INTRODUCTION
The Intermediate Bronze Age culture (IBA hereafter, but also known as EB IV) occupied the southern Levant in the second half of the third millennium BCE. The IBA is accepted by scholars as a non-urban pastoral-rural culture, with clear differentiation in settlement patterns, material culture, and burial habits, from the urban Early Bronze culture (EB hereafter) preceding it, and from the urban Middle Bronze culture (MB hereafter) following it. An abundance of burial sites, each including up to a few hundred burials, characterize the IBA (e.g., Oren Reference Oren1973; Dever Reference Dever1975; Yannai Reference Yannai2016). IBA settlements are mainly unfortified villages established on a new unexploited land (e.g., Smithline Reference Smithline2002; Eisenberg Reference Eisenberg2012; Dever Reference Dever2014; Covello-Paran Reference Covello-Paran2020). Exceptions are cases of IBA remains which were built on EB settlement ruins (e.g., Mazar Reference Mazar2006), a few walled settlements (e.g., Bar et al. Reference Bar, Cohen and Zertal2013), and also a few IBA sites in Transjordan utilizing the EB III city architecture as their base, thus displaying local continuity (e.g., Richard Reference Richard2010). Our knowledge of the IBA has been much enhanced during the last decade (D’Andrea Reference D’Andrea2014; Dever Reference Dever2014; Falconer and Fall Reference Falconer and Fall2019; Richard Reference Richard2020). Yet, the roots of this culture and its interaction with the preceding EB III culture are still not fully understood.
The recent EB “High Chronology,” which is based on a systematic analysis of 14C dates obtained from EB sites in the Southern Levant, showed that EB III cities ceased to exist around 2500 BCE. Such observation extends the duration of the IBA period from about 300 years, as was the previous consensus, to about 500–600 years (Regev et al. Reference Regev, de Miroschedji, Greenberg, Braun, Greenhut and Boaretto2012, Reference Regev, Finkelstein, Adams and Boaretto2014; Höflmayer et al. Reference Höflmayer, Dee, Genz and Riehl2014), shifting more attention to the transition from the EB III to the IBA. Yet another current view is that the end of the EB III did not happen simultaneously everywhere and that there are cases, as in Jericho, where EB III ended later, around 2300 BCE (Nigro et al. Reference Nigro, Calcagnile, Yasin, Gallo and Quarta2019). Early IBA absolute radiocarbon (14C) dates from around 2500 BCE or even somewhat earlier have been published lately from short-lived samples from Tell Abu el-Niaj in Transjordan (Falconer and Fall Reference Falconer and Fall2019; Fall et al. Reference Fall, Falconer and Höflmayer2020) and Khirbat el-'Alya in the Judean Shefelah region (Lev et al. Reference Lev, Shalev, Paz, Regev and Boaretto2020). Absolute dates between 2500 and 2300 have also been published lately from IBA contexts in the Negev (Dunseth et al. Reference Dunseth, Junge and Lomax2017). As stated above, most IBA settlements excavated to date in the southern Levant were established as new rural sites. Tel Hazor in the north of modern-day Israel (Figure 1) is one of the few sites where IBA architectural remains were found above the EB III city ruins (Bechar Reference Bechar2017a). Other such sites west of the Jordan River are Beth-Shean (Mazar Reference Mazar2006), Jericho (Nigro Reference Nigro2003), and possibly Megiddo (Adams Reference Adams2017). In the later EB III stratum at Hazor (Stratum XIX) only a few sherds of the distinct pottery type Khirbet Kerak Ware, a type which is common in EB III phases of sites in northern Israel, were found. This stands in contrast to the earlier EBIII stratum (Stratum XX), where large amounts of sherds of this family were found (Ben-Tor Reference Ben-Tor2017: 149), thus suggesting the existence of a late EB III phase (EB IIIB according to the Hazor excavators) in Stratum XIX at Hazor (Ben-Tor and Bechar Reference Ben-Tor and Bechar2017: 1, 3). The research questions to be tackled here are the following: (1) what is the date of the last phase of Hazor’s EB III city? (2) What is the date of the IBA occupation above it? And (3) what is the chronological relationship between these two settlements? The excavators of Hazor proposed two scenarios in their discussion regarding the relationship between these two strata: either … only a short occupational gap (if any) between the Early and Intermediate Bronze Age strata at Hazor—or—an occupation gap of two or three centuries at Hazor. Of the two options—they favored only a short occupational gap, if any (Ben-Tor and Bechar Reference Ben-Tor and Bechar2017: 1). Our approach will complement the relative chronological considerations applied to date. This was done by locating and 14C dating organic samples from representative contexts of the relevant strata at Hazor, in order to answer our research questions.

