INTRODUCTION
Radiocarbon (14C) marine reservoir effects (MRE) have been determined from Holocene coastal sediments in the East Asia region (Yu et al. Reference Yu, Hua, Zhao, Hodge, Fink and Barbetti2010; Nakanishi et al. Reference Nakanishi, Hong, Sung and Lim2013, Reference Nakanishi, Hong, Sung, Sung and Nakashima2015, Reference Nakanishi, Hong, Shimoyama, Sato, Park and Lee2017a, Reference Nakanishi, Hong, Sung, Nakashima, Nahm, Lim and Katsuki2017b, Reference Nakanishi, Takemura, Matsuyama, Shimoyama, Hong and Okuno2017c; Nakamura et al. Reference Nakamura, Masuda, Miyake, Hakozaki, Kimura, Nishimoto and Hitoki2016; Yang et al. Reference Yang, Wang, Burr, Liu and Fan2018). The reservoir ages ranged from 60 to 1100 yr, scattered over the period 100–10,200 cal BP, which were significantly larger than the values from “prebomb” samples of known age, before AD 1955 in this area (Figure 1A; Konishi et al. Reference Konishi, Tanaka and Sakanoue1982; Hideshima et al. Reference Hideshima, Matsumoto, Abe and Kitagawa2001; Kuzmin et al. Reference Kuzmin, Burr and Jull2001, Reference Kuzmin, Burr, Gorbunov, Rakov and Razjigaeva2007; Southon et al. Reference Southon, Kashgarian, Fontugne, Metivier and Yim2002; Kong and Lee Reference Kong and Lee2005; Shishikura et al. Reference Shishikura, Echigo and Kaneda2007; Yoneda et al. Reference Yoneda, Uno, Shibata, Suzuki, Kumamoto, Yoshida, Sasaki, Suzuki and Kawahata2007; Hirabayashi et al. Reference Hirabayashi, Yokoyama, Suzuki, Miyairi and Aze2017). These results indicate that the information on MRE is indispensable for chronological control not only from prebomb samples but also from geological and archaeological samples. One such effect from the warm Kuroshio Current environment in southwestern Japan was determined at the Oita Plain within Seto Inland Sea (Nakanishi et al. Reference Nakanishi, Takemura, Matsuyama, Shimoyama, Hong and Okuno2017c). To identify the direct chronological changes of the MRE under the Kuroshio Current, 14C ages from pairs of marine mollusks and terrestrial plants were measured from the same stratigraphic horizons within a single core of Holocene sediments beneath the Sukumo Plain, in the southwestern portion of Shikoku Island. Subsequently, the results were compared with values from the Oita Plain, 310 ± 130 yr (n = 7), and Hakata Bay, 310 ± 150 yr (n = 33), on the northern coast of Kyushu (Nakanishi et al. Reference Nakanishi, Hong, Shimoyama, Sato, Park and Lee2017a, Reference Nakanishi, Takemura, Matsuyama, Shimoyama, Hong and Okuno2017c), as well as the Korean Peninsula (Nakanishi et al. Reference Nakanishi, Hong, Sung and Lim2013, Reference Nakanishi, Hong, Sung, Sung and Nakashima2015; 380 ± 190 yr [n = 48], Reference Nakanishi, Hong, Sung, Nakashima, Nahm, Lim and Katsuki2017b; 450 ± 230 yr [n = 9]), the South China Sea (Yu et al. Reference Yu, Hua, Zhao, Hodge, Fink and Barbetti2010), and Taiwan (Yang et al. Reference Yang, Wang, Burr, Liu and Fan2018).
