A high intake of vegetables is associated with a reduced risk of numerous cancers, including colorectal cancer (CRC). The mechanisms by which vegetables exert their protective effects are diverse and generally involve changes in gene expression(Reference Steinmetz and Potter1).
Control of gene expression is largely determined by chromatin structure. The accessibility of the chromatin is determined by the patterns of post-translational modifications of histones and by the methylation state of CpG dinucleotides in the promoter region of genes. Methylation of CpG dinucleotides is the main epigenetic modification of DNA(Reference Strachan, Read, Strachan and Read2).
There is considerable evidence that aberrant DNA methylation plays an integral role in oncogenesis(Reference Feinberg, Ohlsson and Henikoff3). Transcriptional silencing of genes involved in the regulation of cell proliferation, apoptosis and DNA repair by epigenetic mechanisms is critical in the pathogenesis of cancer, including CRC(Reference Jubb, Bell and Quirke4), and may be an early event predisposing to neoplasia(Reference Feinberg, Ohlsson and Henikoff3).
The pattern of methylation is potentially reversible and offers an excellent target for modification of CRC risk by environmental factors, including diet(Reference Cameron, Bachman and Myohanen5). However, information about the effects of dietary factors on gene-specific changes in methylation, especially in human subjects, is limited.
In a previous study, we examined the effect of altering vegetable intake on gene expression changes in the colon of female sporadic adenoma patients and healthy controls(Reference van Breda, van Agen and Engels6). The present study was initiated to test the hypothesis that changes in expression of genes involved in CRC in response to altered vegetable intake are associated with changes in methylation of the promoter regions of these genes, thereby contributing to understanding of the mechanism(s) of action responsible for the chemopreventive properties of vegetables. Therefore, we have investigated the methylation status of specific CpG within the CpG islands of the promoter region from a subset of these genes, by means of the modified combined bisulfite restriction analysis assay. Four genes were analysed: protein kinase C β 1 (PKCB1), ornithine decarboxylase 1 (ODC1), fos proto-oncogene (C-FOS) and 5,10-methylenetetrahydrofolate reductase (MTHFR). Within the group of differentially expressed genes, these four genes were selected for methylation analysis because only these genes met the following criteria: functionally relevant for CRC; gene expression significantly modulated; the presence of CpG islands in the promoter region of the gene and the availability of a methylation-specific restriction enzyme to permit quantitative assay.
Materials and methods
Subjects
The present study on DNA methylation is part of a project investigating the effects of increasing or decreasing the vegetable intake on gene expression in the colon mucosa of female adenoma patients and healthy controls. The characteristics of the study population and the study design have been published previously(Reference van Breda, van Agen and Engels6). In short, the participants were recruited at the Maasland Hospital Sittard (The Netherlands). Eligible patients had a history of colorectal adenomas but no familial CRC syndrome or inflammatory bowel disease; eligible controls had no history of colorectal adenomas or other colorectal disorders. Both patients and controls were allocated at random into two groups of ten and four individuals respectively, receiving either a low (75 g/d) or high (300 g/d) vegetable diet (consisting of cauliflower, carrots, peas and onions) for a period of 2 weeks. Four rectal biopsies were collected at the first endoscopic examination and on the day following the last day of the intervention period. The present study was approved by the Medical Ethical Committee of the Maasland Hospital (Sittard, The Netherlands) and written informed consent was obtained from the participants before the start of the study.
DNA isolation
Four rectal biopsies (about 20 mg in total) from each subject were pooled and ground to a powder in a stainless steel mortar under liquid N2 and homogenised in 800 μl TRIzol Reagent (GIBCO BRL, Life Technologies, Carlsbad, CA, USA). After isolation of RNA, which was used for gene expression analyses(Reference van Breda, van Agen and Engels6), DNA was extracted from the residuals according to the manufacturer's instructions. However, additional purification of the DNA appeared necessary. Therefore, DNA was treated overnight at 55°C with Proteinase K (Roche Diagnostics, Basel, Switzerland) (0·5 mg/ml in 2 mm-2-amino-2-hydroxymethyl-propane-1,3-diol-HCl, pH 7·4), followed by treatment with Rnase A (Roche Diagnostics) (100 μg/sample) and Rnase T1 (Roche Diagnostics) (50 units/sample) for 30 min at 37°C. Next, DNA was purified and concentrated using Dneasy® columns (Qiagen, Valencia, CA, USA). Quantity and quality of each DNA sample were assessed spectrophotometrically. The amount of DNA per sample varied from 25 to 68 μg.
