responses can occur at various scales, including the wind farm (macro), within the wind farm (meso), and at the wind turbine (micro) (Cook et al., Reference Cook, Humphreys, Masden and Burton2014). These include behaviors, such as avoidance and displacement, that can affect survival and reproduction if individuals alter their normal activity, use, or flight patterns to maneuver around the wind farm (May, Reference May2015). Another type of behavioral response, attraction, can increase collision risk if individuals spend more time near the rotor-swept area (Cryan & Barclay, Reference Cryan and Barclay2009; Guest et al., Reference Guest, Stamps, Durish, Hale, Hein, Morton, Weaver and Fritts2022; Kunz et al., Reference Kunz, Arnett, Erickson, Hoar, Johnson, Larkin, Strickland, Thresher and Tuttle2007; Thaxter et al., Reference Thaxter, Ross-Smith, Bouten, Clark, Conway, Masden, Clewley, Barber and Burton2019). Habitat alteration can also indirectly affect species if the development of a wind farm results in the loss of foraging, roosting, or mating resources (Watson et al., Reference Watson, Kolar, Ferrer, Nygård, Johnston, Hunt, Smit-Robinson, Farmer, Huso and Katzner2018).
3.3 OSW environmental priorities
3.3.1 Initial open-ended, offshore responses
For OSW, the first questionnaire yielded 88 individual responses, which the team consolidated into a set of stressors (n = 13) and receptors (n = 22) (Figure 5). The stressors included all of those identified for LBW, plus several offshore-specific stressors, including mooring lines and entanglement (e.g. floating wind turbines), underwater noise, and vessel collisions. Receptors also included some identified for LBW, such as birds and bats, but additionally included those exclusive to OSW, including marine mammals and fish. Marine mammals were categorized by respondents into specific groups, including cetaceans, whales, dolphins, and porpoises.

Figure 5. Summary of offshore responses from the first questionnaire with further grouping of categories indicated (ordered alphabetically; bold font) for inclusion in the second questionnaire.
A range of offshore monitoring needs and mitigation measures were identified during the first stage of the study (Figure 5). Several respondents noted the need for monitoring of migratory populations, as well as behavior of marine life and distributions (e.g. telemetry studies). Specific recommendations included monitoring during offshore pilot projects, improved collection of underwater benthic imagery, and monitoring existing decommissioning operations to formulate best practices. Mitigation measures recognized included effective use of marine spatial planning, including to avoid cumulative effects, informed choice of substrates for new physical structures, such as for scour protection, and where necessary, effective curtailment based on modeling. Overall, as for LBW, the need for using environmentally friendly wind farm siting tools was identified.
3.3.2 Offshore stressors, receptors, and challenges
Following the second questionnaire, the team collated and analyzed results from all offshore respondents (n = 80), resulting in development of priorities based on overall rankings (Figure 6). Stressor priorities were analyzed for each receptor group identified by respondents, including birds, marine mammals, ecosystems, and habitat, as well as other receptors identified less often (i.e. fish, food webs, bats, migrating animals, and hydrodynamics). The offshore stressor with the most votes was cumulative effects (29%), followed by turbine collisions (15%), displacement (14%), and underwater noise (14%). Within the bird category, seabirds received the most votes (53%), followed by birds in general (34%) and migratory birds (13%). Within the marine mammal category, marine mammals in general received the most votes (60%), followed by cetaceans (29%), whales (8%), and dolphins and porpoises (3%). The greatest offshore challenge to monitoring and mitigation approaches was related to environmental sciences (44%), specifically the lack of data related to impacts, lack of baseline data, and effectiveness of mitigation measures. The next highest challenge was associated with the regulatory arena (28%).

Figure 6. Offshore responses from second questionnaire for environmental stressors identified for each receptor group.
Similar to LBW, the largest number of responses for OSW were received from Europe and North America and offer the best opportunity for regional comparisons (Figure 7). Cumulative effect was the highest-ranked stressor in both Europe and North America (31 and 25% of responses, respectively). Some differences were observed in the next-highest rankings by region. For Europe, the next-highest stressor was underwater noise (16%), whereas in the United States, the next-highest stressor was displacement (21%). A small number of responses were received from other regions, including Central and South America and Africa, with none from the Asia Pacific or Eurasia. For Central and South America and Africa, cumulative effect was similarly identified along with several other stressors.

Figure 7. Highest ranked offshore environmental stressors by region, ordered by votes (separation by a comma denotes tied votes).
