There is substantial literature on fatty acid compositions in mammalian heart, but little is known about their oxygenated bioactive metabolites called oxylipins. The most well-studied class of oxylipins are the eicosanoids generated by cyclo-oxygenase, lipoxygenase and cytochrome P450 oxygenase activities from arachidonic acid (ARA, 20 : 4n-6); these bioactive lipids are the major mediators of ARA effects in the body(Reference Gabbs, Leng and Devassy1, Reference Funk2). Oxylipins also are synthesised from other PUFA such as EPA, DHA, α-linolenic acid (ALA, 18 : 3n-3) and linoleic acid (LA, 18 : 2n-6). Oxylipins have many biological functions, and several effects of free oxylipins on the heart in health and disease have been shown(Reference Li, Liu and Qiu3, Reference Caligiuri, Parikh and Stamenkovic4). For example, ARA-derived PGE2 modulates cardiac contractility depending on which receptor it activates(Reference Gu, Xu and Zhu5), 19-hydroxy-eicosatetraenoic acid (19-HETE) reduces cardiomyocyte hypertrophy(Reference Elkhatali, El-Sherbeni and Elshenawy6), 20-HETE and epoxy-eicosatrienoic acids (EpETrE) induce cardiac hypertrophy(Reference Alsaad, Zordoky and Tse7), EpETrE prevent lipopolysaccharide-induced cardiac dysfunction(Reference Dai, Wu and He8) and 5-, 12- and 15-HETE induce cellular hypertrophy in human ventricular cardiomyocytes(Reference Maayah and El-Kadi9). Oxylipins derived from other PUFA also have effects on the heart, such as EPA-derived 18-hydroxy-eicosapentaenoic acid (18-HEPE), which is anti-inflammatory in cardiac fibroblasts(Reference Endo, Sano and Isobe10), and DHA-derived 13,14-epoxy-docosapentaenoic acid (13,14-EpDPE), which potently dilates porcine coronary microvessels(Reference Ye, Zhang and Oltman11). Little is known about the existence and functions of heart oxylipins derived directly from 18-carbon PUFA (LA and ALA), although there is some evidence that LA affects heart function indirectly by altering ARA-derived oxylipins(Reference Hoffmann, Mentz and Blass12). LA-derived oxylipins such as 13-hydroxy-octadecadienoic acid have been shown to attenuate platelet adhesion to endothelial cells(Reference Tloti, Moon and Weston13), and dihydroxy-octadecenoic acids may induce oxidative stress and proinflammatory events in vascular endothelial cells(Reference Viswanathan, Hammock and Newman14), but direct effects of LA oxylipins on the heart have not been reported. Furthermore, there is little information on the heart oxylipin profile in the literature(Reference Willenberg, Rund and Rong15). Thus, the first objective of the present study was to provide a comprehensive profile of the free oxylipins in the rat heart.
Dietary PUFA effects on oxylipins in different tissues havehistorically been predicted based on their effects on tissue PUFA composition. However, comparisons of PUFA and oxylipin profiles in rat kidney, liver and adipose reveal that oxylipins do not always reflect their parent PUFA levels. Further, tissue oxylipin levels are not only modulated by dietary alterations in their direct fatty acid precursors(Reference Pinto, Sanders and Kendall16–Reference Mendonca, Cayer and Pauls21) but also can be altered by dietary fatty acids that are not their direct precursors, such as ARA oxylipins being reduced in the heart by dietary n-3 fatty acids(Reference Abeywardena and Charnock22), or being increased by dietary LA(Reference Hoffmann, Mentz and Blass12). Thus, the second objective of the present study was to examine the effect of differing dietary oils on the heart oxylipin profile.
Oxylipin profiling in the heart also may be relevant to further understanding of sex differences in the normal and diseased heart(Reference Caligiuri, Parikh and Stamenkovic4). Previous oxylipin profiling studies demonstrated higher levels of renal, hepatic and serum oxylipins in male rats(Reference Leng, Winter and Aukema23, Reference Leng, Winter and Aukema24). In several adipose depots, sex differences depended on the diet: for example, rats provided a diet rich in DHA had higher adipose oxylipins in males, while those provided diets enriched in either ALA or EPA had higher oxylipins in females(Reference Mendonca, Cayer and Pauls21). There are few data on sex differences in heart oxylipins, but some studies indicate that some individual ARA-derived oxylipins may be higher in females in vascular tissue(Reference Jun, Chen and Pace25, Reference Calkin, Sudhir and Honisett26). Therefore, the third objective of the present study was to examine sex differences in the heart profile.
To achieve these three objectives, female and male rats were provided diets that differed only in oil composition so that each test diet compared with control had higher levels of specific PUFA. Rats were used since collection of hearts from humans consuming specific diets is not possible, and rats are a good model of human lipid metabolism for a whole body approach.