Analysis of CpG island methylation: modified combined bisulfite restriction analysis assay
A modified combined bisulfite restriction analysis assay(Reference Belshaw, Elliott and Williams7) was used to determine the methylation status of CpG within the CpG islands in the promoter region of the four genes C-FOS (at +40 from the start of transcription), PKCB1 (at +166), ODC1 (at +82) and MTHFR (at +384).
Bisulfite modification
Genomic DNA (2 μg) was treated with sodium bisulphite according to the method of Raizis et al. (Reference Raizis, Schmitt and Jost8).
Polymerase chain reaction amplification
By means of PCR, components of the CpG islands within the promoter regions of the four genes were amplified. An approximately 600 bp long DNA sequence upstream of the transcriptional start area of each gene was selected as the promoter region using Ensemble (http://www.Ensemble.org/). The presence of CpG islands within these promoter sequences was predicted using the online software program Methprimer (http://www.urogene.org/methprimer/). PCR reactions (25 μl), containing 12·5 μl 2 × HotStarTaq Master Mix (Qiagen), 4 pmol of each forward and reverse primer (MWG Biotech, Milton Keynes, Bucks, UK), 1 μl bisulfite modified DNA and supplemented with MgCl2 to a final concentration of 2 mm, were subjected to the following cycling conditions: one cycle at 95°C for 15 min, thirty-five cycles at 95°C for 30 s, annealing temperature for 1 min (50°C for PKCB1, C-FOS and MTHFR; 57°C for ODC1), 72°C for 1 min, followed by a 5 min extension at 72°C. PCR products were stored at 4°C until restriction analyses.
Restriction analyses
PCR mixtures were digested overnight with 5 units of the restriction enzyme (NruI for PKCB1; TaqI for ODC1, and AclI for C-FOS and MTHFR) (New England Biolabs, Ipswich, MA, USA), according to the conditions specified by the manufacturer. Restriction enzymes at a methylation-sensitive CpG site were selected using the online program webcutter (http://rna.lundberg.gu.se/cutter2/). Digested PCR products were separated on gel and stained with SYBR green I (Molecular Probes, Invitrogen, Eugene, OR, USA). The DNA SYBR green fluorescence was captured using a CCD camera fitted with UVIphoto software and band intensities were quantified using UVIband (Uvitec Limited, Cambridge, Cambs, UK). The proportions of methylated and unmethylated DNA were calculated from the relative intensities of cut and uncut PCR product (total intensity set at 100 %). Next, a methylation difference for each subject and gene was calculated by subtracting the proportion of methylated alleles before the intervention from the proportion of methylated DNA after the intervention.
Statistical analysis
Statistical analysis of the data was carried out using SPSS (version 12.0 for Windows; SPSS Inc., Chicago, IL, USA). Potential differences in methylation status were assessed using ANOVA for patients v. controls, for increased (high) v. decreased (low) vegetable intake, and for patients and controls within each vegetable group. All tests were two-sided and adjusted for differences in age. A P value < 0·05 was considered to indicate statistical significance.
Results
Methprimer predicted CpG islands within the promoter region of all four genes. PCR amplification of a region of these CpG islands was successful (data not shown). Next, PCR products were digested using a restriction enzyme that cuts at a predicted methylation site, and separated on a gel (data not shown). The extent of methylation at the chosen CpG site in the promoter region of the four genes for both patients and controls before and after the dietary intervention is shown in Table 1. This Table also shows the fold changes of the expression of these four genes after the vegetable intervention and the difference in promoter methylation (after – before) which facilitates direct comparison between changes in DNA methylation and in gene expression by an altering intake of vegetables.
