Introduction
Aquaculture development is prominently based on the growth, development, and health of farmed species in which finfish predominates. Finfish production in Asia amounts to >80% of the global total cultured fish yield (FAO, 2018). According to a recent FAO report, among aquaculture harvest, finfish dominated global fish production in 2018, amounting to 54.3 million tonnes (MT); largely 47 MT contributed from inland while the remaining 7.3 MT from marine sources (FAO, 2020).
Quality feed is important for scaling up fisheries outturn by fulfilling the balanced nutritional requirements of fish. Combating opportunistic pathogens, effects of anti-nutrients and antimicrobial substances in the food and gut environment of fish are also of major concern. Growing focus is presently on supplementing feed for additional health benefits in terms of promulgating gastrointestinal development, augmenting digestive enzyme activity, immunoregulation, and ameliorating disease resistance, thereby improving overall fish health beyond managing preeminent dietary demands. Formulating feed based on the nutrition and health needs of fish is thus an imperative task. In this regard, the use of probiotics, prebiotics-based functional feeds, and in preference, their synergic combination as ‘synbiotics’ has gained momentum. Vouched for their safety and immunostimulating effects, these feed additives are believed to be future biotherapeutics for fish health and welfare. Present work evaluates developing strategies in the use of functional feeds to fulfil finfish aquaculture demands of quantity as well as the quality of produce based on improved health status and well-being of fish. In this aspect, taming the fish gastrointestinal tract (GIT) microbiota through dietary manipulations also provides capacious promise.
Fish gastrointestinal tract ‘microflora’: role and need of supplementation in diet
The GIT of all vertebrates and consequently fish harbours a complex consortium of a resident microbial community regulating host nutrition and health status. The terms ‘microbiota’ and ‘microflora’ are designated to this vast diversity of microbial population which colonize and coexist within the host ecosystem (Neish, Reference Neish2009). GIT microbiota of fish have been categorized as ‘autochthonous’ indigenous, adherent microbiota, and ‘allochthonous’ transient, exogenous microbiota (Berg, Reference Berg1996; Nayak, Reference Nayak2010; Ringø et al., Reference Ringø, Zhou, Vecino, Wadsworth, Romero, Krogdahl, Olsen, Dimitroglou, Foey, Davies, Owen, Lauzon, Martinsen, De Schryver, Bossier, Spersta and Merrifield2016). Aerobic, anaerobic, obligate anaerobic, and facultative-type bacteria are the chief inhabitants in the GIT of fish (Llewellyn et al., Reference Llewellyn, Boutin, Hoseinifar and Derome2014). It is estimated that 107–1011 bacteria per gram of intestinal content colonize fish gut (Nayak, Reference Nayak2010). Studies indicate the existence of intra- and inter-specific variations among gut microbial communities with bacterial count often varying according to age, environmental factors, and more importantly nutrition status of fish (Gomez and Balcazar, Reference Gomez and Balcazar2008). Distinguished variations in microbial density, composition, and function depending on the physicochemical conditions in fish GIT is also observed (Zhou et al., Reference Zhou, Shi, Yao, He, Su and Ding2007; Clements et al., Reference Clements, Angert, Montgomery and Choat2014), with a continuous increase stomach onwards to distal regions of gut (Cahill, Reference Cahill1990; Ringø et al., Reference Ringø, Sperstad, Myklebust, Refstie and Krogdahl2006). Dominant microbial phyla representing 90% of marine and freshwater fish comprise the phyla Proteobacteria, Fusobacteria, and Firmicutes, along with Bacteroidetes, Actinobacteria, and Verrucomicrobia to a nominal extent (Larsen et al., Reference Larsen, Mohammed and Arias2014; Hennersdorf et al., Reference Hennersdorf, Kleinertz, Theisen, Abdul-Aziz, Mrotzek, Palm and Saluz2016; Tarnecki et al., Reference Tarnecki, Burgos, Ray and Arias2017). Genera Acinetobacter, Corynebacterium, Alteromonas, Flavobacterium, Micrococcus, Vibrio, and Pseudomonas are preeminent microbiota of marine fish (Cahill, Reference Cahill1990; Onarheim et al., Reference Onarheim, Wiik, Burghardt and Stackebrandt1994; Blanch et al., Reference Blanch, Alsina, Simon and Jofre1997; Nayak, Reference Nayak2010; Wang et al., Reference Wang, Ran, Ringø and Zhou2018); whereas in freshwater fish, prominent enteric microbiota comprise Aeromonas, Pseudomonas, Bacteroides type A; whilst to a lesser extent Plesiomonas, Micrococcus, Acinetobacter, Clostridium, Enterobacteriaceae, Fusarium, and Bacteroides type B (Sugita et al., Reference Sugita, Tokuyama and Deguchi1985; Gomez and Balcazar, Reference Gomez and Balcazar2008; Wang et al., Reference Wang, Ran, Ringø and Zhou2018). All these groups characteristically include allochthonous and autochthonous microbiota (Tarnecki et al., Reference Tarnecki, Burgos, Ray and Arias2017).
Externally acquired microflora of fish GIT, the allochthonous’ microbial group, are mainly associated with ingested food and lack the ability to colonize the epithelial surface of the intestine or are outcompeted on the basis of competitive exclusion by endogenous bacteria adherent to mucus and epithelium of fish GIT (Ringø and Birkbeck, Reference Ringø and Birkbeck1999; Ringø et al., Reference Ringø, Zhou, Vecino, Wadsworth, Romero, Krogdahl, Olsen, Dimitroglou, Foey, Davies, Owen, Lauzon, Martinsen, De Schryver, Bossier, Spersta and Merrifield2016). Autochthonous microbiota can successfully inhabit the epithelial surfaces of the digestive tract resisting the action of acidic gastric juice, bile, and make up the core community colonizing the host tissues (Ringø and Birkbeck, Reference Ringø and Birkbeck1999). Based on the deep sequencing technique using 16S ribosomal RNA (rRNA) gene sequence comparisons, the concept of core community was investigated in zebrafish, Danio rerio. According to the results, despite drastic differences among life history and domestication status of the host, marked continuity in community structure of core communities prevails (Roeselers et al., Reference Roeselers, Mittge, Stephens, Parichy, Cavanaugh, Guillemin and Rawls2011). Similar observations are supported by meta-analysis of 16S rRNA gene sequence data of teleost gut communities suggesting the tendency for conspecific fish to foster similar gut bacteria substantiating commonality of core gut communities across a wider range of fish (Sullam et al., Reference Sullam, Essinger, Lozupone, O'Connor, Rosen, Knight, Kilham and Russell2012). Evidently, a stable core microbiome is thus present within fish species across environments, and such microbial signatures, unrestrained of host phylogeny, are strongly governed through environmental factors (Roeselers et al., Reference Roeselers, Mittge, Stephens, Parichy, Cavanaugh, Guillemin and Rawls2011). Incidentally, it was indicated that an environmentally regulated fish species-based continuum of core microbials which can be influenced under modulatory effects of putative probiotic strains. Autochthonous gut bacteria in fish are known to assist digestion and nutrition by the production of extracellular enzymes (Cahill, Reference Cahill1990). These distinctive microbe-derived digestive enzymes available in the GITs of fish are in addition to endogenous digestive enzymes produced by the fish itself. Exoenzyme-producing bacteria in fish are involved in various proteolytic, amylolytic, lipolytic, cellulolytic, chitinolytic, and phytolytic activities (Roy et al., Reference Roy, Mondal and Ray2009; Das et al., Reference Das, Mandal, Khan, Manna and Ghosh2014; Rajasekaran et al., Reference Rajasekaran, Sivakumar and Mathialagan2014; Simora et al., Reference Simora, Ferdinand, Traifalgar and Legario2015; Armada and Simora, Reference Armada and Simora2016). Utilizing the potential of enzyme-producing bacterial isolates as probiotics in feed formulation can provide cost-effective aquafeeds, especially when the enzyme system is not efficiently developed, as in larval stages (Rajasekaran et al., Reference Rajasekaran, Sivakumar and Mathialagan2014).
Superfluous bacterial populations intrinsic to gut mucosal surfaces are the first line of defence toward the pathogenic challenge. Any perturbations in the microbial bionomics can lead to dysbiosis or dysregulated flora often associated with a disease state (Vyas and Ranganathan, Reference Vyas and Ranganathan2012). Pathogenic bacteria are the main reason for infectious disease inflicted mortality in wild capture fish as well as in aquaculture fish reserves. Virulent bacterial species of finfish include (1) Vibrio anguillarum (Bergeman, 1909) causing vibriosis in many marine fishes; (2) pathogenic Bacillus sp. septicaemia and bacillary necrosis in various fresh water fish; (3) Clostridium botulinum causing botulism in salmonids; (4) Aeromonas hydrophila hemorrhagic septicaemia and motile Aeromonas septicaemia produced in most fresh water fish species; (5) Aeromonas salmonicida furunculosis in salmonids; (6) Vagocococcus salmoninarum septicaemia in Atlantic salmon; (7) Micrococcus luteus micrococcosis in rainbow trout; (8) Mycobacterium sp. mycobacteriosis in most species; (9) Staphylococcus aureus eye diseases in red sea bream, carp; (10) Edwardsiella ictaluri enteric septicaemia in catfish; (11) Yersinia ruckeri enteric redmouth in salmonids; (12) Flavobacterium sp. gill diseases in turbot, barramundi, and many fresh water fishes; (13) Pseudomonas sp. bacterial hemorrhages in most freshwater fish species; (14) Vibrio alginolyticus pathogen of several marine fishes (such as silver sea bream, cobia, grouper, Asian seabass, epidemic vibriosis in large yellow croaker, eye diseases, septicaemia in groupers); and (15) Streptococcus difficilis meningoencephalitis in carp, trout, silver pomfret, and tilapia (Austin and Austin, Reference Austin and Austin2007; Rameshkumar et al., Reference Rameshkumar, Nazar, Pradeep, Kalidas, Jayakumar, Tamilmani, Sakthivel, Samal, Sirajudeen, Venkatesan and Nazeera2017). With prolonged stressors such as environmental changes (pH, temperature, salinity of water), indiscriminate antibiotic and chemical use with intensification of aquaculture activities, expanse as well as host range of opportunistic fish pathogens is broadening, leading to the emergence of unknown disease outbreaks and newer pathogens (Sudheesh et al., Reference Sudheesh, Al-Ghabshi, Al-Mazrooei and Al-Habsi2012; Llewellyn et al., Reference Llewellyn, Boutin, Hoseinifar and Derome2014). Among these emerging pathogens belong Plesiomonas shigelloides, Sphingomonas paucimobilis, Acinetobacter spp., and Stenotrophomonas maltophilia, and the Gram-positive it is bacteria Lactococcus garviae and Streptococcus iniae. Infections caused by the Gram-positive bacterium Kocuria rhizophila have been reported in recent years, which earlier was considered to be non-pathogenic to fish. (Pękala-Safińska, Reference Pękala-Safińska2018).