Materials and methods
Animals and diets
A total number of seventy-two female and male Sprague–Dawley weanling rats were randomly provided six different diets for 6 weeks as described in detail in references(Reference Mendonca, Cayer and Pauls21, Reference Leng, Winter and Aukema23, Reference Leng, Winter and Aukema24), which describe diet and sex effects on kidney, liver, serum and adipose oxylipins in these rats. Briefly, the diets were based on the standard American Institute of Nutrition (AIN)-93G diet but had 10 g oil instead of 7 g oil per 100 g diet. Oil blends were designed for each diet so that the control diet had adequate levels of the essential fatty acids, ALA and LA, and high levels of MUFA. In the test diets, MUFA were replaced with oils high in ALA, EPA, DHA and LA so that these diets contained 3 g (per 100 g diet) more of each of these PUFA, respectively, compared with the control diet. The LA + ALA diet also contained 3 g more LA per 100 g diet and had additional ALA so that the LA:ALA ratio was similar to the control diet. The percentage of energy from LA and ALA in all diets except the ALA diet is within the range of human consumption patterns. The higher amounts of ALA, EPA and DHA in the ALA, EPA and DHA diets, respectively, compared with control, were matched with the increased amount of LA in the LA compared with control diet, so that direct comparisons could be made between PUFA. However, the levels of these n-3 PUFA in the ALA, EPA and DHA diets are not commonly achieved in the human population. The levels of unsaturated fatty acids and SFA were similar across all diets.
The rats were housed individually, weighed weekly and the dietary intervention was carried out for all rats during the same 6-week period. Rats were anaesthetised using isofluorane before termination in random order and harvested heart tissues were weighed, immediately frozen in liquid N2 and stored at −80°C until analysis. All animal procedures were performed according to the Canadian Council for Animal Care guidelines and approved by the University of Manitoba Animal Care Committee.
Free oxylipin analysis
Hearts were rinsed with ice-cold Tyrode’s salt (pH 7·6) solution to remove blood before homogenisation of the whole heart in fresh Tyrode’s solution. An optimum amount of heart homogenate (400 µl containing 70 mg tissue) for oxylipin quantification was determined from a dose–response curve, and analysis of oxylipins was performed by HPLC/MS/MS as described(Reference Monirujjaman, Devassy and Yamaguchi20, Reference Leng, Winter and Aukema23). Briefly, deuterated internal standards (Cayman Chemicals) were added to sample homogenates containing antioxidants and adjusted to pH < 3·0. Strata-X-SPE (Phenomenex) columns preconditioned with methanol followed by pH 3·0 water were used for solid phase extraction. After column loading and washing, free oxylipins were eluted with 100 % methanol. Samples obtained were dried and re-suspended in the mobile phase (water/acetonitrile/formic acid, 70/30/0·02, v/v/v) for oxylipin analysis by HPLC/MS/MS (QTRAP 6500; Sciex). Quantification of oxylipins was performed using the stable isotope dilution method(Reference Hall and Murphy27). Oxylipins screened but below the level of detection (<3 times above baseline) and those detected but below the level of quantification (>3 to <5 times above baseline) are provided in online Supplementary Table S1a and 1b, respectively. Further details of all oxylipins scanned, mass transitions, internal standards, standard curve slopes and retention times are provided in references(Reference Monirujjaman, Devassy and Yamaguchi20, Reference Leng, Winter and Aukema23).
Fatty acid analysis
Total lipids were extracted from 250 µl of the heart homogenate and fatty acids were analysed as described(Reference Caligiuri, Love and Winter19, Reference Sankaran, Lu and Bankovic-Calic28). Briefly, after solvent extraction of total lipids, total phospholipid (PL) and neutral lipid (NL) were separated by TLC (heptane–isopropyl ether–acetic acid, 60:40:3, by vol.)(Reference Aukema, Yamaguchi and Takahashi29). Fatty acids were methylated using methanolic H2SO4, extracted in hexane and analysed by GC as described(Reference Aukema and Holub30, Reference Fair, Ogborn and Weiler31). Analyses of both fatty acids and oxylipins were performed in random order.
Statistical analysis
With six rats in each diet/sex group (total of seventy-two rats), this study had a power of 0·8 to detect an effect size of 0·45 at a significance level of P < 0·05 (G*Power Software version 3.1.9.2). Statistical analysis was performed using SAS Software Version 9.3 (SAS Institute Inc). The Shapiro–Wilk test was performed to test for normality, followed by one- or two-way ANOVA if data were distributed normally. The Kruskal–Wallis test was performed when data were not normal even after transformation. Tukey’s test was used for post hoc analyses. Observations greater than the mean ± 3 sd of a group were removed as outliers. All data are reported as means with their standard errors.
Results
Overall oxylipin and fatty acid distributions
Out of 164 oxylipins scanned, seventy-five were detected and quantified in the rat hearts. Approximately two-thirds of the oxylipins were derived from n-6 PUFA; two-thirds of these were formed from ARA. Approximately half of the remaining n-3 PUFA-derived oxylipins were formed from DHA, one-third were from EPA and one-fifth were from ALA (online Supplementary Tables S2 and S3).