3.3.3 Discussion of offshore priorities
For OSW, the identification of cumulative environmental effects as a top-priority stressor (Figure 7) is consistent with current international targets to increase deployment of large offshore projects, often with bigger turbines, and the associated need for a holistic understanding of potential effects on the marine ecosystem. Cumulative effects were the top-priority stressor in the study, in application to multiple receptor groups, including birds, marine mammals, ecosystems, habitat, and several other receptors. While cumulative effects have been previously identified as an environmental issue, the anticipated large-scale roll-out of offshore wind in the next 30 years, including both fixed-bottom and floating wind installations, is likely to cause ecosystem-scale cumulative effects which are currently understudied. Depending on the region, various marine life receptors (e.g. seabirds, marine mammals, fish, and sea turtles) may be impacted during the life cycle of an OSW project, including site surveys during preconstruction, pile driving during construction, presence of infrastructure throughout operations, and increased boat traffic during all project phases (Goodale & Milman, Reference Goodale and Milman2019). The complexities and uncertainties surrounding cumulative effects of OSW farms have caused significant delays during the consenting process in Europe and the United States (e.g. Willsteed et al., Reference Willsteed, Jude, Gill and Birchenough2018). The first U.S. federal OSW farm, the Vineyard Wind project, faced delays due to a decision in 2019 to pause the environmental assessment process to allow time for a more comprehensive review of the cumulative impacts of OSW development along the Atlantic Coast, which was completed in 2021.
Improved practices are being identified for cumulative impact assessments that better support regional marine management and marine spatial planning (Piet et al., Reference Piet, Tamis, Volwater, de Vries, van der Wal and Jongbloed2021). In Europe, one such approach is the Common Environmental Assessment Framework (CEAF), with involvement from those countries bordering the North Sea. The framework has been developed as an instrument for assessing cumulative impacts of offshore renewable energy, particularly wind energy, and has been tested for seabirds and marine mammals (e.g. Leemans et al., Reference Leemans, Middelveld and Gyimesi2019). Also in the North Sea, a spatial and temporal analysis has recently been performed on the cumulative environmental effects of multiple OSW farms in the basin (Gușatu et al., Reference Gușatu, Menegon, Depellegrin, Zuidema, Faaij and Yamu2021). Potential impacts were assessed on selected seabed habitats, fish, seabirds, and marine mammal species, spanning OSW development during 1999–2050 across the North Sea basin. In the United States, frameworks have been developed for cumulative impact assessments for OSW scenarios (e.g. NYSERDA, 2017), with cumulative exposure assessments for particular species, such as seabirds along the U.S. Atlantic coast (e.g. Goodale et al., Reference Goodale, Milman and Griffin2019).
For birds, turbine collision risk and displacement at OSW farm developments were also voted among the top stressors (Figure 6). In the early 2000s, there was a recognized lack of empirical evidence from OSW farms in Europe with a need to develop a deeper understanding of how seabirds behave within and around farms, including gathering empirical evidence to improve collision risk models for key seabird species (Exo et al., Reference Exo, Huppop and Garthe2003). To assess this issue, a joint-industry project was initiated in Europe in 2014 called The Offshore Renewables Joint Industry Programme (ORJIP) Bird Collision Avoidance (BCA) study (Skov et al., Reference Skov, Heinänen, Norman, Ward, Méndez-Roldán and Ellis2018). In the United States, early assessments have been undertaken to understand collision and displacement vulnerability among marine birds at OSW farms, and avian survey guidance has been developed by the government (e.g. Adams et al., Reference Adams, Kelsey, Felis and Pereksta2016; BOEM, 2020). As indicated by this study there remains an urgent need for more research on migratory bird and seabird behavior around OSW farms to inform evidence-based planning decisions, within ecologically sustainable limits (Perrow, Reference Perrow2019).
Underwater noise was also identified through this study as an important emerging environmental effect associated with OSW development, with considerations for marine mammals, fish, other marine animals, and the broader ecosystem (Figure 6). Considerations associated with underwater noise and OSW development have been the subject of several recent reviews (ICF, 2021; SEER, Reference SEER2022; Tsouvalas, Reference Tsouvalas2020). These reviews are largely focused on the noise pollution during construction, which is generated by the driving of the large piles which support OSW structures. Improvements are being made to comprehensive state-of-the-art computational methods to predict the underwater noise emission by the installation of foundation piles offshore including the available noise mitigation strategies. Future challenges for minimizing the effects of underwater noise include understanding the implications of the increasing size of wind turbines, emerging pile driving technologies, and cumulative effects across wind farm stages. While the risk of underwater noise to marine life from the non-construction phases of wind farm development (e.g. site surveys, operations, and maintenance) is considered to be lower, further monitoring is still needed to help fill existing research needs and gaps in understanding (e.g. Tougaard et al., Reference Tougaard, Hermannsen and Madsen2020). Future understanding of underwater noise effects should be considered across a broad range of marine life species, including marine mammals, fish, and sea turtles.