Figure 1 Left: Location of Tel Hazor and other sites with IBA remains mentioned in the paper. Right: Partial view of Area A on the acropolis of Tel Hazor before our probe excavation, looking north (after Zuckerman and Bechar Reference Zuckerman and Bechar2017 Photo 1.6). Our probe excavation parameters are drawn on the photo.
The Kimmel Center for Archaeological Science at the Weizmann Institute of Science conducted a small probe excavation at Tel Hazor during June 2017, in collaboration with the directors of the Selz Foundation Hazor Excavations in Memory of Yigael Yadin (Hebrew University), Amnon Ben-Tor, and Shlomit Bechar. The location of this probe excavation was carefully chosen to collect samples that best represent both the last phase of the EB III city of Hazor and the IBA settlement above it. Locus L.80035 of the Hazor excavations is a representative context of the Stratum XIX, the last phase of the EB III city (Zuckerman and Bechar Reference Zuckerman and Bechar2017: 9, Plan 1.2). According to its ceramic assemblage, this locus is contemporary with the other post Khirbet Kerak ware EB III contexts identified by Yadin’s excavations at Hazor (Ben-Tor Reference Ben-Tor2017: 149). Architectural remains from the IBA at Hazor were found solely by the renewed excavations. Locus L.80006 best represents Stratum XVIII, dated to the IBA. This locus is a plaster floor with multiple IBA vessels found in situ on it (Bechar Reference Bechar2017a: 14, 2017b: figs. 6.11, 6.12). The selected location for the probe excavation was a baulk in area A (the acropolis) that held the northern continuation of both L.800035 (Stratum XIX) and L.80006 (Stratum XVIII). Although our probe excavation covered only a small area (2 × 1 m wide and 1.5 m deep, see Figures 1, 2), it was able to reach well-excavated contexts of Hazor excavations, dating to Stratum XIX and Stratum XVIII, precisely the contexts to be investigated within the framework of this study.

Figure 2 The parameters of the 2017 probe excavation marked in relation to the IBA remains, located in the southern part of the acropolis (after Bechar Reference Bechar2017a: Plan 2.2).
MATERIALS AND METHODS
Excavation Method
The excavation was performed in layers of 5–10 cm. Each such layer was defined as a locus to allow high context analysis control. Particular contexts (installations, floors, stone accumulations) were assigned another separate locus. For each locus, multiple sediment samples were collected in plastic vials of 10 mL for later context analysis. Changes in color or consistency of the sediments were also recorded, and characteristic material was collected. When a conspicuous context was encountered (e.g., burnt material, pottery in articulation, plaster material), higher sampling resolution was performed to obtain an adequate record of that context. Charred remains were carefully searched for visually within in-situ sediments during excavation. Sediments estimated to contain potential in-situ charred remains were collected and dry-sifted in the field through a 2-mm mesh sieve to locate seeds, pits, and charcoal. The identified organic remains were documented and collected in aluminum foil envelopes. No wet sieving was performed as we have verified that wet sieving recovers less material than dry sieving in the type of sediment that was excavated, as some charred remains dissolved in the water.