The proportions of oxylipin mass did not necessarily reflect PUFA mass proportions in either the PL or NL fractions. For example, in the control group, the proportion of LA and ARA oxylipins was approximately two-thirds and one-third, respectively, while the proportion of LA and ARA was almost equally split between these two PUFA in the PL fraction and skewed more towards LA in the NL fraction (Fig. 1(A)). Further, the distributions of oxylipin and PUFA mass also were different with the different dietary oil treatments; examples of the DHA and LA groups are shown in Fig. 1(B) and (C), respectively; values for all diets can be found in online Supplementary Table S4.

Fig. 1. Distribution of heart oxylipin and PUFA mass in phospholipid (PL) and neutral lipid (NL) fractions in rats provided the control (A), DHA (B) and linoleic acid (LA) (C) diets. Data shown are for combined data from female and male rats. Separate female and male data for all diet groups are provided in online Supplementary Table S4. ARA, arachidonic acid; ALA, α-linolenic acid.
Effects of dietary PUFA on n-3-derived oxylipins
At the end of the feeding period, there were no differences in body or heart weights between the dietary treatments. Heart and body weights were higher in males, while heart weights relative to body weight were higher in females (online Supplementary Table S5). Compared with the control group, ALA and DHA oxylipins were higher only in hearts from rats given diets that were higher in their specific precursor PUFA (Fig. 2(A); online Supplementary Table S2). On the other hand, EPA oxylipins were higher not only in EPA rats but also in rats given ALA and DHA diets. ALA and DHA diets did not increase EPA oxylipins as much as with the EPA diet, however. The LA + ALA diet with higher levels of ALA than the control diet, but lower levels than in the ALA diet, also increased ALA and EPA oxylipins when compared with the control diet, but fewer oxylipins were affected and the effect was smaller than in hearts from rats given the ALA diet. The LA diet did not affect the n-3 oxylipin levels in the rat heart, except for 13-hydroxy-octadecatrienoic acid.
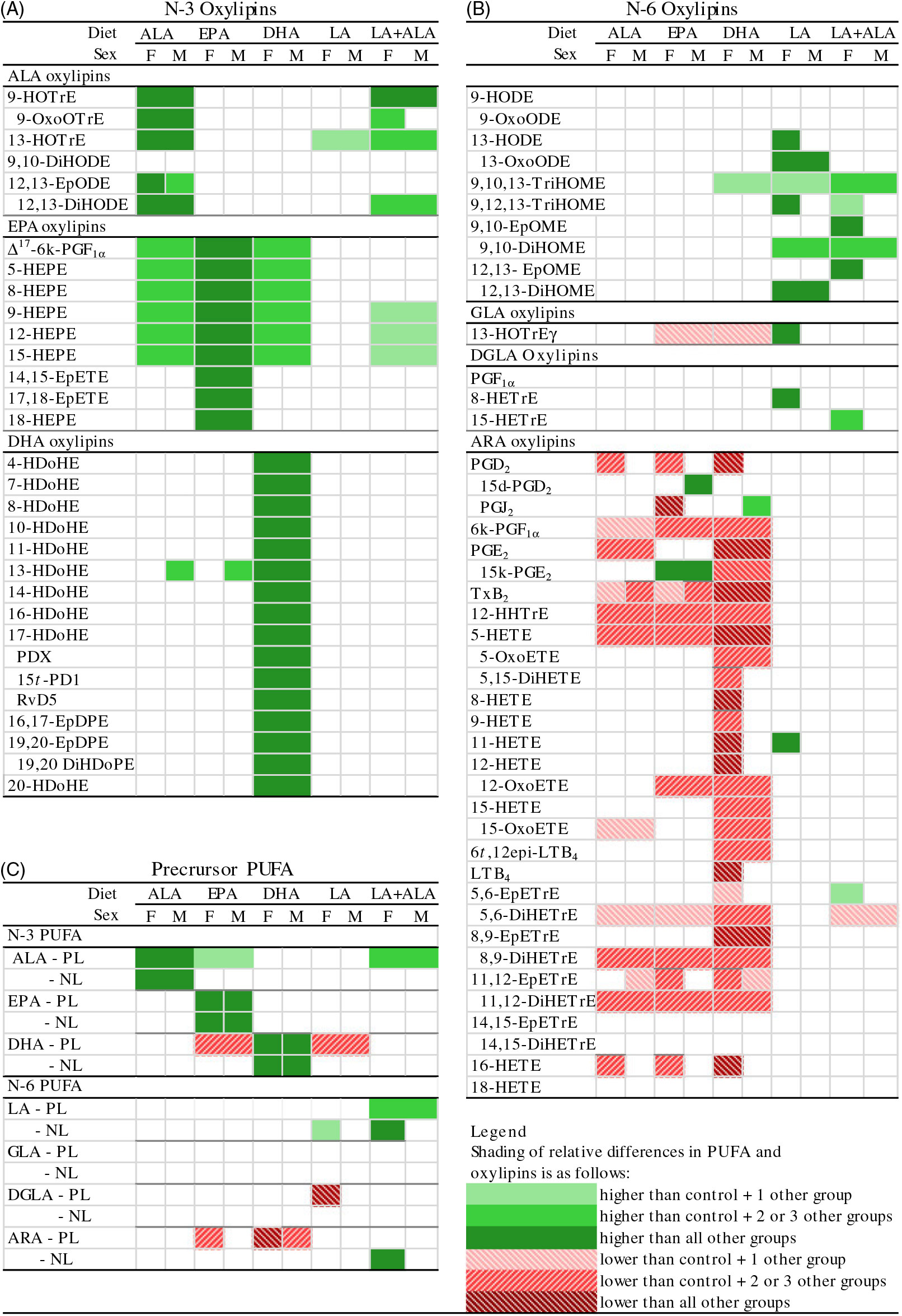
Fig. 2. Relative differences in heart (A) n-3 oxylipins, (B) n-6 oxylipins and (C) their precursor PUFA in rats provided ALA, EPA, DHA, linoleic acid (LA) and LA + α-linolenic acid (ALA) diets compared with control diets. Data were analysed by two-way ANOVA when normally distributed and by Kruskal–Wallis when not, followed by Tukey’s post hoc test. Cells are only shaded when there is a significant statistical difference (P < 0·05). Means, standard errors, P values and complete statistical analysis of diet, sex and interaction effects are provided in online Supplementary