Improperly apportioned commensal gut microbiomes and opportunistic bacteria can cause disruption of host homeostasis. In such constrained disposition, reinstitution of fish health status becomes an imperative and resolute task. Significant movement is witnessed toward the application of phages in this field, as both a prophylactic as well as a therapeutic measure, ‘phage therapy’, against various bacterial infections (Laanto et al., Reference Laanto, Bamford, Ravantti and Sundberg2015; Zaczek et al., Reference Zaczek, Weber-Dąbrowska and Górski2020). Abundance and ubiquity of phages, narrow host range, along with target specificity for bacteria, makes phage therapy a plausible alternative to untoward antibiotic fallouts (Richards, Reference Richards2014; Kowalska et al., Reference Kowalska, Kazimierczak, Sowińska, Wójcik, Siwicki and Dastych2020). Phage therapy in aquaculture is extended toward the prevention and treatment of challenging bacterial outbreaks in fishes and consequently aquatic environments (Silva et al., Reference Silva, Costa, Pereira, Mateus, Cunha, Calado, Gomes, Pardo, Hernandez and Almeida2014; El-Araby et al., Reference El-Araby, El-Didamony and Megahed2016; Huang and Nitin, Reference Huang and Nitin2019; Akmal et al., Reference Akmal, Rahimi-Midani, Hafeez-ur-Rehman, Hussain and Choi2020) alongside the immune modulatory effects of phage preparations; decreasing disease-based mortality, improving the survivability of farmed fish (Schulz et al., Reference Schulz, Robak, Dastych and Siwicki2019a, Reference Schulz, Pajdak-Czaus, Robak, Dastych and Siwicki2019b). Choice of the phage, life history stage of fish at which therapy is applied (egg, larvae, juvenile, or adult), multiplicity of infection (the ratio of phage particles to host), dosage, as well as mode of phage delivery (feedable, immersion, injectable, etc.) are key considerations in the success of the treatment (Silva et al., Reference Silva, Costa, Pereira, Mateus, Cunha, Calado, Gomes, Pardo, Hernandez and Almeida2014; Schulz et al., Reference Schulz, Robak, Dastych and Siwicki2019a). There is a paucity of recognizably developed commercial phage formulations and considerable data on phage effective doses in aquaculture applications (Silva et al., Reference Silva, Costa, Pereira, Cunha, Calado, Gomes and Almeida2013; Culot et al., Reference Culot, Grosset and Gautier2019), and much work is warranted in this area. Technical issues of phage stability and storage in commercial preparations (Malik et al., Reference Malik, Sokolov, Vinner, Mancuso, Cinquerrui, Vladisavljevic, Clokie, Garton, Stapley and Kirpichnikova2017) as well as global approval of therapy requires due addressal for successful establishment of phage therapy as large-scale industrial use (Culot et al., Reference Culot, Grosset and Gautier2019). Moreover, problems of limited host range, limited specificity, and bacterial phage resistance (Laanto et al., Reference Laanto, Bamford, Laakso and Sundberg2012; Kowalska et al., Reference Kowalska, Kazimierczak, Sowińska, Wójcik, Siwicki and Dastych2020) should be addressed. Phage combinations as mixtures of strains/types (Castillo and Middelboe, Reference Castillo and Middelboe2016; Chen et al., Reference Chen, Yuan, Liu, Mai, Yang, Deng, Zhang, Liu and Ma2018a) or compounded to other antimicrobials (Chandrarathna et al., Reference Chandrarathna, Nikapitiya, Dananjaya, De Silva, Heo, De Zoysa and Lee2020), ‘phage cocktails’; are believed as suggestible solutions to overcome these shortcomings. Regulated use in terms of type and number of strains added is required to explore their augmentative potential in cocktails (El-Araby et al., Reference El-Araby, El-Didamony and Megahed2016).
Introducing beneficial bacteria into formulated fish diets for effective colonization in the fish gut tract can therefore largely be rewarding for commercial aquaculture practice (Ramachandran and Ray, Reference Ramachandran and Ray2007; Askarian et al., Reference Askarian, Kousha, Salma and Ringø2011; Saha and Ray, Reference Saha and Ray2011; Simora et al., Reference Simora, Ferdinand, Traifalgar and Legario2015). The commensal gut microbiota has the capacity to prevent pathogenic infection by numerous mechanisms based on competitive exclusion exhausting nutrition resources of pathogens, niche exclusion by colonizing mucosal tissues and other tissue sites preventing pathogenic adhesion, and antagonism by the production of antimicrobials. Endogenous microflora by means of bacterial antagonism enables the host immune system to instigate heightened response against pathogens restraining pathogenic adherence in the gut tract. Commensal microbes can compete with pathogens by quenching siderophores (low-molecular-weight iron-chelating molecules with high specific affinity) produced by the pathogen or by means of producing siderophores of very high affinity toward iron (de Bruijn et al., Reference de Bruijn, Li, Wiegertjes and Raaijmakers2017). Many probiotic bacterial strains additionally possess a specialized mechanism of quorum-quenching (QQ) by producing quorum-quenching enzymes to carry out the feat of virulence regulation (Zhou et al., Reference Zhou, Zhang, Yin and Chu2016; Haridas and Pillai, Reference Haridas and Pillai2019). Quorum-quenching can cause enzymatic disruption of bacterial quorum sensing (QS) capacity thus inhibiting the gene expression controlling bacterial pathogenic repertoire. QQ has been a recognizable non-antibiotic strategy toward downregulating bacterial virulence (Grandclément et al., Reference Grandclément, Tannières, Moréra, Dessaux and Faure2016).
As is thoroughly acclaimed, healthy gut microbial communities in fish have an essential role in the establishment of normal gut function (Bird et al., Reference Bird, Conlon, Christophersen and Topping2010; Liu et al., Reference Liu, Guo, Gooneratne, Lai, Zeng, Zhan and Wang2016) including digestion of food, production of accessory digestive enzymes, development of microvasculature of the intestinal villi (Stappenbeck et al., Reference Stappenbeck, Hooper and Gordon2002), biological nitrogen fixation (McDonald et al., Reference McDonald, Watts and Schreier2019), production of secondary metabolites, and secretion of several antipathetic compounds including short-chain fatty acids (SCFAs), lysozyme, siderophores, bacteriocin as ribosomally synthesized antimicrobial peptides that render protection against colonization of opportunistic pathogens (Nayak, Reference Nayak2010; Li et al., Reference Li, Ringø, Hoseinifar, Lauzon, Birkbeck and Yang2018; Mukherjee et al., Reference Mukherjee, Rodiles, Merrifield, Chandra and Ghosh2020); maneuvering this potential can profoundly indemnify host fitness.
Dietary manipulation of GI microbiota in fish: need of supplementation in fish diet
Effect of diet on GI microbiota in fish
Host diet is an important environmental variable directing gut microbiota composition (Ringø and Olsen, Reference Ringø and Olsen1999; Ringø et al., Reference Ringø, Zhou, Vecino, Wadsworth, Romero, Krogdahl, Olsen, Dimitroglou, Foey, Davies, Owen, Lauzon, Martinsen, De Schryver, Bossier, Spersta and Merrifield2016). Fish diet eminently governs the qualitative and quantitative organization of GI bacterial community; respectively in terms of taxonomic composition as well as the relative abundance of taxa. In a study, the impact of diet divergence on the enteric bacterial constitution of sympatric whitefishes Coregonus lavaretus pidschian and C. l. pravdinellus was substantiated (Solovyev et al., Reference Solovyev, Kashinskaya, Bochkarev, Andree and Simonov2019). Remarkable dissimilarities were found in the taxonomical composition of the bacterial communities of both fishes as evident from the study; demonstrating an appreciable role of diet in shaping the composition and corresponding functionality (functional significance) of the gut microbial community. Moreover, it is also noteworthy that diet-associated microbes to a larger extent regulate the diversity of the gut microbiome as in comparison with water-derived microbes (Smith et al., Reference Smith, Snowberg, Caporaso, Knight and Bolnick2015). Food/diet-associated microbes represent a source of potential gut colonizers and with effective colonization, contribute to the diversity of resident gut flora (Givens et al., Reference Givens, Ransom, Bano and Hollibaugh2015). In a 16S rRNA-based sequencing approach comparing microbial communities in the guts of herbivorous and carnivorous fish populations (Givens et al., Reference Givens, Ransom, Bano and Hollibaugh2015), diverse food sources based on varied trophic levels of fish supported a greater diversity of microbiota assemblages into the gut potentially provisioning a wider range of substrates for the core microbial community to act on. Diet-based shifts in microbial community profile had been explored in juvenile rainbow trout Oncorhynchus mykiss with progressive substitution of plant-based protein 0, 50, and 97% in lieu of animal protein in first feed fish diet (Michl et al., Reference Michl, Ratten, Beyer, Hasler, LaRoche and Schulz2017). With plant diet, relative abundance of orders Lactobacillales, Bacillales and Pseudomonadales were significantly enhanced contrastingly to animal protein diet that supported orders Bacteroidales, Clostridiales, Fusobacteriales, Alteromonadales, and Vibrionales. Lactobacillales is an order of lactic acid-producing bacteria (LAB) that promotes carbohydrates fermentation in a plant diet. Lactococcus, a predominant LAB in O. mykiss, markedly inhibits various fish pathogens, and has a protective role during the initial development stages of fish. Order Bacteroidales include several bile-resistant microorganisms that are linked to protein fermentation.
Based on the analysis of the core microbiome, another study suggests a strong modulating effect of diet predominantly at the lower taxonomical levels of common operational taxonomic units (OTUs), because fish feeding either on fishmeal or a plant-derived diet shared merely two OTUs post first diet regime and none after a subsequent feed. The results of the analysis moreover rule out ‘nutritional-programming’ effects of the earliest fed diet on enteric microbiota of trout fry, instead of revealing that diet fed at the time of sampling have an impact on the microbial community provisioning the scope of microbiome manipulation throughout life stages of fish. In a recent study, grass carp Ctenopharyngodon idellus fed on two different diet regimes has been shown to exhibit gut compartment-based segmented microbial profile (Feng et al., Reference Feng, Zhang, Jakovlić, Xiong, Wu, Zou, Li, Li and Wang2019). Gut segments are known to be a major determiner of enteric microbial community composition in land mammals (Ley et al., Reference Ley, Lozupone, Hamady, Knight and Gordon2008) and fish (Gajardo et al., Reference Gajardo, Rodiles, Kortner, Krogdahl, Bakke, Merrifield and Sørum2016). In C. idellus, dietary attuning effects are based on intestinal compartments as diet remains a considerable effector partly toward proximal regions of the gut where bacterial communities are selected-out based on variation in diet alone, whereas in the mid and hindgut compartments, dietary effect is less profound with resulting microbial selection in this region on the basis of anatomy and physiological makeup of intestine. Concomitantly, it is hence worthy for studies on feed or feed-manipulation-impact on the gut microbiome to account for differential impacts on the endogenous and transient microbiota, as well as that of gut compartments.
Diet manipulation through microbial supplementation
The distribution, constitution, and functionality of the gut microflora find a crucial role in the metabolism of feedstuffs, thereby influencing feed utilization, regulating available energy from feed, modulation of immune functions, and stress tolerance, depending on physiological characteristics of fish (Xia et al., Reference Xia, Lin, Fu, Wan, Lee, Wang, Liu and Yue2014). A bidirectional relationship exists between nutrition and the gut microbiome as it is found that the enteric microbial community can both utilize and produce macro as well as micronutrients (Gentile and Weir, Reference Gentile and Weir2018). At the level of GIT, both microbial–microbial and host–microbial interactions manage the course of evolution of host physiology and homeostasis (Neish, Reference Neish2009). This possibly indicates the coevolution of microbial diversity with evolving dynamics of host ecology and vice versa. Such outcomes indicate that microbial–microbial and host–microbiota interactions can conscientiously be manipulated to promote beneficial symbiosis in aquaculture. Diet has an important influence on the gut microbial community. The impact of diet on microbiome structure as a rational link has been explored to study the evolution of microbial flora in various fish species (Givens et al., Reference Givens, Ransom, Bano and Hollibaugh2015; Singh et al., Reference Singh, Bhatnagar, Alok and Ajay2018; Niu et al., Reference Niu, Khosravi, Kothari, Lee, Lim, Lee, Kim, Lim, Lee and Kim2019; Solovyev et al., Reference Solovyev, Kashinskaya, Bochkarev, Andree and Simonov2019). Characterization of gut microbionts is the baseline for future probiotic studies yet issues of the viability of probiotics during GIT transition and in large-scale commercial feed productions need to be addressed. Prebiotic combination to live-microbial-probiotics can potentiate survivability as well as the unhindered establishment of probiotics (Llewellyn et al., Reference Llewellyn, Boutin, Hoseinifar and Derome2014; Terpou et al., Reference Terpou, Papadaki, Lappa, Kachrimanidou, Bosnea and Kopsahelis2019). Pre/pro/synbiotics are being recognized as prospective biotherapeutic alternatives to antibiotic and chemotherapeutic agents as they bear added advantage of being safe, biodegradable, environmentally sustainable compounds with no known residual effects. The use of synbiotics containing probiotics as live-microbial-supplementation in feed/diet is prominently augmenting beneficial microbes as well as host–gut–microbiota interactions through externally procured bacteria. Considering the functional significance of the enteric microbiome in augmenting host fitness, the administration of beneficial microbes may be a promising approach in safeguarding fish from imminent diseases (de Bruijn et al., Reference de Bruijn, Li, Wiegertjes and Raaijmakers2017). Functional feeds based on probiotics and prebiotics, as a combination in synbiotics, aim at establishing the modalities of microbial dynamics to maximize host fitness.