Radiocarbon Samples Context Characterization
A microarchaeological approach was implemented during the excavation and sampling process to provide insight into the site-formation and post-depositional processes. This methodology enabled us to verify secure contexts for dating and to choose samples for 14C dating that were burned or buried in situ. It allowed us to identify and exclude samples that may have been included in the archaeological context by post-depositional processes (e.g., bioturbation), or residual from earlier occupation levels (for examples of microarchaeological research see Boaretto Reference Boaretto2015; Dunseth et al. Reference Dunseth, Junge, Fuchs, Finkelstein and Shahack-Gross2016; Toffolo et al. Reference Toffolo, Maeir, Chadwick and Boaretto2012). The sediment samples that were collected during the excavation, and from around the excavated area as control, were analyzed to understand the sampled location context and its relation to its surroundings by using the following analytical methods:
-
FTIR (Fourier transformed infrared spectroscopy)—Nicolet iS5 FTIR was used to explore the sample mineral composition and to identify anthropogenic features such as burnt clay and phosphates (Berna et al. Reference Berna, Behar and Shahack-Gross2007; Weiner Reference Weiner2010).
-
Phytolith counts (number of phytoliths per 1 g of sediment)—were conducted to compare phytoliths abundance between the various locations and identify dominant vegetation types, using phytoliths extraction and quantification procedure (Katz et al. Reference Katz, Cabanes, Weiner, Maeir, Boaretto and Shahack-Gross2010).
-
Microscopic examination—was done to further understand the sediment context by locating special inclusions such as calcium-oxalate pseudomorphs indicating ash remains and dung spherulites indicating the presence of dung or manure (Gur-Arieh et al. Reference Gur-Arieh, Shahack-Gross, Maeir, Lehmann, Hitchcock and Boaretto2014; Dunseth et al. Reference Dunseth, Junge, Fuchs, Finkelstein and Shahack-Gross2016).
Calcium-carbonate rich substances (especially when suspected as anthropogenic, e.g., floor, plaster, installation)—were analyzed with the FTIR-based “Grinding curve” method (Regev et al. Reference Regev, Poduska, Addadi, Weiner and Boaretto2010) to help determine their formation mechanism. Ceramic, flint, and stone artifacts were documented and collected for further processing as part of this research. The location, layout, and identification of such artifacts helped in understanding the context of the associated organic samples and to determine valid candidates for 14C dating.
Preparation and Dating of Organic Samples
Organic samples were examined and analyzed to determine their exact context, related material culture, and adjacent loci. Short-lived organic samples found in the same contexts as related material culture, and with unlikely intrusion from other layers, were regarded as candidates for 14C dating of their contexts.
Charred samples were identified taxonomically using a Leica M80 binocular microscope at 10–50× magnification. Charred samples chosen for dating were pretreated for removal of carbonates and humic substances using the ABA (acid–base–acid) protocol (Yizhaq et al. Reference Yizhaq, Mintz, Cohen, Khalaily and Weiner2005; Rebollo et al. Reference Rebollo, Cohen-Ofri and Popovitz-Biro2008). After pretreatment, charred material was analyzed by FTIR to verify the absence of clay and carbonates. Percent of efficiency of the pretreatment (eff.%) was recorded as the ratio between the final weight after preparation and the initial weight of the sample.
Combustion and graphitization of pretreated botanic samples were done in the Kimmel Center for Archaeological Science at the Weizmann Institute of Science. The Hazor pretreated samples numbered up to RTD-9235 were combusted to CO2 in vacuumed quartz tubes with 200 mg of copper oxide heated to 900°C for 200 min. The CO2 was reduced to graphite using 2 mg of iron as a catalyst and hydrogen, which was heated to 600°C for 20 hr. The percentage of carbon in the pretreated material (C%) was calculated from the CO2 pressure obtained after combustion and the amount of material used. Pretreated samples numbered from RTD-9844 and above were combusted and graphitized using the Vario Isotope Select Elemental Analyzer (EA), coupled with the AGE-3 graphitization system (Wacker et al. Reference Wacker, Nemec and Bourquin2010) at the D-REAMS lab. Pretreated samples weighed in tin cups were dropped into the EA combustion tube, wherein the presence of external oxygen flash combustion occurred at a temperature of 950°C. CO2 was separated from the rest of the gaseous combustion products and transferred by Helium carrier gas into the AGE-3 graphitization unit, where carbon was converted to graphite through reduction by hydrogen over iron as a catalyst.