Tables S2, S3, S6 and S7. ARA, arachidonic acid; d, deoxy; DGLA, dihomo-γ-linolenic acid; DiHDoPE, dihydroxy-docosapentaenoic acid; DiHETE, dihydroxy-eicosatetraenoic acid; DiHETrE, dihydroxy-eicosatrienoic acid; DiHODE, dihydroxy-octadecadienoic acid; DiHOME, dihydroxy-octadecenoic acid; EpDPE, epoxy-docosapentaenoic acid; EpETE, epoxy-eicosatetraenoic acid; EpETrE, epoxy-eicosatrienoic acid; EpODE, epoxy-octadecadienoic acid; EpOME, epoxy-octadecenoic acid; GLA, γ-linoleic acid; HDoHE, hydroxy-DHA; HEPE, hydroxy-eicosapentaenoic acid; HETE, hydroxy-eicosatetraenoic acid; HETrE, hydroxy-eicosatrienoic acid; HHTrE, hydroxy-heptadecatrienoic acid; HODE, hydroxy-octadecadienoic acid; HOTrE, hydroxy-octadecatrienoic acid; k, keto; LT, leukotriene; NL, neutral lipid; oxoETE, oxo-eicosatetraenoic acid; oxoODE, oxo-octadecadienoic acid; oxoOTrE, oxo-octadecatrienoic acid; PD, protectin; PL, phospholipid; Rv, resolvin; t, trans; TriHOME, trihydroxy-octadecenoic acid; Tx, thromboxane.
To compare the levels of oxylipins with their PUFA precursor, the fatty acid compositions of the PL and NL fractions were analysed (Fig. 2(C); online Supplementary Tables S6 and S7). Similar to oxylipin effects, n-3 PUFA in heart PL were increased in rats provided their specific precursor PUFA (Fig. 2(C); online Supplementary Table S6). However, effects on other n-3 PUFA did not necessarily reflect oxylipin changes. For example, dietary EPA significantly reduced DHA in the PL fraction, even though all DHA oxylipins (except 13-hydroxy-DHA) were not different in rats given this diet. The results of the NL PUFA analyses were similar to the PL results – that is, n-3 PUFA in heart NL also were generally higher in rats provided their specific precursor PUFA, but other n-3 PUFA were not significantly altered, even if their oxylipins were higher (Fig. 2(C); online Supplementary Table S7).
Effects of dietary PUFA on n-6-derived oxylipins
Compared with the control group, the LA diet increased six out of ten LA-derived oxylipins, with the remaining LA oxylipins following the same trend (Fig. 2(B); online Supplementary Table S3). The LA diet also increased the one oxylipin derived from γ-linolenic acid, but very few other n-6 oxylipins were altered by dietary LA; only one of three dihomo-γ-linolenic acid (DGLA) and one of thirty ARA oxylipins were higher in female hearts. The LA + ALA diet had a similar level of LA as the LA diet, but also had a higher level of ALA compared with the control diet to achieve the same LA:ALA ratio as the control diet. n-6 Oxylipin levels in rats given this diet were similar to those given the LA diet, although there were fewer differences between the LA + ALA and the control diet than was the case for the LA compared with control diet.