Table 1 Effect on gene expression and on DNA methylation extent (%) at a single CpG site in the promoter region of four genes significantly modulated by vegetables
(Mean values with their standard errors)
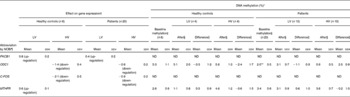
LV, low vegetable group (75 g/d); HV, high vegetable group (300 g/d); NCBI, National Center for Biotechnology Information; PKCB1, protein kinase C β 1; ND, not detectable; ODC1, ornithine decarboxylase 1; C-FOS, fos proto-oncogene; MTHFR, 5,10-methylenetetrahydrofolate reductase.
* Extent (%) of methylation at a single CpG site in the promoter region of four genes whose expression was significantly modulated by vegetable intake.
† Gene expression changes (mean log(2)-fold changes) in colon mucosa after an intervention with vegetables during 2 weeks(Reference van Breda, van Agen and Engels6). Empty cells indicate that there was no significant change in expression.
‡ Promoter methylation (%) before the intervention.
§ Promoter methylation (%) after the intervention.
∥ DNA methylation difference. This was calculated by subtracting the promoter methylation (%) before the intervention from promoter methylation (%) after the intervention.
** Mean value was significantly lower than that in healthy controls (P < 0·01).
For both PKCB1 and CFOS, the extent of methylation in all samples was too low to quantify. Although the extent of methylation was quantifiable for ODC1 and MTHFR, in more than 90 % of the samples the level of methylation was less than 7 %. No significant differences in the extent of methylation in colon DNA were detected for any of the four genes in either patients or controls after the vegetable diet intervention (data were adjusted for age). However, at baseline, the extent of methylation in the promoter region of the ODC1 gene was significantly higher in healthy controls (5·3 (sem 1·1) %) compared with that in adenoma patients (2·0 (sem 0·5) %) (P < 0·01).
Discussion
Little is known about the mechanisms responsible for the regulation of expression of any of the four genes PKCB1, C-FOS, ODC1 and MTHFR, or how the expression can be affected by exogenous factors such as diet. However, the coupling of promoter methylation to gene expression silencing is well established(Reference Klose and Bird9) and other regulatory mechanisms have been reviewed by Mathers(Reference Mathers, Choi and Simonetta10). Furthermore, although biopsies were taken from phenotypically normal tissue, gene expression differences could be detected, indicating that changes in CpG island methylation may be a possible mechanistic explanation.
In the present study, methylation in the promoter region of PKCB1 and C-FOS was below the limit of detection. Consequently the change in expression of these genes by the altering vegetable intake cannot be explained by a change in DNA methylation. No other studies have investigated the effect of diet on either methylation or mRNA expression of PKCB1. Two previous studies have investigated DNA methylation changes in relation to C-FOS gene expression change(Reference Christman, Sheikhnejad and Dizik11, Reference Wainfan and Poirier12). However, this research was carried in rat liver and no comparable human data are available.