The clean graphite 14C content was measured by the D-REAMS accelerator (Regev et al. Reference Regev, Steier and Shachar2017). 14C ages were reported in conventional radiocarbon years BP (Before Present, where “present” is defined as the year 1950) following international conventions (Stuiver and Polach Reference Stuiver and Polach1977). All calculated 14C ages have been corrected for the isotopic fractionation to be equivalent to the standard of δ13C value of –25‰ (Stuiver and Polach Reference Stuiver and Polach1977). The 14C ages were calibrated using the OxCal 4.4 online version (https://c14.arch.ox.ac.uk/oxcal/OxCal.html) and the IntCal20 calibration curve (Reimer et al. Reference Reimer, Austin and Bard2020). Bayesian probability modeling and related tools in OxCal v 4.4 (Bronk Ramsey Reference Bronk Ramsey2009) were applied to the 14C measurements using the sequence of the dated samples based on their stratigraphy to increase the precision of the calibrated results.
RESULTS
Figure 3 is a schematic section of the probe excavation, presenting the main features that were revealed within the excavation. Table 1 aligns between parallel contexts of the probe excavation and the Hazor excavations (Ben-Tor et al. Reference Ben-Tor, Zuckerman, Bechar and Sandhaus2017). The upper layer (loci L100 to L108) extending down approximately 80 cm contained sediment with sherds and charcoals but no architectural remains, of which the uppermost 30 cm (loci L100 to L103) included a high concentration of bones. Below this upper layer, an IBA plastered installation was