Higher levels of dietary n-3 PUFA, however, resulted in lower levels of ARA-derived oxylipins, with little effect on oxylipins derived from other n-6 PUFA (LA, DGLA) (Fig. 2(B); Supplementary Table S3). The effect of individual dietary n-3 PUFA on ARA oxylipins differed, with DHA having the greatest effect: twenty-five out of thirty ARA oxylipins quantified were lower in hearts from DHA compared with control rats, while only nine and ten were lower in hearts from rats given the ALA and EPA diets, respectively.
As was the case with the n-3 PUFA, the changes in n-6 PUFA in the PL and NL sometimes reflected n-6 oxylipin changes (e.g. ARA in the PL fraction in hearts from rats given EPA and DHA diets), but other times did not (e.g. ARA in the PL and NL fractions in hearts from rats given ALA diets followed the same lowering trend but were not significantly different) (Fig. 2(A) and (C)). Another example of how oxylipin levels can differ from their precursor PUFA levels is illustrated by the higher levels of some ARA-derived PG metabolites (i.e. 15-deoxy-PGD2 and 15-keto-PGE2) in hearts from rats given the EPA diet, while twelve other ARA oxylipins were lower (Fig. 2(B)).
Sex effects in oxylipin and PUFA levels
Previous studies in these rats showed that males generally had higher levels of oxylipins in kidney and liver, except for the DHA-derived oxylipins in kidney, which were generally higher in females(Reference Leng, Winter and Aukema23). Hence sex differences in oxylipins were examined in the heart. These analyses revealed that all twenty-seven oxylipins with a main sex effect were higher in females, and that twenty-one of the twenty-two with a sex by diet interaction had higher oxylipins in females in at least one group. Only thromboxane B2 was higher in males given the LA + ALA diet (online Supplementary Table S3). However, sex differences were often not consistent across all groups, even within significant main effects. To further examine these interaction effects, the female oxylipin:male oxylipin mass ratio was calculated for each oxylipin, and the effect of diet on this ratio was tested. This analysis showed an interaction between diet and oxylipins derived from different PUFA, so the effect of diet on the female:male ratio was examined separately for oxylipins grouped by their PUFA precursor. These analyses confirmed the generally higher levels of oxylipins in females (i.e. female:male ratios were predominantly greater than 1) and further demonstrated that the female:male ratio differed with different diets. Dietary ALA resulted in the highest female:male ratio (>2) for all oxylipin groups, and dietary DHA was always among the lowest (0·5–1·5), with the remaining groups being in between (Fig. 3).

Fig. 3. Effect of diet on sex differences in oxylipin levels. Values are means, with their standard errors represented by vertical bars. Female oxylipin:male oxylipin mass ratios were analysed by one-way ANOVA when normally distributed and by Kruskal–Wallis when not, followed by Tukey’s post hoc test. a,b,c,d Ratios with unlike letters are significantly different from each other (P < 0·05). n 10 for linoleic acid ((LA), 30 for arachidonic acid (ARA), 6 for α-linolenic acid (ALA), 9 for EPA and 16 for DHA oxylipins.
Sex effects on PUFA, however, were not consistent with sex effects on oxylipins. Main effects of sex were observed in three PUFA that were oxylipin precursors in the PL fraction: LA and ALA were higher in males, and DHA was higher in females. DGLA in the PL fraction also was higher in males, but only in rats given the control and ALA diets. In the NL fraction, the only oxylipin precursor with a sex effect was EPA, which was higher in females, but only in rats given the EPA diet. Details are provided in online Supplementary Tables S6 and S7.
Oxylipin:PUFA ratios
Oxylipin:PUFA ratios were calculated to compare the relative levels of oxylipins with their precursor PUFA in the PL fraction. In almost all cases, these ratios were higher in females compared with males, reflecting the higher oxylipin levels in females. With few exceptions, oxylipin:PUFA ratios were higher for n-3 compared with n-6 PUFA, and the order of oxylipin:PUFA ratios by chain length was 18-carbon ≥20-carbon ≥22-carbon PUFA. A representative figure of these data is shown in Fig. 4 and data for all ratios can be found in online Supplementary Table S8.

Fig. 4. Heart oxylipin:PUFA ratios for the 15-lipoxygenase enzyme in rats (, female;
, male) provided the linoleic acid (LA) diet. Values are means, with their standard errors represented by vertical bars. Data were analysed by two-way ANOVA when normally distributed and by Kruskal–Wallis when not, followed by Tukey’s post hoc test. a,b,c,d Ratios with unlike letters are significantly different from each other (P < 0·05). All ratios for the 5-, 12-, 15-lipoxygenase, cytochrome P450 hydroxylase and epoxygenase enzymes for all diets are provided in online Supplementary Table S8. HODE, hydroxy-octadecadienoic acid; HETrE, hydroxy-eicosatrienoic acid; DGLA, dihomo-γ-linolenic acid; HETE, hydroxy-eicosatetraenoic acid; ARA, arachidonic acid; HDoHE, hydroxy-DHA; HOTrE, hydroxy-octadecatrienoic acid; ALA, α-linolenic acid; HEPE, hydroxy-eicosapentaenoic acid.