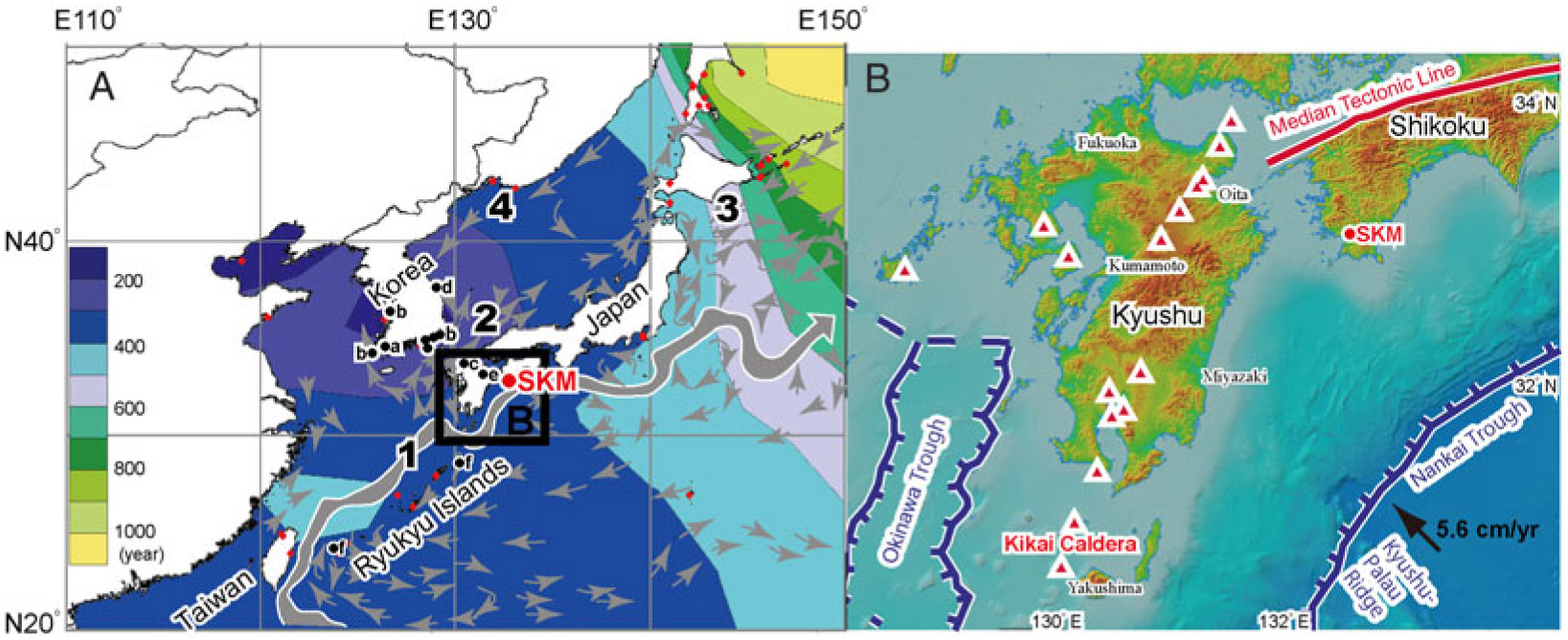
Figure 1 A: Study sites and modern marine reservoir ages in East Asia (modified after Nakanishi et al. Reference Nakanishi, Hong, Sung, Sung and Nakashima2015). Data from The Marine Reservoir Database. 1, Kuroshio Current; 2, Tsushima Current; 3, Oyashio Current; 4, Liman Current; a, YAR-4 from Nakanishi et al. (Reference Nakanishi, Hong, Sung and Lim2013); b, study sites from Nakanishi et al. (Reference Nakanishi, Hong, Sung, Sung and Nakashima2015); c, HKA and HIUB from Nakanishi et al. (Reference Nakanishi, Hong, Shimoyama, Sato, Park and Lee2017a); d, PMR-2 from Nakanishi et al. (Reference Nakanishi, Hong, Sung, Nakashima, Nahm, Lim and Katsuki2017b); e, KUO-1 from Nakanishi et al. (Reference Nakanishi, Takemura, Matsuyama, Shimoyama, Hong and Okuno2017c). f, study sites from Hirabayashi et al. (Reference Hirabayashi, Yokoyama, Suzuki, Miyairi and Aze2017). B: Tectonic map around the Kyushu area and drilling site of Sukumo (SKM) core. The base map is modeled after the Geospatial Information Authorityof Japan. Kikai Caldera is the source of the Kikai-Akahoya tephra (Machida and Arai Reference Machida and Arai2003).
STUDY SITE
The Sukumo Plain is situated in the southwestern portion of Shikoku Island, the smallest of the four major islands of Japan (Figure 1A). The plain is located on the subsidence area created by the convergence of the Philippine Sea Plate with the Eurasian Plate (Fitch and Scholz Reference Fitch and Scholz1971; Okamura Reference Okamura1990). The coastal water around the Sukumo Plain is influenced by the warm Kuroshio Current, which originates from the North Equatorial Current. This alluvial plain is formed by the Matsuda River. A melange matrix of Late Cretaceous to Oligocene accretionary complexes and middle to late Miocene felsic plutonic rocks form the bedrock of this catchment, without any carbonate rocks (Tanaka Reference Tanaka1980). The Sukumo (SKM) core was drilled in the northern part of the plain (33°55′55.7″N, 132°42′21.3″E; elevation +0.99 m) (Tsuji et al. Reference Tsuji, Nanayama, Yamaguchi, Ikeda, Nakanishi, Kondo, Maeno, Hongo and Kimura2018). This drilling site is on reclaimed land and approximately 700 m north from the mouth of the Matsuda River. The SKM core is 38.5 m in length and 8 cm in diameter. It was collected at 1.0 m intervals, using a double-walled tube sampler. The proportion of core recovery is almost 94%.
METHODS
Sedimentological and Paleontological Analyses
To infer the sedimentary environments of the SKM core, we described lithologies, colors, sedimentary structures, textures, contract characteristics, fossil components, and grain sizes. In addition, to improve the understanding of sedimentary environments, mollusk assemblages were identified on the basis of the classification of Okutani (Reference Okutani2017) and World Register of Marine Species (http://www.marinespecies.org/index.php). Ostracode taxa were identified, using Ishizaki (Reference Ishizaki1968), Yasuhara and Irizuki (Reference Yasuhara and Irizuki2001), and Irizuki et al. (Reference Irizuki, Seto and Nomura2008) as references.