revealed. This installation was filled with cobblestones (L.109 on the higher layer and L.115 on the lower layer) and a complete IBA cooking pot (L.111). The globular hand-made cooking pot (Figure 4) can be ascribed to the IBA “Northern Family” (Dever Reference Dever1980: fig. 2:4) and has parallels from Hazor and other IBA sites in Northern Israel (e.g., Bechar Reference Bechar2017b: Fig. 6.3:4,5,7; Eisenberg Reference Eisenberg2012: Fig. 44:1-3; Tadmor Reference Tadmor1978: Fig. 5). Two samples from seed clusters between the installation cobblestones in L.109 and L.115 (RTD 9232 and RTD 9235, respectively) and an olive pit sample from within cooking pot L.111 (RTD 9233) were dated (Table 2).
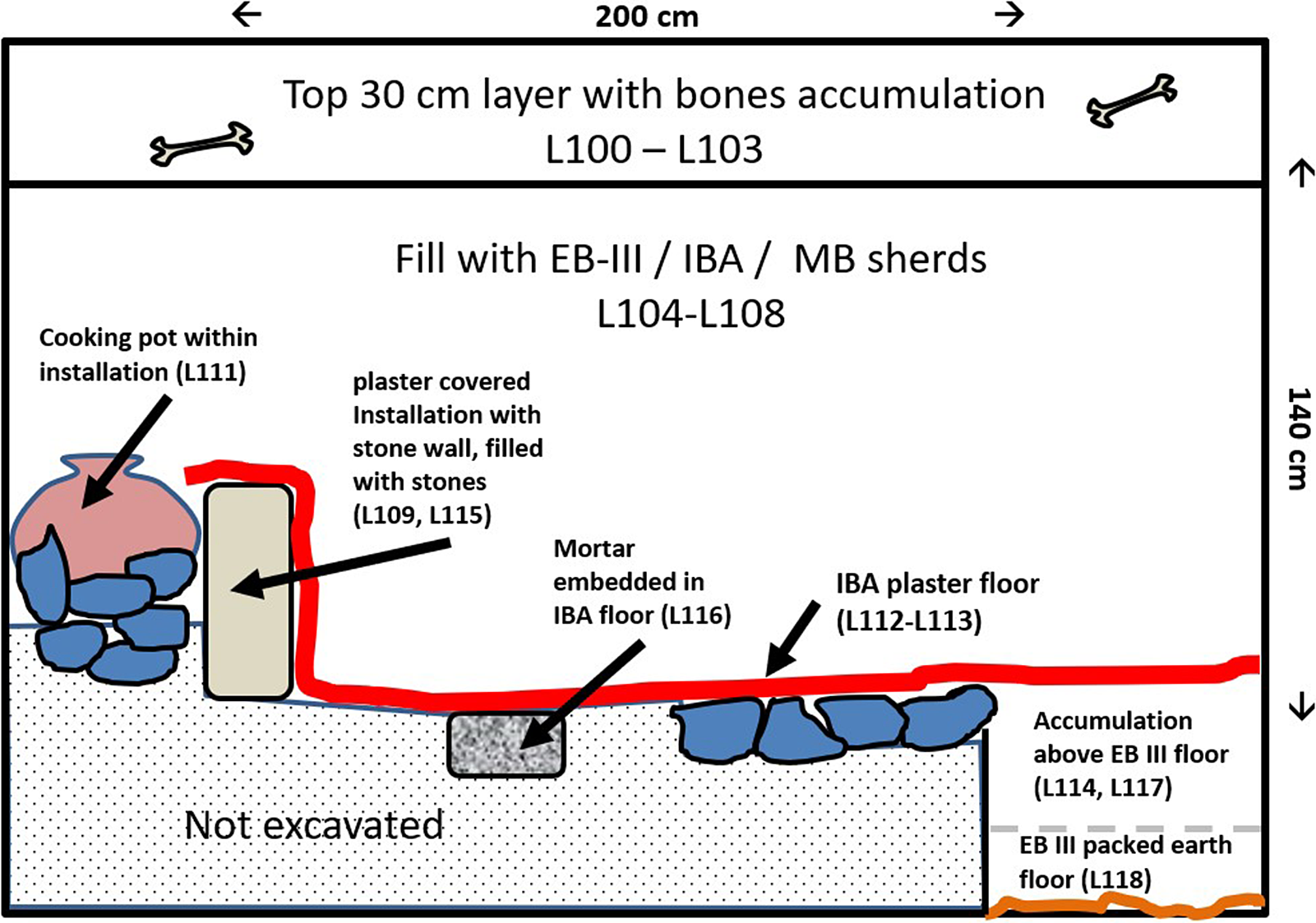
Figure 3 Schematic cross-section of the 2017 probe excavation at Hazor.
Table 1 Parallel contexts of the Hazor excavations (Ben-Tor et al. Reference Ben-Tor, Zuckerman, Bechar and Sandhaus2017) and the 2017 probe excavation.


Figure 4 Cooking pot (L.111) that was found inside the IBA installation after restoration.

Figure 5 IBA excavated area in Tel Hazor 2017, looking north. IBA plastered installation with stones and embedded cooking pot on the left. Plaster floor L.113 of the IBA is on the right. Embedded mortar L.116 within the IBA floor is indicated by the red arrow. The scale bar is 50 cm. The inset on the upper right corner shows the mortar after clearing it. Looking north (photo: Ron Lev).
Table 2 14C dates and chemical treatment data of dated samples from the probe excavation. Samples are ordered according to stratigraphy.