Discussion
The data reported herein provide fundamental data on the rat heart oxylipin profile and demonstrate how it is affected by sex and by dietary oils enriched in ALA, EPA, DHA and LA. Compared with kidney and liver in these rats, the distribution of n-6 and n-3 PUFA-derived oxylipins was generally similar, with the exception of eleven detectable epoxygenated fatty acids (i.e. epoxy-octadecenoic acid, epoxy-octadecadienoic acid, EpETrE, epoxy-eicosatetraenoic acid, EpDPE) in the heart, compared with one in the kidney and three in the liver in these same rats(Reference Leng, Winter and Aukema23). These cytochrome-P450-derived oxylipins also have been reported in a study of mouse heart oxylipins(Reference Willenberg, Rund and Rong15). Cytochrome P450 epoxygenase enzymes are highly expressed in heart(Reference Wu, Chen and Murphy32, Reference Delozier, Kissling and Coulter33), and their epoxygenated products appear to be cardioprotective, especially in post-ischaemic states, with roles such as vasodilation via activation of Ca2+-sensitive K+ channels, mitochondrial protection of cardiomyocytes, anti-apoptotic and pro-survival effects, and decreased cardiac fibrosis and inflammation effects(Reference Zhao, Wang and Xu34–Reference Samokhvalov, Alsaleh and El-Sikhry36).
Altering the dietary oil content markedly changed the heart oxylipin profiles, as would be expected from the effects on their fatty acid precursors, and from what has been observed in other tissues in these rats(Reference Mendonca, Cayer and Pauls21, Reference Leng, Winter and Aukema23, Reference Leng, Winter and Aukema24). The effects of individual n-3 PUFA could be evaluated because each diet was only enriched in one specific n-3 PUFA compared with the control diet. As in the other tissues in these rats, n-3 oxylipins were higher in rats provided the diets rich in their own individual PUFA precursor fatty acid. The heart compared with other tissues, however, was much more resistant to changes in oxylipins derived from other n-3 PUFA. EPA oxylipins were higher in hearts from rats provided the EPA, as well as the ALA and DHA diets, while ALA and DHA oxylipins were only elevated when rats were provided their direct precursor PUFA. In comparison, in kidney and some adipose depots, DHA oxylipins were higher in rats given diets enriched either in ALA, EPA or DHA. Heart oxylipins also changed less in response to dietary PUFA than the liver(Reference Leng, Winter and Aukema24), in which some ALA oxylipins were higher in rats given EPA and DHA diets, and in kidneys(Reference Leng, Winter and Aukema23)and some adipose depots, in which some ALA oxylipins were lower in rats given EPA and/or DHA diets(Reference Mendonca, Cayer and Pauls21). The apparent lack of effect of ALA or EPA on DHA oxylipins in the heart is supported by findings that the rat heart lacks elongase-2(Reference Igarashi, Ma and Chang37).
These findings may indicate that EPA oxylipins are key n-3-derived oxylipins in the heart, and a number of EPA oxylipins have beneficial effects in this regard. For example, 18-HEPE can prevent cardiac remodelling in the pressure-overload-induced mouse model(Reference Endo, Sano and Isobe38) and epoxygenated EPA such as 17,18-epoxy-eicosatetraenoic acid have antiarrhythmic effects in neonatal cardiomyocytes(Reference Arnold, Markovic and Blossey39) and vasodilatory effects in coronary smooth muscle cells(Reference Ye, Zhang and Oltman11). Other EPA-derived eicosanoids such as PGD3, PGE3 and thromboxane B3 have lesser or no effects on inducing arrhythmias in cultured neonatal rat cardiac myocytes, when compared with their ARA-derived counterparts(Reference Li, Kang and Leaf40), and EPA-derived resolvin E1 can limit infarct size and ischemia-reperfusion injury in male rats(Reference Keyes, Ye and Lin41). Nevertheless, DHA oxylipins such as 13,14-EpDPE, 13,14-dihydroxy-DHA and 17,18-EpDPE have vasodilatory effects in coronary smooth muscle cells(Reference Ye, Zhang and Oltman11, Reference Li, Hong and Li42) and 19,20-EpDPE has antiarrhythmic effects in neonatal cardiomyocytes(Reference Westphal, Konkel and Schunck43), indicating that these also have potential roles in the heart. To our knowledge, no effects of ALA oxylipins on heart function have been reported.