Probiotics in finfish aquaculture
Probiotics are live microorganisms whose adequate administration confers health benefits to the host (FAO/WHO, 2002). Increasing concerns over antibiotic usage in extensive aquaculture practices and problems of bioaccumulation, residual effects as well as the proliferation of antibiotic resistance in microbes, have shifted focus toward alternative, bio-therapeutic compounds such as probiotics for disease treatment and prevention (Balcazar et al., Reference Balcazar, Blas, Ruiz-Zarzuela, Cunningham, Vendrell and Múzquiz2006; Ringø et al., Reference Ringø, Olsen, Gifstad, Dalmo, Amlund, Hemre and Bakke2010). In an aquaculture scenario, probiotic feed supplementation is considered to confer immunoprophylactic control to pathogenic incidence and contribute to the overall health status of fish majorly by improving gut-microbial ecology.
Well-known probiotics used in finfish aquaculture include bacterial genera Bacillus, Lactobacillus, and Bifidobacteria as well as non-pathogenic yeast, mostly genus Saccharomyces. Viability, the stability during storage and at genetic and processing levels as well as health safety, is an essential criterion for characterization of probiotics (depicted in Fig. 1). Some peculiarities of probiotic organisms appropriated to aquaculture are non-pathogenicity, acid tolerance, rapid regeneration, robustness, anti-genotoxicity, genetic stability, survival during technical procedures, as well as lack of resistance toward antibiotics (Rubiolo et al., Reference Rubiolo, Botana, Martínez and Derome2019). Probiotic development is based on the source of isolation whether from within the host (autochthonous) or obtained exogenously (allochthonous). In allochthonous strategy, screening of probiotic properties of candidates isolated from another organism is performed whereas the autochthonous probiotic development targets the native microbiota of the host. Identification, isolation, and characterization of probiotic strain are performed by PCR amplification, gel electrophoresis, and subsequent sequencing of obtained 16S rRNA (Seghouani et al., Reference Seghouani, Garcia-Rangel, Füller, Gauthier and Derome2017; Tarkhani et al., Reference Tarkhani, Imani, Hoseinifar, Ashayerizadeh, Moghanlou, Manaffar, Van Doan and Reverter2020a). Putative probiotic strains isolated from native or allochthonous source can be identified for their probiotic potential based on screening procedures such as in vitro assays as well as in vivo experiments. Generally, isolates that could be stored as cryo-culture are evaluated using specific in vitro assays. In vitro assays were performed by Tarkhani et al. (Reference Tarkhani, Imani, Hoseinifar, Ashayerizadeh, Moghanlou, Manaffar, Van Doan and Reverter2020a); for screening host-associated LAB Enterococcus faecium and commercial isolate Pediococcus acidilactici for roach (Rutilus rutilus caspicus) fingerlings based on their antimicrobial activity toward A. hydrophila and Y. ruckeri, extracellular enzyme production, tolerance to low pH, and high bile concentration as well as safety; whereas in vivo evaluations assessed the effects of dietary administration of candidate probiotic on the growth performance, serum immunity, digestive enzyme activity, and disease resistance.
In another work, native probiotic assessment was performed on flatfishes, Scophthalmus maximus, Platichthys flesus, and Limanda limanda, based on in vitro antagonism toward pathogenic Tenacibaculum maritimum, Edwardsiella tarda, and Listonella anguillarum, synthesis of essential fatty acids (SEFA) and precursors, and saponin metabolization as feed-derived anti-nutrient (Wanka et al., Reference Wanka, Damerau, Costas, Krueger, Schulz and Wuertz2018). Probiotic substances can comprise a single or admix of two or more strains. Effects of probiotics are believed to be peculiar to the strain (Hill et al., Reference Hill, Guarner, Reid, Gibson, Merenstein, Pot, Morelli, Canani, Flint, Salminen, Calder and Sanders2014; Shewale et al., Reference Shewale, Sawale, Khedkar and Singh2014) and even an individual strain can exhibit variable benefits when used separately or in a mixture (Chapman et al., Reference Chapman, Gibson and Rowland2011). Hence proper identification at genus, species, and strain levels, as well as individual and combined behavior of strains in consolidated mixtures, needs to be performed for determining the probiotic potential of microorganisms (FAO/WHO, 2002). A comparative study investigated the growth-promoting effects of a multi-strain probiotic PTX (75,150 mg kg−1 diet) and commercial prebiotic Fermacto (at 3 g kg−1 feed level) in common carp (C. carpio). Best growth-promoting results with a significant increase in body weight, length, and condition factor were ascertained at 75 mg kg−1 PTX probiotic, while considerable effects on meat fat and increased body proteins in C. carpio were obtained with 150 mg PTX per kg feed, although the feed conversion ratio (FCR) was low in treated experimental diet compared to the control (Asadian et al., Reference Asadian, Shahsavani and Kazerani2015). Additionally, the concentration of probiotics has also been found to have an effect on its performance as is substantiated by providing different concentrations of Lactobacillus plantarum 104, 106, and 108 colony-forming unit CFU g−1 kg−1 Nile tilapia diet (Ruiz et al., Reference Ruiz, Owatari, Yamashita, Ferrarezi, Garcia, Cardoso, Martins and Mouriño2020). As compared to other concentrations, probiotic at 108 CFU g−1 level improved number of goblet cells, cordonal aspects, decreasing hepatic obstruction; with improved immunophysiological conditions of fish.
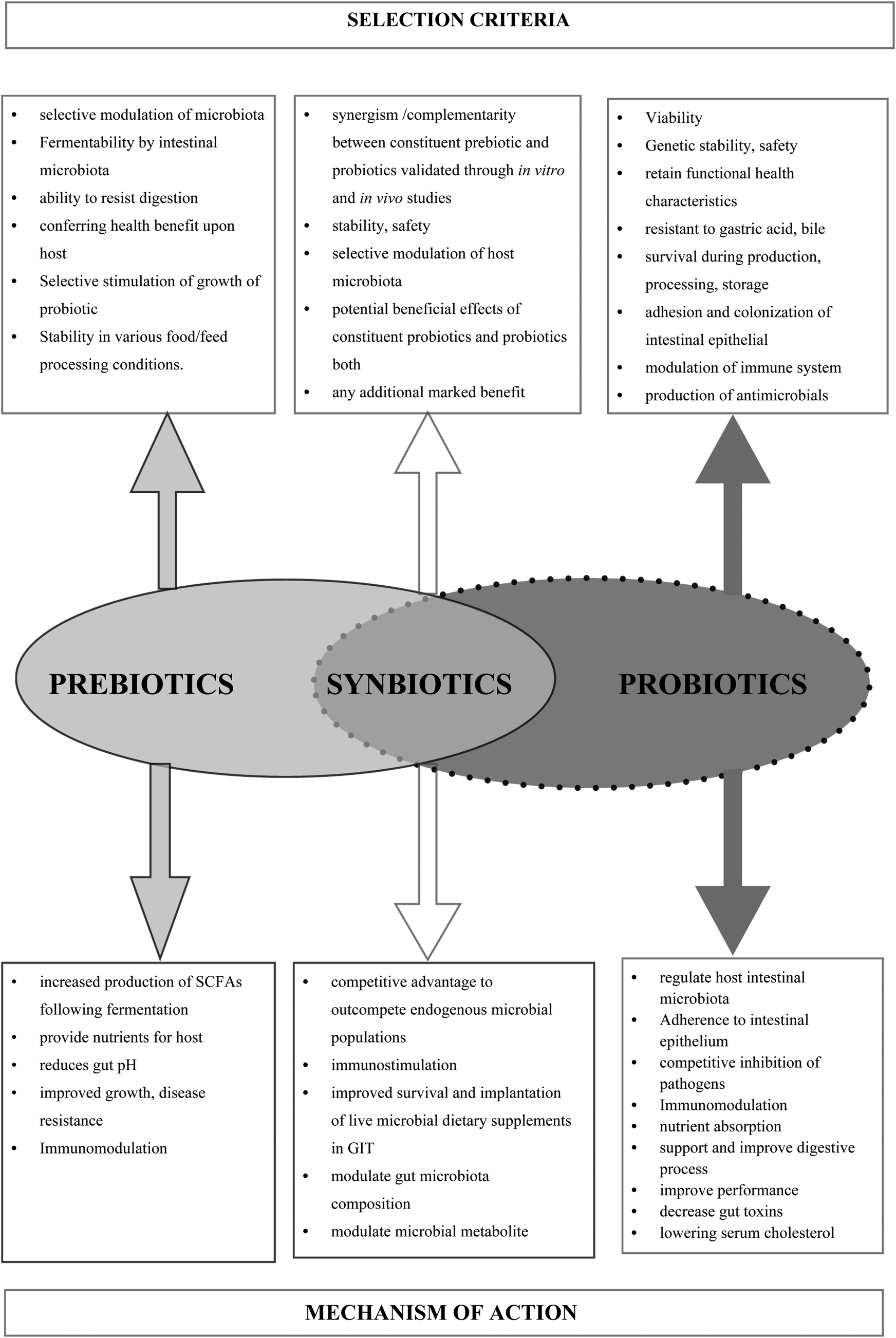
Fig. 1. Mechanism of action and key selection criteria of prebiotics, prebiotics, and synbiotics.
In aquaculture, the definition of probiotics has been reviewed, understating the viability requirement to redefine elaborately as ‘live or dead, or even a component of the bacteria that act under different modes of action’ (that is when added to the water or feed) and ‘confer beneficial effects to the host or to its environment’ (Merrifield et al., Reference Merrifield, Dimitroglou, Foey, Davies, Baker, Bøgwald, Castex and Ringø2010). The applicability of this definition is yet to be established, as many other works have used the term ‘paraprobiotics’ to indicate the use of inactivated microbial cells or cell fractions, products, or microbial metabolic by-products to confer health benefit to the consumer (Taverniti and Guglielmetti, Reference Taverniti and Guglielmetti2011). ‘Paraprobiotics’ are also known as ‘ghost probiotics’, ‘postbiotics’, non-viable probiotics, or inactivated probiotics (Aguilar-Toalá et al., Reference Aguilar-Toalá, Garcia-Varela, Garcia, Mata-Haro, González-Córdova, Vallejo-Cordoba and Hernández-Mendoza2018) and the usage of paraprobiotics in finfish aquaculture has recently been reported in several applications (Li et al., Reference Li, Zhang, Li and Sun2014; Dawood et al., Reference Dawood, Koshio, Ishikawa and Yokoyama2015; Midhun et al., Reference Midhun, Neethu, Vysakh, Radhakrishnan and Jyothis2018). In a work in gilthead seabream (Sparus aurata), the effect of monospecies and multispecies probiotic formulations has been evaluated (Salinas et al., Reference Salinas, Abelli, Bertoni, Picchietti, Roque, Furones, Cuesta, Meseguer and Esteban2008). Heat-deactivated Lactobacillus delbrueckii subspecies lactis and Bacillus subtilis administration, individually and combined, was investigated for their differential systemic, local immunostimulatory roles on adult and juveniles. Adults were fed diets supplemented with 1 × 107 CFU g−1 Lactobacillus, 1 × 107 CFU g−1 Bacillus, 0.5 × 107 CFU g−1 Lactobacillus plus Bacillus for 3 weeks. In adults, multi-species heat-killed bacterial mixture significantly increased natural complement, serum peroxidase, and phagocytic activities while juveniles fed with similar experimental supplementation showed increased total serum IgM, gut IgM+ cells and acidophilic granulocytes with multispecies treatment compared to monospecies probiotics or control fed groups.