A plaster floor (L.113) was found to the east of the installation, with a basalt mortar embedded in it (L.116, see Figure 5). The plaster floor was found at the elevation of IBA floor L.80006, attributed to Stratum XVIII. Floor L.80006 abutted the baulk, which was excavated within the framework of this study (see Figure 2). The Hazor excavators did not unearth any architectural remains on or above this floor, yet they have already noted the related installation in the baulk (Bechar Reference Bechar2017a: 14). Thus, floor L.113 is the northern continuation of floor L.80006. The plaster floor extended and covered the installation, creating a single unit. The plaster was analyzed (via FTIR “grinding curve” method) as packed powdered limestone. An olive pit sample found resting on floor L.113 (RTD 9234) and an olive pit sample found within mortar L.116 (RTD 9847) were dated (Table 2). A small plaster patch of a different texture on the installation wall indicates a mending operation (Figure 6). This unique plaster patch included charred straw temper, which was mechanically extracted from a plaster sample while being viewed with a binocular microscope in a sufficient amount for 14C dating (Figure 7) and was dated (RTD 9845, Table 2).

Figure 6 The IBA packed ground limestone “plaster” surfaces: (1) floor L.113; (2) plastered installation from the floor and up; (3) negative of the embedded cooking pot (L.111), after its removal; (4) patch with charred straw binders applied on the original installation “plaster.” The scale bar is 10 cm, looking northwest (photo: Ron Lev).

Figure 7 (a) cross-section of the plaster patch (L.112, B17) applied to the installation, showing charred material inserted within the plaster as temper; (b) charred material extracted for 14C dating from L.112, B17 plaster patch matrix (photographs taken via binocular microscope Leica M80).
Excavations continued 40 cm below the IBA plastered floor L.113 (L.80006) on the eastern edge of the excavated area, through sediment accumulation with no architectural remains (L.114, L.117), and reached floor L.118, a packed earth floor with pottery sherds and a few clusters of charred seeds on it (Figure 8). Floor L.118 was found at the elevation of floor L.80035, attributed to Stratum XIX which was defined as EB IIIB post-Khirbet Kerak Ware phase (Ben-Tor and Bechar Reference Ben-Tor and Bechar2017: 3). Floor L.80035 is a packed earth floor covered by an ash layer, which continued into the excavated baulk and was associated with walls W.15011 and W.15012 located outside our probe excavation area (Zuckerman and Bechar Reference Zuckerman and Bechar2017: 9, Pl. 1.2). Thus, floor L.118 is the northern continuation of floor L.80035. The concentration of phytoliths sampled from two contexts on floor L.118 was higher than in all other measured contexts in the probe excavation (Figures 9 and 10). FTIR analysis showed that the sediment in the vicinity of the clustered charred seeds was heated to ∼400°C. In sum, the charred seeds cluster, its location directly on the floor, the adjacent heated sediment, and the concentrated deposition of phytoliths, are all proxies for in-situ context, making the short lived remains from L.118 excellent candidates for 14C dating. Two samples, olive pits RTD 9836 and RTD 9246, from two EB III contexts (L.118 and L.114 respectively), were dated (Table 2).

Figure 8 EB III floor (L.118). Note the IBA plaster floor line on the right baulk, about 40 cm above the EB III floor level. The scale bar is 10 cm (photo: Ron Lev).

Figure 9 Phytoliths/g count in various contexts of the sampling probe excavation. The column labels show the locus and basket number. Note the high phytolith counts from L.118, the Stratum XIX EB III floor.

Figure 10 Microscopic view of phytoliths in sediment sampled on-top of packed earth floor L.118 (parallel to Stratum XIX floor L.80035).
All pottery sherds, bones, and flint remains were collected and documented within the probe excavation. Ten sherds of “Black Wheel-Made Ware” (henceforth BWMW), which is a distinct type of IBA pottery found in northern Israel, Lebanon, and Syria (Bechar Reference Bechar2015), were found in the sediment layers up to 60 cm above the IBA plaster floor L.113.
In total, more than a hundred charred botanic samples were located and collected during the excavation, mainly seeds and charred wood above the floors and in the installation, but also from fill layers L.101 to L.108. Over one hundred sediment and plaster samples were collected and analyzed to support context determination. Charred samples were chosen to be dated based on their identification as short-lived remains, their location within the excavation stratigraphy, and analysis of their context (Figure 11). Two charred olive pit samples (RTD-9844 and RTD-9231) from the fill above the IBA architectural remains (L.106 and L.104, respectively) were also dated. The 14C dates of the Hazor samples, together with the calibrated date ranges and the chemical details, are presented in Table 2 and Figure 12.