In addition to these effects on n-3 oxylipins, dietary n-3 PUFA may also protect the heart by reducing the levels of n-6 oxylipins, particularly those derived from ARA. DHA was more potent in this regard, compared with both EPA and ALA, suggesting that DHA may have been more responsible for the reduction in the ARA oxylipins that has previously been observed in rat myocardium, as well as other tissues, with fish oil feeding(Reference Abeywardena and Charnock22, Reference Devassy, Yamaguchi and Monirujjaman44, Reference Ibrahim, Jia and Devassy45). ARA oxylipins are associated with several functional effects in the heart. For example, increased PGF2α and PGE2 are associated with hypertrophic growth and production of arrhythmias in neonatal cardiac myocytes(Reference Li, Kang and Leaf40, Reference Adams, Migita and Yu46, Reference Mendez and LaPointe47), the lipoxygenase-generated HETE are increased in mitochondria from failing human hearts(Reference Moon, Liu and Cedars48) and 20-HETE induces vasoconstriction in small porcine coronary arteries(Reference Randriamboavonjy, Busse and Fleming49). Some effects of ARA oxylipins are protective, however, such as the vasodilatory EpETrE, which may prevent obesity-induced cardiomyopathy and reduce post-ischaemic ventricular polarisation in an isolated heart model(Reference Cao, Singh and McClung50, Reference Batchu, Law and Brocks51), and PGI2, which displays protective effects against the arrhythmias induced by other prostanoids in rat cardiac myocytes(Reference Li, Kang and Leaf40). However, ARA oxylipins are more often associated with detrimental effects, while the EPA and DHA oxylipins are associated with cardio protective effects, as described above. Thus, the combination of higher levels of EPA (and DHA, and possibly ALA) oxylipins and lower levels of ARA oxylipins with ALA, EPA and DHA feeding would be predicted to have an overall protective effect on rat heart functions.
Increased dietary LA also has been promoted as heart healthy due to improvements in the blood lipoprotein profile, but the benefits of LA diets on CVD remain controversial(Reference Harris, Mozaffarian and Rimm52–Reference Ramsden, Zamora and Majchrzak-Hong54). Dietary LA increases renal and hepatic n-6 oxylipins derived from n-6 PUFA, including ARA(Reference Caligiuri, Love and Winter19, Reference Leng, Winter and Aukema23), suggesting that there may be effects on oxylipins that are not evident based on tissue fatty acid composition alone. In contrast to these tissues, dietary LA effects in the heart are restricted to increases in LA, γ-linolenic acid and DGLA oxylipins, further indicating greater resistance to changes in oxylipin composition by dietary oils in the heart compared with other tissues. Whether these oxylipins have significant effects on heart health, however, remains to be elucidated. On the one hand, the LA oxylipin 9,10-epoxy-octadecenoic acid can induce heart failure in dogs(Reference Sugiyama, Hayakawa and Nagai55), and its metabolite, 9,10-dihydroxy-octadecenoic acid, has been reported to decrease left ventricular-developed pressure recovery in the ischaemic/reperfused mouse heart(Reference Edin, Wang and Bradbury56). On the other hand, the LA oxylipin, 13-hydroxy-octadecadienoic acid, and several DGLA oxylipins have been demonstrated to have anti-platelet effects(Reference Whitaker, Wyche and Fitzpatrick57–Reference Ikei, Yeung and Apopa59).
Interestingly, there also were several exceptions to these generalised patterns. For example, ARA oxylipins such as 15-deoxy-PGD2 in EPA male, PGJ2 in DHA male and 15-keto-PGE2 in (both male and female) EPA rats were increased while most of the other ARA oxylipins were decreased. Exceptions similar to this were observed in the kidney, liver and serum of these rats(Reference Leng, Winter and Aukema23), as well as in human plasma after dietary intervention with individual EPA or docosapentaenoic acid (22 : 5n-3)(Reference Markworth, Kaur and Miller60). These PG metabolites are formed by hydroxy PG dehydrogenase (PGDH) activity(Reference Anggard, Larsson and Samuelsson61) and often have been considered to be inactive metabolites of their parent compounds. Although their functions are not well characterised, several studies report physiological effects. For example, 15-keto-PGE2 can inhibit inflammatory cytokine production in acute liver injury(Reference Yao, Chen and Song62) and 15-PGDH activity suppresses colon tumorigenesis in vivo (Reference Myung, Rerko and Yan63). How dietary n-3 PUFA affects PGDH activity or whether its oxylipins play functional roles in the heart by increasing these PG metabolites remains to be elucidated.
These data also illustrated other differences between fatty acid and oxylipin levels. For example, oxylipin product:PUFA precursor ratios are higher for shorter compared with longer chain PUFA and for n-3 compared with n-6 PUFA. This is similar to findings in other rat tissues(Reference Caligiuri, Love and Winter19, Reference Leng, Winter and Aukema23, Reference Leng, Winter and Aukema24), and in studies of ARA compared with LA, and with EPA and DHA metabolism by 15-lipoxygenase(Reference Soler, Saura and Garcia-Lopez64, Reference Kutzner, Goloshchapova and Heydeck65), and ARA compared with EPA and DHA metabolism by cytochrome P450 enzymes in vitro (Reference Arnold, Markovic and Blossey39, Reference Fischer, Konkel and Mehling66). These findings may suggest that dietary 18-carbon and n-3 PUFA may have greater effects on oxylipins in the heart. Thus, along with the greatest sex difference in oxylipins in ALA-fed rats, dietary ALA may have greater impact on heart physiology than suggested by fatty acid data alone.