Frequently, applications of probiotics in finfish aquaculture feed-supplementation are based on LAB such as Lactobacillus species L. plantarum, L. brevis, L. lactis, L. rhamnosus, L. paracasei; Bifidobacterium bifidum, B. animalis, B. lactis, and Bacillus spp. incuding B. subtilis, B. licheniformis, B. coagulans, and B. amyloliquefaciens. The use of above-mentioned LAB and genus Bacillus has the most promising effects on survival, stress tolerance, growth, and immunological parameters in the studies for a vast variety of fish such as rainbow trout, olive flounder (Paralichthys olivaceus), Nile tilapia (O. niloticus), Dabry's sturgeon (Acipenser dabryanus), rohu (Labeo rohita), Caspian roach (R. r. caspicus), golden pompano (Trachinotus ovatus), zebrafish (D. rerio), fresh turbot (S. maximus), and Acipenser baerii (Lv et al., Reference Lv, Ma, Sun, Lin, Bai, Li and Zhang2018; Van Doan et al., Reference Van Doan, Hoseinifar, Khanongnuch, Kanpiengjai, Unban, Kim and Srichaiyo2018; Di et al., Reference Di, Chu, Zhang, Huang, Du and Wei2019; Mukherjee et al., Reference Mukherjee, Chandra and Ghosh2019; Yi et al., Reference Yi, Liu, Chuang, Chang and Hu2019; Hassani et al., Reference Hassani, Jourdehi, Zelti, Masouleh and Lakani2020; Liu et al., Reference Liu, Wang, Cai, Li, Ren, Wu, Guo, Sun and Zhou2020; Niu et al., Reference Niu, Lee, Kothari, Lee, Hur, Lim, Kim, Kim, Kim and Kim2020; Vazirzadeha et al., Reference Vazirzadeha, Roostaa, Masoumia, Farhadia and Jeffs2020; Tarkhani et al., Reference Tarkhani, Imani, Hoseinifar, Ashayerizadeh, Moghanlou, Manaffar, Van Doan and Reverter2020a, Reference Tarkhani, Imani, Hoseinifar, Moghanlou and Manaffar2020b). Specifically, genera Bacillus has been found to have the most profound effects on pathogenic resistance toward A. hydrophila in L. rohita through probiotic Bacillus methylotrophicus, B. licheniformis (Mukherjee et al., Reference Mukherjee, Chandra and Ghosh2019) and A. hydrophila, A. veronii, A. media, S. iniae by B. subtilis (Di et al., Reference Di, Chu, Zhang, Huang, Du and Wei2019) in A. dabryanus; pathogenic Vibrio ponticus (Macián et al., 2005) by probiotic strain Bacillus pumilus A97 in golden pompano, T. ovatus L. (Liu et al., Reference Liu, Wang, Cai, Li, Ren, Wu, Guo, Sun and Zhou2020). Spores of Bacillus sp. have endured probiotic potential, along with the competence to withstand severe GIT environments as well as technical processing during preparations (Prieto et al., Reference Prieto, O'Sullivan, Tan, McLoughlin, Hughes, Gutierrez, Lane, Hickey, Lawlor and Gardiner2014). Bio-supplementation of fish feed with B. subtilis spores has been employed to prevent challenging diseases in aquaculture environment (Sun et al., Reference Sun, Shang, Tang, Jiang, Dong, Zhou, Lin, Shi, Ren, Zhao, Shi, Zhou, Pan, Chang, Xuerong Li, Huang and Yu2020a, Reference Sun, Zhang, Hui Chen, Wei and Ning2020b). Fervently, the surface-display property of B. subtilis spores has been utilized as a probiotic-based oral vectored vaccine for surface expression of engineered heterologous antigens against various fish diseases (Yao et al., Reference Yao, Chen, Cui, Zhang, Zhou, Guo, Li and Zhang2019; Sun et al., Reference Sun, Zhang, Hui Chen, Wei and Ning2020b). Bacillus subtilis spore display is a potential alternative to classical display organizations because of its improved stability and enhanced safety (Wang et al., Reference Wang, Wang and Yang2017). Bacillus subtilis GC5 spore-based recombinant vaccines GC5-VP4 and GC5-NS38 against reovirus II (GRVII) are found to provide relative percent survival rates of 30 and 36.4%, respectively, in grass carp Ctenopharyngodon idella, with highly improved systemic and mucosal immunity (Chen et al., Reference Chen, Yao, Cui, Zhang, Guo, Zhou and Zhang2019a). Additionally, in a work on tilapia Oreochromis niloticus, GC5-Sip-based recombinant spores could induce effective humoral, innate, local as well as systemic cellular immune responses providing a relative percentage of survival (RPS) of 41.7% against S. agalactiae infections (Yao et al., Reference Yao, Chen, Cui, Zhang, Zhou, Guo, Li and Zhang2019). Bacillus subtilis spores are capable to prevent the degradation of surface-displayed heterologous antigens in a hostile GIT milieu. However, the spores can germinate in GIT (Casula and Cutting, Reference Casula and Cutting2002), which causes loss of the antigens with the disassembly of spore coat. To combat this problem, a novel antigen expression strategy using germination-arrest spore-surface display was developed by a group (Sun et al., Reference Sun, Zhang, Hui Chen, Wei and Ning2020b). Oral administration of GCRV Vp7 antigen germination-arrested spore-coated feed pellets to grass carp elicited augmented immunoprotection in comparison to the administration of wild-type spores thus providing a promising avenue in the development of spore-based display systems.
Nonetheless, probiotics in aquaculture encompass the usage of a vast range of bacteria (Gram-negative or Gram-positive), yeasts, and unicellular algae. Applications on probiotic use of E. faecium, and P. acidilactici yeast Rhodotorula and fungus (Amir et al., Reference Amir, Zuberi, Kamran, Imran and Murtaza2019; Wang et al., Reference Wang, Liu, Sun, Li and Liu2019; Tarkhani et al., Reference Tarkhani, Imani, Hoseinifar, Ashayerizadeh, Moghanlou, Manaffar, Van Doan and Reverter2020a, Reference Tarkhani, Imani, Hoseinifar, Moghanlou and Manaffar2020b) in finfish aquaculture are also growing. Apart from their beneficial effects on growth and immunological parameters, probiotic feeding can greatly empower fish physiology with mechanisms of combatting stressors (pathogens, high-density stocking, water quality parameters-pH, salinity) in aquatic environment. Transcriptome screening and transcriptome expression analysis for apoptosis-related gene was performed to elucidate the impact of functional diets in regulating homeostatic-apoptotic mechanisms in O. mykiss as a response to high-density stocking (Détrée and Gonçalves, Reference Détrée and Gonçalves2019). Genes involved in apoptotic mechanisms were found to be particularly responsive to fish density and diets. It is largely acknowledged that under suboptimal conditions both the neuro-immune endocrine connection and energetic-metabolic machinery are key players in the ability of fish to maintain its overall homeostasis. Trout fed a functional diet with the probiotic yeast Saccharomyces cerevisiae during 30 days persistent density stressor could develop stress mitigation effects through downregulation of genes associated to neuro-immune endocrine connection and genes related to extrinsic pathway, such as death receptors (Fas, TNF-R1), death domain-associated protein as well as initiator and effector caspases. Overall, these results attest to the role of probiotic feeding in the activation of homeostatic machinery and particularly genes involved in the apoptotic pathway, contravening apoptotic molecular repression derived from chronic stress. Current advancements in probiotic research in aquaculture have been directed toward the implementation of commensal, host-derived bacterial isolates, like probiotics. Recently, several studies have advocated the supremacy of the host-associated probiotic supplementation due to their better ability to modulate autochthonous microbiota, enzyme activities, disease resistance as well as immunocompetence of host fish species (Van Doan et al., Reference Van Doan, Hoseinifar, Khanongnuch, Kanpiengjai, Unban, Kim and Srichaiyo2018; Kuebutornye et al., Reference Kuebutornye, Lu, Abarike, Wang, Li and Sakyi2020; Tarkhani et al., Reference Tarkhani, Imani, Hoseinifar, Ashayerizadeh, Moghanlou, Manaffar, Van Doan and Reverter2020a). In aquaculture, many candidate probiotics are being developed from their native fish host, rather than the use of commercial forms derived from terrestrial mammals; probably because of the limited ability of terrestrial probiotic isolates to effectively colonize the GIT of fishes (Gatesoupe, Reference Gatesoupe1999; Lazado et al., Reference Lazado, Caipang and Estante2015; Wanka et al., Reference Wanka, Damerau, Costas, Krueger, Schulz and Wuertz2018). In this respect, putative probiotic strain Bacillus paralicheniformis strain FA6 from grass carp has been found to possess better adhesion and colonization potentials in the host intestine, extending its probiotic effects for longer durations (Zhao et al., Reference Zhao, Wu, Feng, Jakovlić, Tran and Xiong2020). However, in aquaculture, the effective apportionment of probiotics can be tasking with reported loss from leaching in water, low survivability of the bacteria during storage processing, and issues related to handling and feed preparation (Merrifield et al., Reference Merrifield, Dimitroglou, Foey, Davies, Baker, Bøgwald, Castex and Ringø2010). Encapsulation of probiotic as a nutritional strategy can address most of these issues. Microencapsulation of probiotic in aquafeeds has been one of the powerful techniques to attribute safe passage and survivability to probiotic through acidic gastric compartment (Shori, Reference Shori2017; Boonanuntanasarn et al., Reference Boonanuntanasarn, Ditthab, Jangprai and Nakharuthai2019). Additionally, controlled release of entrapped probiotics in the intestine from encapsulating matrix can ensure prolonged and persistent effects. In microencapsulation, generally food-grade biopolymers such as guar gum, alginate, and pectin are employed as an encapsulating matrix for the incorporation of microbial cells. Microencapsulation of probiotic fungus Geotrichum candidum strain QAUGC01 with sodium alginate has been carried out for fingerlings of L. rohita raised in a semi-intensive culture system. For 11 weeks, experimental schedule fed basal diet was supplemented with 109 CFU g−1 unencapsulated and encapsulated G. candidum; respectively (Amir et al., Reference Amir, Zuberi, Kamran, Imran and Murtaza2019). Significantly improved parameters of growth, hemato-immunological parameters (RBCs, hemoglobin (Hb), hematocrit (Ht), WBCs, mean corpuscular Hb concentration (MCHC), respiratory burst activity (RBA) and phagocytic activity (PA), total protein, lysozyme activity (LA), and IgM), intestinal enzyme activities (protease, amylase, and cellulase); upregulated muscle heat shock protein HSP70 gene; reduced serum aspartate aminotransferase (AST) and alanine aminotransferase (ALT) activities, total cholesterol (CHO), triglyceride (TG) in the intestine and liver tissues; in fish fed G. candidum supplemented diets were observed. However, a diet formulated with encapsulated G. candidum rendered the most appreciable effect as compared to its unencapsulated form. Thus, microencapsulation can extend practical and commercial prospects in the application of probiotic feed additives to achieve growth, health status, and immunity in semi-intensive aquaculture systems.
Prebiotics in finfish aquaculture
Prebiotic, as an initial conception in work on colonocytes in humans (Gibson and Roberfroid, Reference Gibson and Roberfroid1995), was described as a non-digestible nutrient substance that beneficially affects the host by selective stimulation of growth and/or activity of colonic bacteria, thus improving host health. Since its introduction, several definitions have been proposed to describe prebiotic compounds (Table 1). The currently accepted definition is in accordance to International Scientific Association for Probiotics and Prebiotics (ISAPP). These nutritional substrates are resistant to digestion, particularly in preliminary regions of the digestive tract and are fermented by endogenous bacteria in the intestinal area to release energy, metabolites, and SCFAs (essentially butyrate, acetate, propionate, and lactic acid) as fermentation by-products. Some of the intestinal bacterial species survive by cross-feeding ( = syntrophy) products of fermentation and metabolites from complex carbohydrate degradation by other bacterial species (Ringø et al., Reference Ringø, Dimitroglou, Hoseinifar, Davies, Merrifield and Ringø2014). SCFA production tends to increase acidity lowering intestinal pH. Acidic gut environment influences the microbial diversity as well as functionality and directly affects host health (Ríos-Covián et al., Reference Ríos-Covián, Ruas-Madiedo, Margolles, Gueimonde, de los Reyes-Gavilán and Salazar2016; Parada Venegas et al., Reference Parada Venegas, De la Fuente, Landskron, González, Quera, Dijkstra, Harmsen, Faber and Hermoso2019). SCFA can thus significantly contribute to modelling the physiology of as well as the ecology of the GIT. Prebiotics can also promote the propagation of probiotics (Gibson and Roberfroid, Reference Gibson and Roberfroid1995). The increased production of SCFAs as an outcome of prebiotic fermentation is contemplated to subserve the prebiotic effects. As known butyrate is a principal energy source for enterocytes (Dimitroglou et al., Reference Dimitroglou, Merrifield, Carnevali, Picchietti, Avella, Daniels, Güroy and Davies2011; Ringø et al., Reference Ringø, Dimitroglou, Hoseinifar, Davies, Merrifield and Ringø2014), while acetate as a major SCFA produced in fishes serves as a substrate for cholesterol biosynthesis (Sharpe et al., Reference Sharpe, Drolet and MacLatchy2006). Found variations in the chemical structure of prebiotic compounds, such as molecular weight, type of linkage involved, and the degree of polymerization (DP), are known for their impact on effective utilization by the gut microflora that in turn regulates SCFA output (Geraylou et al., Reference Geraylou, Souffreau, Rurangwa, Maes, Spanier, Courtin, Delcour, Buyse and Ollevier2013b; Fehlbaum et al., Reference Fehlbaum, Prudence, Kieboom, Heerikhuisen, van den Broek, Schuren, Steinert and Raederstorff2018). For the criteria of selection and detailed action mechanism of prebiotics, see Fig. 1. Prebiotics are considered to be important functional feed additives due to their capacity to influence gut microbiota in a selectively specified manner. Among the most recognized prebiotics for use are carbohydrates that include polysaccharides (inulin and β-glucans) and oligosaccharides (fructooligosaccharides (FOS = oligofructose), galactooligosaccharides (GOS), and mannanoligosaccharides (MOS)). Prebiotic oligosaccharides are low molecular weight carbohydrates with DP ranging 3–10 (Patel and Goyal, Reference Patel and Goyal2011). Most commonly used prebiotics in the aquafeeds for finfish include FOS, short-chain fructooligosaccharides (scFOS), MOS, GOS, and inulin. Although many applications based on novel prebiotics have come to fore, these involve xylooligosaccharides (XOS), arabinoxylooligosaccharides (AXOS), transgalactooligosaccharides, isomaltooligosaccharides (IMOS), as well as commercial prebiotic combinations (Ringø et al., Reference Ringø, Olsen, Gifstad, Dalmo, Amlund, Hemre and Bakke2010; Ganguly et al., Reference Ganguly, Dora, Sarkar and Chowdhury2013; Guerreiro et al., Reference Guerreiro, Serra, Oliva-Teles and Enes2018). Some commercial prebiotics used in fish aquafeeds are listed in Table 2. Additional applications based on chitooligosaccharides in Nile tilapia (O. niloticus) (Meng et al., Reference Meng, Wang, Wan, Xu and Wang2017); alginate oligosaccharide (AlgOS) in Atlantic salmon (Salmo salar) (Gupta et al., Reference Gupta, Lokesh, Abdelhafiz, Siriyappagouder, Pierre, Sørensen, Fernandes and Kiron2019); konjac oligosaccharide (KOS) in ya-fish, Schizothorax prenanti (Chen et al., Reference Chen, Wu, Yan, Lv, He, Feng, Zhao and Duan2018b), have been reported.