Figure 11 Find locations of dated samples marked by their lab number (circled in red). Samples RTD-9231 and RTD-9844 were found in the fill 46cm and 65cm respectively above the IBA floor L.113, and are therefore not presented here (photo: Ron Lev).

Figure 12 The unmodeled calibrated age probability distribution for charred seeds and straw samples from Hazor. Samples are ordered according to site stratigraphy (upper layer on top).
Modeling of Hazor IBA Dated Samples
Based on the stratigraphic relations between excavated contexts, Bayesian modeling was performed on relevant dated samples to define a probable range for each context. The two samples from the fill above the IBA floor, RTD-9231 (46 cm above the floor) and RTD-9844 (65 cm above the floor), do not represent a secure context and were excluded from the modeling exercise. AMS ages from three separate contexts were included in our modeling:
-
Context 1: Seeds found directly on the IBA floor, inside the mortar, and in the in-situ cooking pot (RTD-9234, RTD-9847, RTD 9233);
-
Context 2: Charred straw within the plaster patch on the installation wall (RTD-9845); and
-
Context 3: Seeds found between the cobblestones inside the installation (RTD-9232, RTD-9235).
Samples from context 1 are stratigraphically located above the floor. They mark the end-use of the IBA floor and installation complex and are therefore later than contexts 2 and 3. The stratigraphic order of context 2 and context 3 could not be clearly determined. Age modeling in which samples within the installation (context 3) are later than samples from the plaster patch (context 2) gave a lower than minimum model agreement (Amodel=35). The model in which the installation seeds (context 3) are earlier than the plaster patch (context 2) has a much stronger agreement value (Amodel=115) and was adopted (Table 3, Figure 13). This Bayesian modeling suggests the Hazor IBA occupation phase began in the second half of the 24th century BC and ended not later than 2200 BCE (1σ). The model’s 2σ range for the installation use (context 3) would push the suggested start 100 years earlier, closer to the middle of the 25th century BCE, prolonging the IBA installation lifespan to be 100–200 years, as the installation patch (context 2) 2σ date range starts around 2300 BC. The archaeological evidence for the IBA occupation at Hazor presents a single phase with scant remains, suggesting a shorter IBA occupation period.
Table 3 Bayesian modeling of 14C dates from 3 sequential IBA contexts at Hazor.


Figure 13 IBA sequential dating model of three phases (from earlier to later): 1-Seeds inside installation. 2-Plaster patch. 3-Seeds above the floor.
DISCUSSION
Tel Hazor is one of the most important southern Levantine sites for IBA research as it holds substantial IBA remains directly above the ruins of its EB III city. The new 14C dates obtained in this research clearly show a gap of over 100 years and probably more than 200 years between L.118 and L.112 (L.80035 and L.80006 respectively in Hazor excavations). The architecture of the IBA features revealed at Hazor is uniform, similar in nature, and is interpreted as being contemporaneous (Bechar Reference Bechar2017a: 18). Hazor excavators also identified floor L.80035 as belonging to the last phase of Hazor EB III city (Zuckerman and Bechar Reference Zuckerman and Bechar2017: 9, 149). On this basis, we suggest that the dates presented here reflect a chronological gap between the end of the last EB III city (Stratum XIX) and the IBA settlement (Stratum XVIII) at Hazor (see Figure 12). Such a gap was assumed at two other southern Levantine sites west of the Jordan river: Beth-Shean, based on differences in the pottery assemblage (Mazar Reference Mazar2012: 28), and Jericho, based on stratigraphic observations (Nigro Reference Nigro2003: 131, 138). At Hazor, however, we now have clear 14C-based chronological evidence for a gap that probably extended over many decades, negating any direct relation between the new IBA settlement and the previous EB III city. The absolute date ranges of short-lived