ODC1 catalyses the first step in the polyamine biosynthetic pathway, a highly regulated pathway associated with rapid growth states, including higher CRC risk(Reference Martinez, O'Brien and Fultz13). Although low levels of ODC1 promoter methylation could be quantified in the colonic mucosa of both healthy control subjects and adenoma polyp patients, there was no evidence that down-regulation of expression by vegetable intake, as reported in our previous study(Reference van Breda, van Agen and Engels6), coincided with a significant change in methylation. Several studies have investigated the methylation status of the promoter region of the ODC1 gene(Reference Alhonen-Hongisto, Leinonen and Sinervirta14–Reference Wahlfors16) and found that the promoter region was extensively methylated(Reference Alhonen-Hongisto, Leinonen and Sinervirta14–Reference Wahlfors16). Furthermore, hypomethylation within and/or around the ODC1 gene was associated with an enhanced accumulation of ODC1 mRNA(Reference Alhonen-Hongisto, Leinonen and Sinervirta14, Reference Halmekyto, Hirvonen and Wahlfors15). It must, however, be noted that most of these studies investigated the methylation status of the ODC1 gene in tumour cells, and so do not provide information relevant to healthy normal tissue. To our knowledge, only one previous study has examined ODC1 methylation status in humans(Reference Alhonen-Hongisto, Leinonen and Sinervirta14). ODC1 was less methylated in leukaemia cells than in leucocytes from healthy subjects. We now report that the methylation of the ODC1 gene at target tissue level, i.e. in the colonic mucosa of healthy subjects, was higher than that in the adenoma patients who are at higher risk of CRC. This difference would be expected to contribute to reduced ODC1 expression, ODC1 activity and polyamine concentrations in healthy mucosa.
Although methylation of the promoter region of the MTHFR gene was quantifiable in mucosa from both healthy controls and adenoma polyp patients, the levels of methylation were low (and similar) in both subject groups. The up-regulation of MTHFR gene expression in the colon of healthy controls cannot be explained by a change in methylation status of the promoter region of this gene. We are not aware of other published studies that have investigated the methylation status of the promoter region of MTHFR in response to dietary intervention.
There are several possible explanations for the absence of methylation changes in the promoter region of the investigated genes in response to the dietary intervention. First, most of the previous dietary studies that have found an effect on DNA methylation have modified methyl group supply specifically. Most of these studies have been undertaken in rodents in which dietary methyl (folate, choline and methionine) deficiency causes global or specific DNA hypomethylation(Reference Lawrance, Fiocchi and Chakravarti17–Reference Feil19). The results of more recent studies of manipulation of folate supply in humans and implications for CRC have been reviewed by Mathers(Reference Mathers20). It has been suggested that dietary interventions which do not involve major changes in methyl group supply are unlikely to have a major effect on DNA methylation(Reference Fang, Chen and Yang21), but this has yet to be evaluated. Second, the time-scale for the establishment of DNA methylation changes might be longer. The third possibility is that the gene expression changes that we have observed are probably not the result of changes in DNA methylation. Other epigenetic markers such as changes in histone acetylation patterns could have contributed to the observed effect on gene expression, as could changes in the supply of transcription factors due to the dietary intervention(Reference Mathers, Choi and Simonetta10).
This is the first study in which the methylation status of genes in response to dietary intervention has been investigated in the colonic mucosa of human subjects. We have shown the presence of low levels of methylation of the promoter region of the ODC1 and MTHFR gene, in both healthy control subjects and adenoma polyp patients. Interestingly, the extent of ODC1 promoter methylation was lower in the colonic mucosa of the adenoma polyp patients when compared with the healthy control subjects, which could contribute to the increased ODC1 activity in CRC. However, we found no evidence that halving (i.e. 75 g/d) or doubling (i.e. 300 g/d) the consumption of vegetables for 2 weeks had a detectable effect on the extent of methylation of the chosen genes in humans.
Acknowledgements
These DNA methylation studies were supported through an award from the European Nutrigenomics Organization (NuGO; CT-2004-505944) a Network of Excellence funded by the European Commission's Research Directorate General under Priority Thematic Area 5, Food Quality and Safety Priority, of the Sixth Framework Programme for Research and Technological Development.
All authors of this research paper have directly participated in the planning, execution or analysis of the study. S. G. J. vB. was involved in the setting up and executing of the human dietary intervention study, performed all the analyses and wrote the manuscript; J. H. M. vD. and J. C. S. K. were involved in the planning and setting up of the study and supervised the human intervention and gene expression studies. L. G. J. B. E. was the gastroenterologist who was involved in patient recruitment and examination of all endoscopic procedures. J. C. M. supervised the methylation analyses and was head of the department where all methylation analyses were performed. None of the authors have any conflicts of interest.