The higher levels of DHA and lower levels of LA observed in female heart PL in the present study have previously been reported in the rat, although not always consistently(Reference Slater-Jefferies, Hoile and Lillycrop67, Reference Kitson, Smith and Marks68). Further we also observed higher ALA in male heart PL, which was not observed in these studies, possibly due to the inclusion of the high-ALA diet herein. However, to our knowledge, sex effects on the heart oxylipin profile have not been examined. In other tissues in these rats, we found that males generally have higher levels of oxylipins in kidney and liver(Reference Leng, Winter and Aukema23). In several adipose depots, oxylipin sex effects were observed in fewer oxylipins, with many having interaction effects. Interestingly, in those with interactions, they were higher in females given the control, ALA and EPA diets, but higher in males given the DHA diet(Reference Mendonca, Cayer and Pauls21). This pattern is similar to the present findings in the heart where oxylipins in DHA rats were similar in females and males, but oxylipins in rats given the ALA diet were higher in females. The mechanism by which dietary PUFA interact with sex effects on heart oxylipins is not known, as this is a novel finding. In one study, a high-fat diet reduced renal cortical 20-HETE and EpETrE production in male but not female rats(Reference Zhou, Lin and Chang69), indicating that dietary fat may exert differential sex effects. However, the mechanisms by which sex alone, or in combination with diet, affects oxylipin levels remain largely unexplored in any tissue. There are examples of oestrogen increasing individual oxylipins and/or the enzymes that produce them(Reference Jun, Chen and Pace25, Reference Calkin, Sudhir and Honisett26, Reference Geary, Krause and Duckles70) or testosterone reducing these(Reference Pergola, Rogge and Dodt71). However, there are also studies which demonstrate higher levels of oxylipins in males or ovariectomised females(Reference Leng, Winter and Aukema23, Reference Zuniga-Munoz, Guarner Lans and Soria-Castro72, Reference Holla, Adas and Imig73), so much remains to be elucidated in this regard. Interestingly, sex differences in oxylipins are exhibited to the greatest extent in oxylipins derived from DHA, compared with all other PUFA. This may be due to the higher levels of DHA in female heart PL, but this also remains to be investigated.
It should be noted that free oxylipins represent only a portion of the total oxylipins present in tissue. There is also a large pool of esterified oxylipins, but free oxylipins have been presumed to be the most active form and have been the most frequently studied. However, several reports also describe functions for esterified oxylipins(Reference O’Donnell and Murphy74, Reference Slatter, Percy and Allen-Redpath75), and the presence of these in the heart would have an impact on the interpretation of the present data, although one study has reported that dietary modulation affects free and esterified oxylipins in a similar manner(Reference Schebb, Ostermann and Yang76).
Conclusion
In conclusion, this study provides novel data on the free oxylipin profile of the rat heart and on the differential effects of dietary n-3 and n-6 PUFA and sex on this profile. The dietary PUFA have the greatest effect on their own oxylipins, more so in the heart than other tissues. In addition, ARA oxylipins are markedly reduced by dietary n-3 PUFA with the effect being much greater with DHA compared with EPA and ALA. LA oxylipins, however, were largely unaffected by dietary n-3 PUFA, but were increased with higher dietary LA intake, even in the absence of increased LA in heart PL. Other discrepancies between oxylipin and fatty acid levels were observed for several PGDH-derived ARA oxylipin metabolites in EPA rats, for differences in oxylipin to parent PUFA ratios and for sex differences, further demonstrating that oxylipins do not necessarily reflect fatty acid compositions. Notably, these data show that sex differences in heart oxylipins interact with dietary PUFA effects, with dietary ALA compared with DHA resulting in higher levels of oxylipins in females. These fundamental data on heart oxylipins with unique dietary PUFA and sex effects will help guide further investigations on the functions of oxylipins in the heart.
Acknowledgements
The authors thank Dennis Labossiere and Dennis Joseph for technical support and the St. Boniface Hospital Foundation for infrastructure support.
This study has been supported by the Natural Sciences and Engineering Research Council of Canada, the University of Manitoba Graduate Enhancement of Tri-Council Stipends (GETS) programme, Pizzey Ingredients and Manitoba Agriculture, Food and Rural Initiatives. Harold M. Aukema received grant support from Pizzey Ingredients.
A. F., S. L., H. M. A. designed the research; A. F., S. L., and T. W. conducted the research; A. F. and H. M. A. analysed the data, wrote the manuscript and had primary responsibility for final content.
The authors declare that there are no conflicts of interest.
Supplementary material
To view supplementary material for this article, please visit https://doi.org/10.1017/S0007114519001211