Table 1. Variously proposed definitions of prebiotics (source: Kolida and Gibson, Reference Kolida and Gibson2011; Gibson et al., Reference Gibson, Hutkins, Sanders, Prescott, Reimer, Salminen, Scott, Stanton, Swanson, Cani, Verbeke and Reid2017)
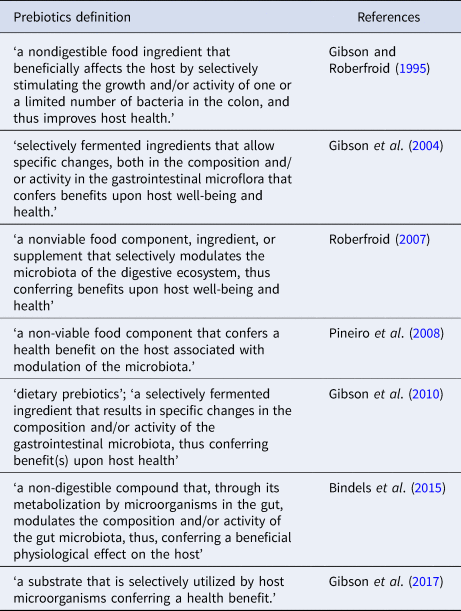
Table 2. Commercial prebiotics and probiotics used in finfish aquafeeds
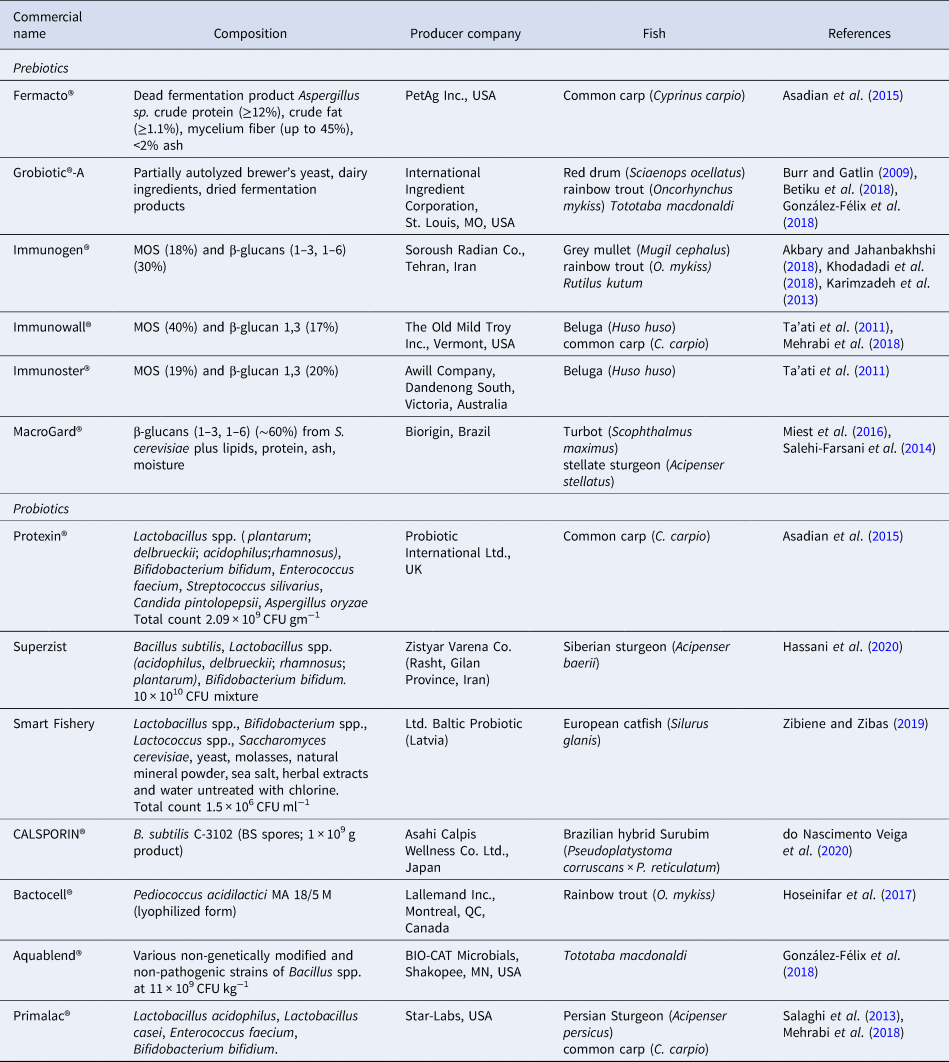
Plant-derived prebiotics
Inulin
Inulin is one of the pre-eminently studied prebiotics, consisting of polydisperse fructan with a DP of 2–60 with D-fructose held through β-(2→1) linkages and terminal D-glucose residue (Sissons and Fellows, Reference Sissons, Fellows, Victor and Zibadi2014). Inulin as a polysaccharide compound is naturally found in many plants including bananas, barley, and Jerusalem artichoke (Roberfroid, Reference Roberfroid2007). It has been reported to have beneficial effects on immune function and overall health parameters in fishes (Mahious et al., Reference Mahious, Gatesoupe, Hervi, Metailler and Olivier2006; Cerezuela et al., Reference Cerezuela, Cuesta, Meseguer and Esteban2008; Akrami et al., Reference Akrami, Abdolmajid, Abbas and Abdolmohammad2009). Inulin supplementation at 15 g kg−1 level has beneficial effects on growth, FCR, and gut microbiota of Asian seabass (Lates calcarifer) (Ali et al., Reference Ali, Ambasankar, Nandakumar, Praveena and Syamadayal2016). One percent dietary inulin inclusion increased total LAB, LA, complement C3, C4 values, with significantly higher blood protein and globulin on inulin supplementation to the diet of common carp, C. carpio (Mousavi et al., Reference Mousavi, Mohammadiazarm, Mousavi and Ghatrami2016). While works in beluga (Reza et al., Reference Reza, Abdolmajid, Abbas and Abdolmohammad2009) and red drum Sciaenops ocellatus (Burr and Gatlin, Reference Burr and Gatlin2009) do not confirm any observed beneficial effects of inulin supplementation on growth parameters, specific growth rate (SGR), energy and protein retention (ER, PR), weight gain (WG), feed efficiency (FER), protein efficiency ratio (PER), or gut microbial diversity, for C. idella (Mo et al., Reference Mo, Cheng, Choi, Lun, Man, Wong, Chen, Lau and Wong2015), dietary inclusion of inulin (0.2, 2% level) along with yeast-cell-wall-derived mannanoligosaccharide (2% MOS) was capable to improve relative weight gain (RWG), SGR, PER, and FCRs.
Fructooligosaccharides (FOS), short-chain fructooligosaccharides (scFOS), galactooligosaccharides (GOS), mannan oligosaccharides (MOS), xylooligosaccharides (XOS)
FOS obtained by enzymatic hydrolysis of inulin is a fructan with a DP 2-20 (Hoseinifar et al., Reference Hoseinifar, Soleimani and Ringø2014). It is commonly found in foods such as artichoke, asparagus, and garlic. Beneficial effects of dietary FOS supplementation on growth performance, survival, gut microbiota, digestive enzyme activity, hematoimmunological parameters, and stress resistance have been reported in several fish species (Abid et al., Reference Abid, Davies, Waines, Emery, Castex, Gioacchini, Carnevali, Bickerdike, Romero and Merrifield2013; Zhang et al., Reference Zhang, Li, Xu, Jiang, Lu, Wang and Liu2013; Hoseinifar et al., Reference Hoseinifar, Soleimani and Ringø2014; Paz et al., Reference Paz, Mota da Silva, Marques da Silva and Val2019). In Caspian roach (Rutilus rutilus), gut autochthonous LAB was increased in fish fed 1 and 2% GOS (Hoseinifar et al., Reference Hoseinifar, Khalili, Rostami and Esteban2013). Whilst in a comparative supplementation study, scFOS and XOS altered European sea bass Dicentrarchus labrax gut microbial community in terms of increased number of OTUs and Margalef species richness index with no effect of GOS supplementation in sea bass diet (Guerreiro et al., Reference Guerreiro, Serra, Oliva-Teles and Enes2018). Prebiotic MOS can reduce the adverse impacts of feed distress on growth and reproductive performance in starved zebrafish. Contrary effects of poor feed on final weight (FW), length, SGR, FCR, levels of thyroxine, and LAB decreased with MOS supplementation; suggesting at the impact of MOS diet in amending negative effects of nutrient deprivation in zebrafish. Changes in sex steroids were affected by MOS supplementation, whereas no effect in the levels of testosterone and female 17β-estradiol in control or starved prebiotic treated fish was produced (Forsatkar et al., Reference Forsatkar, Ali, Rafiee, Farahmand and Lawrence2018).
Konjac oligosaccharide (KOS) and acidolysis-oxidized Konjac oligosaccharide (A-OKGM)
KOS is mainly an acid degradation or enzyme hydrolyzed product of konjac glucomannan (KGM); a water-soluble polysaccharide dietary fibre from Amorphophallus konjac tuber. A-OKGM is a low-molecular-weight (9.8 kDa) acidolysis-oxidized KGM that can be used as a feed additive owing to its small size compared to high viscosity KGM, size 500–2000 kDa. Both KOS and A-OKGM have been utilized as prebiotic in ya-fish (S. prenanti), an endemic freshwater cold fish of China. A-OKGM diets improved the growth performance of S. prenanti, increasing body lipid content by upregulating lipogenic genes expression, causing increased activity of lipogenic enzyme glucose-6-phosphate dehydrogenase (G6PD), while it contrarily depressed the activity of lipolytic lipase enzyme through downregulation of the mRNA levels of the lipolytic genes. Changes in lipogenic gene expression and enzymes activities were believed to be related to endogenous SCFAs production by fish gut bacteria (Chen et al., Reference Chen, Wu, Yan, Lv, He, Feng, Zhao and Duan2018b). Study with KOS-supplemented diets (Chen et al., Reference Chen, Wu, Yan, Zhao, Feng, He and Lv2019b) showed improved gut LAB, LA levels, total superoxide dismutase (SOD), serum ACH50, complement 3, nitric oxide content, and a significant decrease in stress indicators malondialdehyde as well as Aeromonas populations at all KOS inclusion levels. Immune-related gene expressions of toll-like receptor TLR-22, IL-1β, tumor necrosis factor TNF-α, were upregulated in fish with KOS supplementations.