samples which were obtained from the post-Khirbet Kerak Ware phase of the EB III city at Hazor (RTD-9236 and RTD-9846 from L.80035, Stratum XIX) end by 2580 BCE, aligned with the “High Chronology” for the EBA in the southern Levant (Regev et al. Reference Regev, de Miroschedji, Greenberg, Braun, Greenhut and Boaretto2012). The latest EB III phase in Beth-Shean, another major EB III city in the region, was also identified as a post Khirbet Kerak Ware phase: Stratum R-7a in Area R (Mazar Reference Mazar2012: 27). Recently published 14C dates from Bet Yerah (RTT 5854 and RTT 5852), collected from the accumulation above an EB III period D floor (Regev et al. Reference Regev, Paz, Greenberg and Boaretto2019: 69, table 2) are similar to the dates collected from the Stratum XIX floor of Hazor presented above. Assuming the post Khirbet Kerak Ware layers mark the last phase of EB III cities in the region before their demise, the new 14C dates from Stratum XIX at Hazor suggest that the end of EB III cities in the Hula and Jordan Valleys is synchronized with that of other EB III cities in the southern Levant. The end of those cities was 14C dated, and they all end by 2500 BCE, including Hebron, Numeira, Tel Yarmuth, Megiddo, and Tel es-Safi (Regev et al. Reference Regev, de Miroschedji, Greenberg, Braun, Greenhut and Boaretto2012, Reference Regev, Finkelstein, Adams and Boaretto2014; Shai et al. Reference Shai, Greenfield, Regev, Boaretto, Eliyahu-Behar and Maeir2014). The absolute dates of the IBA in Hazor have great chronological implications for the relative dating of IBA ceramic assemblages. Using these dates together with the rich ceramic assemblages published from Hazor (Bechar Reference Bechar2017b) will allow the identification of chronological anchors in the ceramic assemblages throughout the Hula Valley and beyond. Ceramic assemblages of the IBA are characterized by regional features (Dever Reference Dever1980; D’Andrea Reference D’Andrea2014), but they are also known to have interregional ceramic traditions and similarities throughout the country. Therefore, the absolute dates of the Hazor assemblage will also have significant implications for the interpretation of assemblages found outside the Hula Valley. For example, the dated samples above the IBA floor were retrieved from the same context as BWMW sherds. The modeled date range of samples obtained from above the IBA floor provides us with an absolute timeframe for the presence of BWMW pottery vessels at Hazor: 2300–2200 BCE (2σ). This is a key date range for BWMW since Hazor has the largest assemblage of BWMW samples in the southern Levant (Bechar Reference Bechar2015). This timeframe indicates that BWMW vessels were in use at Hazor in the middle of the IBA, synchronous with the beginning of the EB IVB in the Northern Levant (D’Andrea Reference D’Andrea2018), where similar vessels appeared, e.g., in Byblus, Yabrud, and Tel Hizin (Bechar Reference Bechar2015).
CONCLUSION
This research was set to examine the absolute dates of the end of the EB III city at Hazor and the IBA settlement at the site. These dates were obtained within a probe excavation that was conducted at the site, adjoining previously excavated EB III and IBA floors. The dates suggest many decades of abandonment between the last EB III city phase and the rural IBA settlement established above it. The date of the latest EB III phase at Hazor is contemporary to the last phases of other EB III cities in the southern Levant. The dates obtained from the context above the IBA floor at Hazor presents a limited date range in the middle of the IBA and can help to refine the chronology of related IBA pottery types.
ACKNOWLEDGMENTS
The Exilarch Foundation supported the radiocarbon research for the Dangoor Research Accelerator Mass Spectrometer (D-REAMS) Laboratory. We wish to thank the Kimmel Center for Archaeological Science and George Schwartzman Fund for funding support to RL, laboratory, and material analysis. EB is the incumbent of the Dangoor Professorial Chair of Archaeological Sciences at the Weizmann Institute of Science.