4. Conclusions
A horizon scan-like approach with the Delphi technique was effective in identifying international priorities for environmental issues associated with wind energy development. A total of 294 responses from 28 countries were collected across both questionnaires, with representation from a wide variety of professional sectors and environmental expertise across LBW and OSW. Based on the networks available, Europe and North America were most highly represented, with more modest feedback from other regions, including Africa, Asia Pacific, Central and South America, and Eurasia. Future wind-environmental efforts should focus on building stronger relationships from these other regions to provide a broader global understanding of wind-environmental issues. This study builds on past horizon scanning and prioritization efforts associated with wind energy development (e.g. Köppel et al., Reference Köppel, Biehl, Wachendörfer and Bittner2019; Piorkowski et al., Reference Piorkowski, Farnsworth, Fry, Rohrbaugh, Fitzpatrick and Rosenberg2012), by taking an international approach to elicit feedback from as many countries as possible and specifically addressing environmental issues associated with both LBW and OSW development.
While this study largely analyzed land-based and offshore environmental priorities independently, there are several similarities across the findings that are indicative of interests across the larger wind energy community. For both LBW and OSW, cumulative effects, turbine collisions, and indirect effects (e.g. displacement and avoidance) were identified among the top stressors by respondents. As well, birds, bats, ecosystems, and habitat are considerations for wind energy buildout across the larger landscape/seascape perspective. As wind energy deployment continues to grow, cumulative effects will require greater attention for both land and sea development and across international boundaries, given wind energy can effect several migratory species. A common challenge identified for both LBW and OSW associated with monitoring and mitigation approaches was related to the effectiveness of mitigation measures (e.g. detection and deterrence technologies) and costs for monitoring, minimization, and mitigation.
The results of this study are broadly valuable to the international scientific community and decision-makers in emerging markets. For example, the priorities identified can help to inform wind energy licensing and permitting processes and may help to direct research, monitoring, and mitigation funds to those issues identified as most important. From an international perspective, both LBW and OSW development are projected to increase (IRENA, 2019). Based on this study, there remains a major focus on the potential effects of wind turbines on collision risk for volant species and cumulative effects at the species level and ecosystem level. As wind turbine dimensions increase in height and rotor-swept area, and the number of farms in a region increases, new methods and technologies will be required to monitor and mitigate environmental effects. These next-generation tools are necessary to assess the emerging environmental effects associated with future technologies (e.g. larger turbines and floating turbines), considerations for new species in frontier regions, and the cumulative effects for multiple projects at a regional scale. In addition, indirect, and therefore less visible, environmental effects from wind energy development will need further consideration as deployment continues to expand (e.g. disturbance caused by underwater noise and associated behavioral responses). Collectively, scientists, decision-makers, and industry will need to address the major environmental science and regulatory challenges to monitoring and mitigation associated with wind energy development, including identifying funding strategies, targeting research opportunities, and evaluating the effectiveness of mitigation measures.
Supplementary material
The supplementary material for this article can be found at https://doi.org/10.1017/sus.2022.14.
Acknowledgments
The authors are grateful to all the respondents to the questionnaires, spanning many countries, for their time and perspectives. We also thank Karin Sinclair, Jocelyn Brown-Saracino, Naomi Lewandowski, and Joy Page for their contributions. Anonymous reviewers provided thoughtful feedback which significantly improved the quality and content of the manuscript.
Author contributions
REG, EG, and CH constituted the core implementation, writing, and analysis team. LC, MM, RM, DN, and BR served on the steering committee and provided feedback on methods, analyses, and edits to the manuscript. All authors have read and agreed to the published version of the manuscript.
Financial support
This work was supported by the National Renewable Energy Laboratory, operated by Alliance for Sustainable Energy, LLC, for the U.S. Department of Energy (DOE) under Contract No. DE-AC36-08GO28308. Funding provided by the DOE Office of Energy Efficiency and Renewable Energy Wind Energy Technologies Office. The views expressed in the article do not necessarily represent the views of the DOE or the U.S. Government. The U.S. Government retains and the publisher, by accepting the article for publication, acknowledges that the U.S. Government retains a nonexclusive, paid-up, irrevocable, worldwide license to publish or reproduce the published form of this work, or allow others to do so, for U.S. Government purposes.
Conflict of interest
All authors declare no conflict of interest.