Arabinoxylan oligosaccharide (AXOS), raffinose family polysaccharide, xylans
AXOS are breakdown products of arabinoxylans (AX) found in the cell wall of various cereal grains. AXOS consists of O-2 and/or O-3-L-arabinofuranosyl units linked to the main chain of β-1,4-linked D-xylopyranosyl units. AXOS preparations are made by enzymatic hydrolysis of the AX extracts using AX-degrading enzymes. AXOS compounds are represented by the average degree of polymerization (DPx) and arabinose to xylose ratio (DSy) symbolized in their denomination as AXOS-x-y. AXOS can significantly impact growth performance and gut microbiome profile of juvenile Siberian sturgeon A. baerii in a dose-dependent manner with beneficial shifts in gut microflora, mainly Firmicutes and higher SCFAs at 2% AXOS-32-0.30 levels (Geraylou et al., Reference Geraylou, Souffreau, Rurangwa, Meester, Courtin, Delcour, Buyse and Ollevier2013a). Prebiotic potential of AXOS is found to be strongly related to its average degree of polymerization. Health-promoting effects of AXOS are rendered in fish due to increased relative abundances of LAB and Clostridium, thus supporting the potential application of this prebiotic in sturgeon nutrition and disease prevention.
Prebiotic applications of mushroom-based and herbal/medicinal polysaccharides of aloe vera Astragalus polysaccharides (APS) has been reported in common carp, African catfish Clarias gariepinus, and largemouth bass Micropterus salmoides (Zou et al., Reference Zou, Hoseinifar, Miandare and Hajimoradloo2016; Lin et al., Reference Lin, Jiang, Chen, Luo, Doolgindachbaporn and Yuangsoi2017; Gabriel et al., Reference Gabriel, Wilhelm, Habte-Tsion, Chimwamurombe, Omoregie, Iipinge and Shimooshili2019). Recently, immune responses to purified lignin and hemicellulose in the Atlantic salmon (Yossa et al., Reference Yossa, Levesque, Groman and Lora2018) and proliferative effect of Lycium barbarum polysaccharide LBP (Zhou et al., Reference Zhou, Jiang, Wang, Zhang and Zhao2018) on probiotic Bifidobacterium longum infantis and Lactobacillus acidophilus NCFM have been explored for their potential as prebiotic candidates. Some other plant-derived prebiotic oligosaccharides used in finfish aquaculture are from the raffinose family (RF), raffinose and stachyose, obtained from grain legumes belonging to the family Fabaceae. A recent work (Karimi et al., Reference Karimi, Paknejad, Hoseinifar, Shabani and Mozanzadeh2020) evaluated the impacts of raffinose at increasing levels 1.0, 2.0, 4.0 g kg−1 in the diet of common carp C. carpio. Results with RF incorporation in feed reflected on improved skin mucus immune parameters, total Ig, skin mucus LA, humoral responses, serum LA, and alternative complement (ACH50) related to upregulation of intestinal immunological gene expression of interleukin IL-1α at 1 and 2 g RF per kg feed level in carp. In addition, RF inclusion altered mucus protein levels improving the intensity and generation of protein bands in SDS-PAGE analysis. Observed enhancement in innate responses may be relatable to immunostimulatory effects of RF as a consequence of microbiome modulation in the GIT of fish. Promising results have been obtained regarding functional constancy of mucosal barrier as well as intestinal microbiota in juvenile turbot S. maximus using dietary inclusion of stachyose at 1.25 and 5% levels (Yang et al., Reference Yang, Hu, Liu, Li, Ai, Xu, Zhang, Zhang, Zhang and Mai2018). Prebiotic effects of stachyose were seen on intestinal cellulose-degrading bacteria and gene expressions of barrier-forming tight junction proteins occludin, claudin-3, mucin-2 secretion, ZO-1, and claudin-like in the intestine. However, at a high inclusion level of 5% stachyose, increased abundance of pathogenic bacteria along with increased endogenous bacteria was of further concern. Similar results in juvenile turbot were confirmed based on the use of 1.25% dietary xylan (Yang et al., Reference Yang, Hu, Li, Ai, Zhang, Zhang and Mai2019). Xylans conferred positive effects on the regulation of the immune function, gene expression of tight junction proteins indicative of enhanced enteric mucosal function, and modulated microbiota in S. maximus. As found with high stachyose inclusions, dietary xylan (5%) exerted an untoward impact on intestinal well-being disrupting cellular tight junctions, increasing the gene expression of Muc-2 and pro-inflammatory cytokine IL-1β along with altering abundances of Clostridium, Escherichia coli, and Prevotella copri associated with intestinal debilitation. This establishes dose as an important limiting component in prebiotic use, as has been supported in studies using various prebiotics and above-listed evaluations.
β-glucans
β-Glucans are naturally existing prebiotic polysaccharides. β-Glucan is a glucose polymer with the backbone of β-(1,3); (1,4) or (1,6) linked β-D-glucopyranosyl units with variable length side chains of β-(1,4) or β-(1,6) linkages. These are present in bacteria, algae, fungi, and plants as structural components of cell walls or energy storage compounds (Akramienė et al., Reference Akramienė, Kondrotas, Didžiapetrienė and Kėvelaitis2007). Immune effects of prebiotic β-glucan are dependent on its molecular weight as well as the degree of branching. Structural differences can affect the extraction of β-glucans and in turn the immunostimulatory activity. Even β-glucans from the same species and sources can behave differently biologically, depending on extraction and purification processes used (Pilarski et al., Reference Pilarski, Ferreira de Oliveira, Darpossolo de Souza and Zanuzzo2017). In said work, two biotechnological forms of β-glucan, BG01 and BG02, were evaluated in the diet of Nile tilapia (Meng et al., Reference Meng, Wang, Wan, Xu and Wang2017), earlier to bacterial infection with Streptococcus agalactiae. The results exhibited varied biological behaviors of both forms of β-glucan, with BG01 causing immunostimulation while BG02 improved growth performance. Both forms increased the resistance against bacterial infection. Yet, another work demonstrated the effects of orally administered insoluble yeast β-glucan, microalgal polysaccharide-enriched extracts (MAe), and whole Phaeodactylum tricornutum cells to Senegalese sole (Solea senegalensis). Yeast β-glucans acted locally in the gut, modulating IL-1β; followed by a significant decrease in the abundance of intestinal Vibrio; while MAe effected transient systemic anti-inflammatory response with a less noticeable reduction in Vibrio load (Carballo et al., Reference Carballo, Pinto, Mateus, Berbel, Guerreiro, Martinez-Blanch, Codoñer, Mantecon, Power and Manchado2019). Whole microalgae delayed the activation of IL-1β and largely influenced intestinal microbial diversity probably because of their nutrient content. In aquaculture, particulate β-glucans are known for their enhancement effect on both non-specific and specific immune parameters regardless of the administration route (Vetvicka et al., Reference Vetvicka, Vannucci and Sima2013; Carballo et al., Reference Carballo, Pinto, Mateus, Berbel, Guerreiro, Martinez-Blanch, Codoñer, Mantecon, Power and Manchado2019).
Macroalga-based oligosaccharides: seaweed sodium alginate, alginate oligosaccharide (AlgOS)
Macroalga-based oligosaccharides are prospective prebiotics. Seaweed-derived low-molecular-weight sodium alginate (LWSA) is found to upregulate growth performance, innate immunity, and resistance to disease in tilapia O. niloticus (Van Doan et al., Reference Van Doan, Tapingkae, Moonmanee and Seepai2016). In a 60-day feeding trial, an increasing amount of LWSA 10, 20, 30 g kg−1 was administered to tilapia with 18 days challenge test against pathogenic S. agalactiae. The highest response values of complement, lysozyme (LZ), phagocytosis, RBA were observed at 10 g kg−1 LMWSA. Sodium alginate treatment also significantly improved the survival rate of O. niloticus although no significant difference in survival rate among supplemented diets was observed. Brown alga, Laminaria species-based AlgOS has been studied for its effect on the distal intestine microbiota of S. salar. AlgOS is an oligoalginate preparation of commercial purpose sodium alginate, Satialgine (France). High-throughput 16S rRNA gene amplicon sequencing technique was employed to investigate microbiota habitating intestine and mucus of the fish fed at 0.5 and 2.5% AlgOS levels (Gupta et al., Reference Gupta, Lokesh, Abdelhafiz, Siriyappagouder, Pierre, Sørensen, Fernandes and Kiron2019). At 0.5% AlgOS feed level, intestine and mucus of S. salar supported the dominance of core microbiota Proteobacteria, Aquabacterium parvum, Achromobacter insolitus, Photobacterium phosphoreum, Spirochaetes, Brevinema andersonii and Actinobacteria, Microbacterium ginsengiterrae. Among the following bacteria, certain genera Aliivibrio logei, A. parvum, B. andersonii, A. insolitus provide salmon with advantageous genes effective in carbohydrate degradation and SCFA butyrate production. Results substantiate that the low supplementation level of AlgOS in fish feed can plausibly induce a prebiotic effect on the distal intestinal microbiota of Atlantic salmon.
In aquaculture practices, certain prebiotics, as in the case of inulin, at high concentration are found to negate growth and gut health parameters, deteriorating microbial diversity and abundance, posing an appalling threat of disease incidences (Reza et al., Reference Reza, Abdolmajid, Abbas and Abdolmohammad2009; Nayak, Reference Nayak2010). Alarmingly, various pathogenic strains and opportunistic bacteria can co-feed on certain prebiotic carbohydrates and their degradation products, inflicting health hazards by proliferating inside the fish gut (Gatesoupe, Reference Gatesoupe2005). In this respect, dose-dependent and pathogenicity-related effects of prebiotic need to be thoroughly evaluated.
Synbiotics in finfish aquaculture: future developments
Synbiotics are functional additives containing both a prebiotic and a probiotic that work cooperatively to benefit the host by improving the survival and implantation of live microbial dietary supplement in the host GIT (Gibson and Roberfroid, Reference Gibson and Roberfroid1995; Andersson et al., Reference Andersson, Asp, Bruce, Ross, Wadström and Wold2001). By functional aspect, a synbiotic is an effective amalgamation of its component pre- and probiotic known to stimulate the growth of both probiotic and endogenous microbiota in the gut, enhancing growth performance, hematological parameters, oxidative-status, immunological parameters as well as immune-related gene expression in aquaculture fish species. In conformity, the prebiotic counterpart is able to prepare the environment for probiotic establishment in the intestine either through modulation of indigenous microflora or by specifically providing a competitive edge to the probiotic upon its own successful fermentation by the probiotic. Since the inception of the concept, with the first work on synbiotics in fish published in 2009 (Rodriguez-Estrada et al., Reference Rodriguez-Estrada, Satoh, Haga, Fushimi and Sweetman2009), there have been continuous developments in the aquaculture use of synbiotics (Ringø et al., Reference Ringø, Dimitroglou, Hoseinifar, Davies, Merrifield and Ringø2014). Synbiotic studies have recently gained centerstage of feed supplementation strategies since it bears combined merits of both pre- and probiotic in a single feed additive. Moreover, a synbiotic complements and/synergizes its component's effects with the scope of adding novel enhancing properties of its own as a whole (Kolida and Gibson, Reference Kolida and Gibson2011); for detail refer Fig. 1.
Mechanism of action: advantage over single pro- and prebiotics; choosing synbiotic combination
Because a synbiotic is a combination of defined prebiotic and probiotic, its mechanism of action is understood to be the contribution of both counterparts. The consonance of probiotic and prebiotic in a synbiotic can either be complementary or synergistic (Kolida and Gibson, Reference Kolida and Gibson2011). When both probiotic and prebiotic act independently of each other in influencing the gut microbiota and support host health, their main role is of complementing the intestinal microflora and they may support each other incidentally. Hence, they are complementary in terms of health benefits to host but may or may not potentiate each other; while in a synergistic combination, a prebiotic is primarily based on the aim to potentiate successful establishment and survival of probiotic in the gut where its direct effect on host microbiota, if present, is secondary. For detailed selection criteria and mechanism of action of synbiotics, see Fig. 1. In its entirety, synbiotic synergism fulfils the criteria of an ideal synbiotic scenario in which the prebiotic discreetly supports the propagation of the probiotic component with their beneficial effects being mutual as well as additive (Krumbeck et al., Reference Krumbeck, Walter and Hutkins2018). As admixture of prebiotics with probiotics can markedly benefit their host, in effective combination, this can allow the alteration of the gut environment by a prebiotic that will endow preferential growth conditions for the beneficial probionts. Merits of the following approach have been extended to the aquaculture scenario, with growing applications on the use of synbiotics as functional supplements in fish diet. Recent applications of synbiotics used in finfish aquaculture are listed in Table 3.
Table 3. Recent applications of synbiotics use in finfish aquaculture
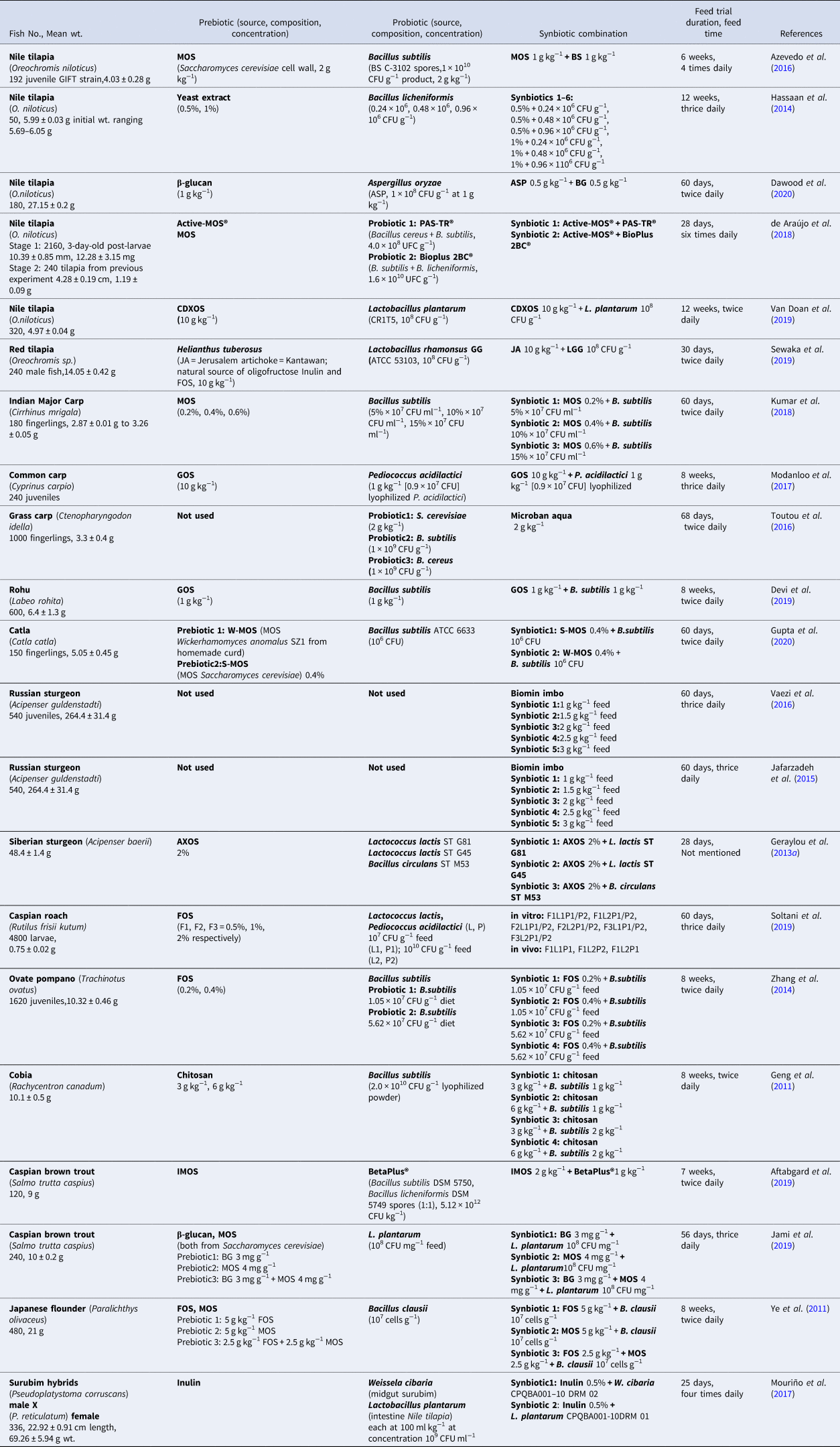
Effects of synbiotics are found to be dependent on dose as well as the dose duration. Time and dose-dependent effects of synbiotics toward changes in the gut microflora of Russian sturgeon (Acipenser guldenstadti) were investigated in a 60-day trial using synbiotic, Biomin imbo (Vaezi et al., Reference Vaezi, Khara and Shenavar2016). The experimental group fish were given a diet containing 1, 1.5, 2, 2.5, and 3 g synbiotic per kg diet, whereas control fish were provided basal diet without synbiotic supplementation. Bacterial counts were conducted at experimental days 20, 40, and 60. At sampling times of 20, 40, 60 days, the highest LAB count was obtained in 3 g synbiotic per kg diet fed fish. The Highest total bacterial counts (TBC) were observed in control and 1 g kg−1 treatment group at days 40 and 60, respectively.
It is suggestive that synbiotic dosage and duration of treatment has a pronounced impact on the colonization of balanced gut microbiota. A similar work evaluating hematological and immunological parameters with synbiotic Biomin imbo was conducted on A. guldenstadti by another group (Jafarzadeh et al., Reference Jafarzadeh, Khara and Ahmadnezhad2015). Following a similar experimental design (1, 1.5, 2, 2.5, and 3 g synbiotic per kg diet for 60 days), synbiotic effects were determined to augment neutrophils, monocytes, and eosinophil counts, LA, and IgM while no considerable effects of synbiotic supplement on Hb, Ht, mean corpuscular volume (MCV), and MCHC values were observed when compared to the control group. In all, synbiotic treatment in sturgeon was beneficial for immune components except leucocyte count, without any significant impact on hematological parameters. Most of the available studies on synbiotics are based on in vivo evaluations, while information on in vitro selection of synbiotics is limited (Rurangwa et al., Reference Rurangwa, Laranja, Van Houdt, Delaedt, Geraylou, Van de Wiele, Van Loo, Van Craeyveld, Courtin, Delcour and Ollevier2009). To this effect, informative work was provided on feeding, growth, and physiological response as well as enteric microbiota in rainbow trout (Hoseinifar et al., Reference Hoseinifar, Mirvaghefi, Amoozegar, Merrifield and Ringø2017). In fingerlings of O. mykiss, optimum synbiotic combination was selected in vitro between probiotic P. acidilactici and prebiotics inulin, GOS, FOS, XOS, and IMOS, based on growth and SCFA output. Best suited in vitro growth outcomes under aerobic as well as anoxic conditions were observed with the combination of P. acidilactici and GOS (1%) producing butyrate as major SCFA. On the basis of the outcomes of in vitro studies, P. acidilactici and GOS were selected for studying their in vivo synbiotic effects. Synbiotic supplementation significantly improved growth parameters such as SGR, FCR, and live WG whereas hematological parameters did not show any significant difference between various treatments. Additionally, autochthonous LAB levels were plausibly improved, while total endogenous bacterial levels remained unaltered. These results ascertain P. acidilactici and GOS as a considerably effective synbiotic combination for trout.
The above work classically exemplifies the synergistic synbiotic approach for the deliverance of synbiotic functionalities in finish aquafeeds. More such studies are warranted for establishing synbiotic concept in the developing scenario of aquaculture feed supplementations.
Microencapsulation of synbiotics: ease of safe delivery
As understood in case of probiotics, microencapsulation of synbiotic feeds expedite means for their safe and viable delivery in the host. In finfish aquaculture, microencapsulation of synbiotic feed supplement follows two different stratagems, one in which encapsulated probiotic is combined to prebiotic for administration to fish species, another where both probiotic as well as prebiotic are combinedly microencapsulated for disbursement. In an example to the first kind of encapsulation approach, probiotic Bacillus sp. NP5 was microencapsulated and combined with MOS as a prebiotic for evaluation of A. hydrophila incidence in Pangasianodon hypophthalmus (Tamamdusturi et al., Reference Tamamdusturi, Widanarni and Yuhana2016). Microencapsulation of tilapia-derived probiotic Bacillus NP5 was carried out in maltodextrin solution as the coating material using spray drying encapsulation method. Encapsulated synbiotic was evaluated in comparison to individual probiotic Bacillus sp. NP5 (1%), and prebiotic MOS (0.2%) ministrations. Highest SGR and lowest FCR were obtained with encapsulated synbiotic. TBC, RBC count, and Hb level of synbiotic groups were best among all treatments, while leukocyte count, PA, and RBA in all treatments during the feeding trial did not show any significantly different effect (P > 0.05) to controls. PA at the end of post-challenge by A. hydrophila showed higher values for all functional treatments as compared to control.
A complete synbiotic microencapsulation approach was carried out in common carp (Djauhari et al., Reference Djauhari, Widanarni, Sukenda, Suprayudi and Zairin2017). Preparation of microencapsulated synbiotic was achieved through a three-step procedure involving preparation of coating materials, microencapsulation of synbiotic, and enumeration of probionts in microencapsulated synbiotic pre- and post-microencapsulation, as well as later post one-month storage. Using spray drying technique, whey protein and maltodextrin were coated to synbiotic in equal mixture. Microencapsulated synbiotic can provide improved growth and health parameters in carp with appreciable results for daily growth rate, FCR, PR, and fat retention along with hemato-immunological parameters at 10 g kg−1 synbiotic feed relative to other treatments.
The prowess of encapsulation has been described in a comparative evaluation study of encapsulated versus unencapsulated supplementation in fish diets (Madreseh et al., Reference Madreseh, Ghaisari and Hosseinzadeh2019). Probiotic Lactobacillus fermentum and prebiotic lactulose-composed functional diets were investigated for their impact on growth performance, prevention of heavy metal, and trace metal absorption-accumulation in trout tissues. Lactobacillus fermentum were encapsulated with sodium alginate supporting material using extrusion method. Five experimental diets were prepared as synbiotic lyophilized L. fermentum (107 CFU g−1 + lactulose at 10 g kg−1 feed), encapsulated synbiotic (L. fermentum 107 CFU g−1 + lactulose at 10 g kg−1), prebiotic (lactulose 10 g kg−1 feed), probiotic (lyophilized L. fermentum), and encapsulated probiotic L. fermentum (107 CFU g−1). Results of the study ascertained that encapsulated synbiotic feed supplementation led to improved growth performance and exclusion of heavy metal toxic accumulation from trout tissues.
Synbiotic studies in finfish aquaculture
Studies on the application of synbiotics in finfish aquaculture widely encompass the evaluation of zootechnical parameters such as growth parameters, feed utilization, digestive enzyme activities, survival, disease resistance, gut microbial alterations, stress physiology, hematoserological indices, oxidative status, immunological parameters, and immune-related gene expression. A comparative account of these studies is discussed here forth.
Zootechnical parameters: growth parameters, feed utilization; digestive enzymes, intestinal morphometry, gut microbiota; survival and disease resistance
Synbiotic treatment in tilapia
Synbiotic combination of yeast S. cerevisiae based prebiotic MOS 1 g and probiotic B. subtilis BS C-3102 strain 1 g was evaluated in comparison to 2 g kg−1 prebiotic and probiotic diet for growth, average daily gain (ADG), FCR, SGR, PER, carcass yield (CY), total, standard length, and body height (TL, SL, H) in Nile tilapia (Azevedo et al., Reference Azevedo, Filho, Pereira, Cardoso, Andrade Ricardo de and Vidal Júnior2016). Fishes treated pre-, pro-, and synbiotic diets achieved better ADG, FCR, SGR, and PER than populations on control diets (P < 0.05). All functional additives pre-, pro-, and synbiotic provided better CY, TL, SL, and H as compared to control diet (P < 0.05) but did not affect HSI, VSI, and DFI values (Table 3). Results of fish carcass composition reflect that moisture, crude energy, and mineral matter values were unaffected by functional diet treatments; while crude protein was increased and ether extract (EE) lowered with synbiotic treatment to fish. Differences in carcass protein and fat levels may be due in relation to effective synthesis and deposition rate in fish muscle; while changes in CP content with synbiotic diets may indicate increased amino acid absorption enhancing the digestible protein and energy of the diet, with resultantly declining carcass EE. Similar results for growth performance in tilapia were observed (Hassaan et al., Reference Hassaan, Soltan and Ghonemy2014), with the highest obtainable FI, final BW, BL, WG, FCR, PER, PPV, and SGR recorded with synbiotic level 0.48 × 106 CUF g−1 Bacillus licheniformis +1.0% yeast extract in comparison to control as well as other synbiotic levels. Corncob-derived xylooligosaccharides (CDXOS) and L. plantarum CR1T5 introduced into tilapia diet also improved FW, WG, SGR, and FCR; however, differences in the survival rate of fish fed formulated or control diets were insignificant (Van Doan et al., Reference Van Doan, Hoseinifar, Tapingkae, Seelaudom, Jaturasitha, Dawood, Wongmaneeprateep, Thu and Esteban2019). Higher RPS and resistance to S. agalactiae were produced with synbiotic CDXOS (10 g kg−1) + 108 CFU g−1 L. plantarum than prebiotic or probiotic groups in fish diet. A significant elevation in villi length, and efficiency of growth and feed (FBW, WG, SGR, FER, and PER) was observable on supplementation of Aspergillus oryzae and β-glucan synbiotic over the other dietary regimes in tilapia (Dawood et al., Reference Dawood, Mohamed, Moustafa and Mohamed2020). The effect of synbiotic composition comprising commercial prebiotic Active-MOS® with probiotics PAS-TR® (Bacillus cereus + B. subtilis) and Bioplus 2BC® (B. subtilis + B. licheniformis), respectively, was evaluated in post-larvae (PL) tilapia for growth gains, carcass analysis, bacterial recoveries, and intestinal histomorphometry during the sex interchange phase (de Araújo et al., Reference de Araújo, Barbas, Ishikawa, Dias, Sussel, Marques and Tachibana2018). In the work, supplementation of PL diet had no contribution in significant differences of growth, survival, microbiological, or histological parameters. Nonetheless, advantages on the use of the additives were observed post experimental infection with A. hydrophila with higher relative protection level (38.10%) and RPS in fish administered synbiotic Active-MOS® and Bioplus 2BC®. Thus synbiotic (Active-MOS® + Bioplus 2BC®) was recommended for O. niloticus PL farming during incidences of bacterial disease outbreaks during the sex interchange episodes. Use of plant-based prebiotic from Jerusalem artichoke tuber admixed to Lactobacillus rhamnosus GG in synbiotic formulation was investigated for red tilapia, Oreochromis spp. (Sewaka et al., Reference Sewaka, Trullas, Chotik, Rodkhum, Chansue, Boonanuntanasarn and Pirarat2019). Synbiotic treatments revealed significantly elevated SGR, ADG, FCR, glucose, TP, CHO levels, and low cumulative mortalities toward A. veronii challenge as compared to control. Marked increase in absorptive area, proximal and distal intestine goblet cell number was also obtained with synbiotic-treated diet than with individual prebiotic, probiotic, or control diet.
Synbiotic treatment in trout
A work explored the effect of β-glucan 3 mg g−1, MOS 4 mg g−1, and L. plantarum 108 CFU mg−1 diet on SGR, CF, FCR, feed intake, PER, DGI, WG, survival, and intestinal microbial of Caspian trout, Salmo trutta caspius (Jami et al., Reference Jami, Kenari, Paknejad and Mohseni2019). Eight treatment groups of individual prebiotic or probiotic were drawn with basal diet as β-glucan, MOS, L. plantarum, MOS + β-glucan, respectively; and synbiotic combinations (β-glucan + L. plantarum; MOS + L. plantarum; MOS + β-glucan + L. plantarum), respectively. Synbiotic along with all other supplemented diets increased WG, PER, and FW, and reduced FI and FCR. Prebiotic MOS + β-glucan treated fish group displayed the highest cortisol and glucose levels. Total lipid increased in fish fed MOS and MOS + β-glucan while CF was lowest in MOS + β-glucan + L. plantarum synbiotic group.
Synbiotic diet of combined commercial probiotic BetaPlus® at 1 g kg−1 and prebiotic isomaltooligosaccharides (IMOS) 1 g kg−1 was evaluated for effects in Caspian brown trout (Aftabgard et al., Reference Aftabgard, Salarzadeh, Mohseni, Shabanipour and Zorriehzahra2019). Intestinal bacterial density was high for TVABC and LAB in the synbiotic diet fed trout. Significant improvement with synbiotic supplementation was obtained for BW, FCR, and survival rate (SUR) compared to control fed fish.
Synbiotic treatment in carp
Growth performance and disease resistance of C. carpio (Mohammadian et al., Reference Mohammadian, Nasirpour, Tabandeh and Mesbah2019) was assessed in a 60-day feed trial to ascertain the impact of β-glucan, MOS, and Lactobacillus casei. Three synbiotic treatments including increasing concentration of β-glucan (0.5, 1, 1.5%) with MOS and L. casei 5 × 107 CFU kg−1 diet were compared to probiotic L. casei in diet. Growth and feed usage were improved with synbiotic containing 1% β-glucan; lowering mortality rate with A. hydrophila following a challenge test. Intestinal enzyme activities of ALP, lipase, amylase, trypsin, and protease significantly enhanced within the treatment regime compared to control. The results suggest that these treatments, especially synbiotic at 1% β-glucan level, can improve C. carpio health parameters.
Hematoserological and immunological indices; immune-related gene expression
Synbiotic B. licheniformis (0.48 × 106 CFU g−1) with 0.5% yeast extract in O. niloticus (Meng et al., Reference Meng, Wang, Wan, Xu and Wang2017) diet gave the greatest hematoloserological values of RBCs, WBCs, and Hb as compared to prebiotic, probiotic, and control diets (Hassaan et al., Reference Hassaan, Soltan and Ghonemy2014). Synbiotic supplementation at 0.96 × 106 CFU g−1 Bacillus + 0.5% yeast extract rendered lowest ALT levels (82.50 l l−1) than high value of 89.50 l l in control. All levels of synbiotics significantly decreased the serum concentrations of ALT and AST. Low ALT and AST levels indicated positive protecting effects of probiotic and yeast extract on hepatic cells. Fungal probiotic, A. oryzae and β-glucan-based synbiotic supplementation (Dawood et al., Reference Dawood, Mohamed, Moustafa and Mohamed2020) enhanced RBA, IgM, LA, bactericidal, and phagocytotic activity indicating improved innate immunity in tilapia by synbiotic additives. Modulated blood Hb, Ht, RBC, WBC, TP, and digestive enzymes were reported in formulated diets with their prominence in the synbiotic group. Moreover, syn- or probiotic diet additionally decreased blood TG levels. Immune response (LA, ACH50, IgM, IL-1, IL-8, and TNF-α) assessed in Caspian trout (Jami et al., Reference Jami, Kenari, Paknejad and Mohseni2019) showed all formulated pre-, pro-, and synbiotic diets enhanced LA, IgM, and ACH50; with greatest concentrations observed in synbiotic (MOS + β-glucan + L. plantarum and β-glucan + L. plantarum) and prebiotic (MOS + β-glucan) fed groups. MOS diet induced the upregulation of TNF-α1 in the head kidney while β-glucan specifically upregulated IL-1β levels. Combined supplementations of both improved the humoral immune system of trout. High WBCs, monocytes and neutrophil levels, were obtained for IMOS 1 g kg−1 based synbiotic in the diet of Caspian brown trout (Aftabgard et al., Reference Aftabgard, Salarzadeh, Mohseni, Shabanipour and Zorriehzahra2019), while the RBCs, Hb, Ht, MCV, and lymphocytes were markedly lower (P < 0.05) in the control group. According to the authors, decreased erythropoiesis in trout fed synbiotic was because of slow iron release from the reticuloendothelial system due to increased competition by synbiotic probiont for preferential absorption of iron to promote endogenous bacteria. Also, a decrease in Hb toward synbiotic treatment might be because of decreased energy and oxygen demands in low-stress condition; based on the nutritional advantage of biotic additives. An increase in serum TG, CHO, TP, albumin, A/G ratio, and IgM concentrations, as well as ALT and lactate dehydrogenase LDH activities was found in synbiotic-treated group. Early manifestation of beneficial synbiotic effects was observed in L. rohita fed on GOS + B. subtilis diet in an 8-week treatment trial. GOS + B. subtilis 1:1 administration significantly elevated WBC, GB, TP levels, serum PA, RBA, IgM, complement C3 level, alternative complement pathway, and LA in head kidney leucocytes; comparatively earlier than prebiotic and probiotic treatments in L. rohita (Devi et al., Reference Devi, Harikrishnan, Paray, Al-Sadoon, Hoseinifar and Balasundaram2019). Inducible nitric oxide synthase (iNOS) and NF-κB are crucial immunomodulators during fish defense against numerous pathogens. Synbiotic diet contributed to upregulation of iNOS and NF-κB gene transcriptions as well as pro-inflammatory cytokines IL-1β, IL-8, and TNF-α, while anti-inflammatory cytokines (IL-10 and TGF-β gene expressions) were significantly downregulated in synbiotic as well as other diet regimes. Early onset of disease combats against A. hydrophila on 4th week was achieved with synbiotic supplementation as compared with individually fed probiotic or prebiotic diets.
Oxidative status; anti-oxidant enzymes
Synbiotic addition effectively enhanced anti-oxidative enzymes SOD, catalase (CAT), and glutathione peroxidase (GPX) fed O. niloticus; during a 60-day trial (Dawood et al., Reference Dawood, Mohamed, Moustafa and Mohamed2020). Synbiotic or probiotic treatment was counterproductive on oxidative enzyme malonaldehyde, suggesting an improved antioxidant response as well as minimized cell damage in treated fish when compared to control fed populations. Early development of prolonged beneficial anti-oxidative effects of synbiotic GOS and B. subtilis was evident in L. rohita (Devi et al., Reference Devi, Harikrishnan, Paray, Al-Sadoon, Hoseinifar and Balasundaram2019). SOD, CAT, and GPX activity peaked from 4 to 8 weeks with synbiotic treatment while these peaks were achieved late during the 6th and 8th weeks in the prebiotic group. Concurrent feeding on bio-additives can provide the ability to mitigate environmental stressors such as alteration in pH conditions in aquatic environment. In this respect, a study (Singh et al., Reference Singh, Tiwari, Chadha, Munilkumar, Nilesh and Pawar2019) investigated the effectiveness of synbiotic diet using Bacillus circulans strain PB7 (106 cfu g−1) and FOS 1%; for L. rohita juveniles reared under acidic, normal pH conditions. Two treatment groups of fishes; one exposed to low pH (5.5) and other to normal pH (7.0) was fed with experimental (pre, pro, and synbiotic) and control diets and examined for their effect on growth performance and immune response of fish. Low pH-control fed group displayed stunted growth and impaired immunophysiological function while subsequent feeding on formulated diet remarkably improved WG, FCR, SGR, PER, and immunophysiological function (hematoserological indices, LA, RBA) of rohu juveniles exposed to similar low pH conditions. It was ascertained that the use of synbiotics in finfish aquaculture provided added advantages over their individual prebiotic and probiotic counterparts; yet, deviations in observations were likely due to choice of prebiont, probiont, diet dosage, fish age/size/species, virulence as well as methods of pathogenic challenge, water quality parameters, feeding management and duration. All these parameters need to be thoroughly evaluated per se while conducting synbiotic studies toward investigating their effectiveness.
Concluding remarks
Fish health depends on the diversity and functionality of its beneficial microbiome; specifically, the healthy GIT microbiota. Promising dietary approaches currently available to modulate the fish gut microbiota involve fermentable ‘prebiotics’, live microbial ‘probiotics’ bio-additives, and potentially their synergistic amalgamation as ‘synbiotics’. Future trends intend at developing strategies for their effective dispersal, growing use of microencapsulation for safe, targeted, prolonged delivery; thereby to attain better effects in terms of growth, survival, improved gut microflora, immunological parameters, disease resistance, stress mitigation, and overall well-being of aquaculture finfish species. Upcoming trends in the use of microbial supplements also show shifting reliance on synbiotic usage deriving additional and novel advantages to their probiotic and prebiotic counterparts. Synbiotics as feed supplements provide a possibility for safe, sustainable, as well as robust fish nutrition; and are viewed as prospective strategic biotherapeutics, curbing the need for hazardous chemical stimulants or antibiotic-type growth promoters. Growing applications based on synbiotics reflect such forward trends for better delivery, improved survival in terms of microencapsulation, in vitro and in vivo selection-evaluations for beneficial outcomes as well importantly consolidating individual advancements in prebiotic and probiotic arena for synbiotic developments.
Acknowledgements
The authors are thankful to their institutions Delhi Technological University and Sri Aurobindo College, University of Delhi for their kind and ardent support throughout the development and formalization of the work.