Accelerator Mass Spectrometry 14C Dating
Thirty-two terrestrial macrofossils and 30 calcareous marine samples, in good condition, were collected from the core for accelerator mass spectrometry (AMS) 14C dating. The sampling depths and weights are shown in Figure 2. Fragile samples, such as twigs and thin bivalves, as well as articulated shells of dominant species were selected as often as possible because they are less likely to have been reworked. Twelve terrestrial macrofossils and 11 calcareous marine samples were washed repeatedly with an ultrasonic cleaner and then cleaned chemically using acid-base-acid (Brock et al. Reference Brock, Higham, Ditchfield and Bronk Ramsey2010) for terrestrial plants and an acid treatment for calcareous marine samples to remove secondary contaminants. The sampling depths are listed in Table 1.
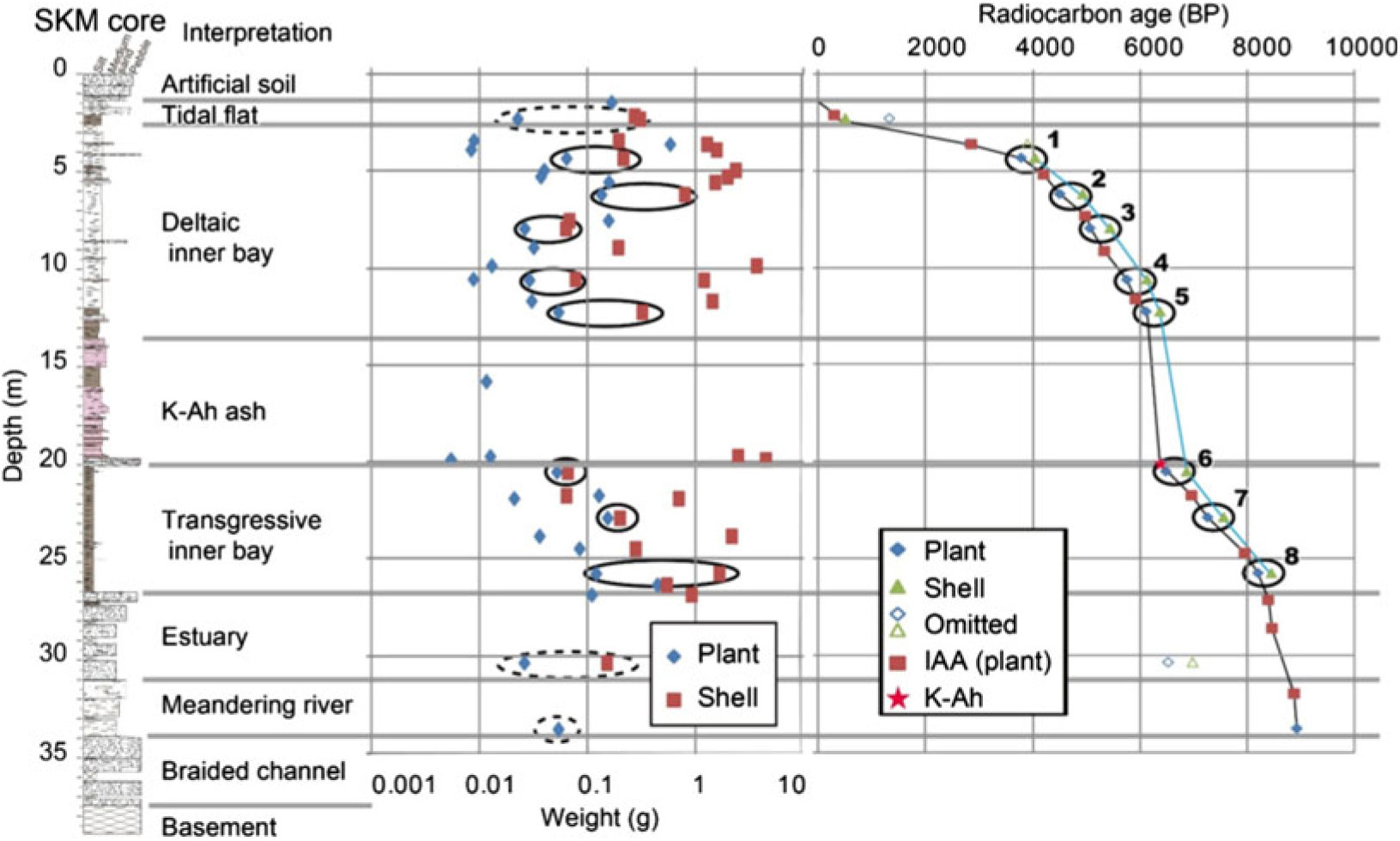
Figure 2 Sediment column of the SKM core with interpretation of paleoenvironments, weights of plant and shell fragments, and 14C ages. Ages of IAA were measured by the Institute of Accelerator Analysis (Tsuji et al. Reference Tsuji, Nanayama, Yamaguchi, Ikeda, Nakanishi, Kondo, Maeno, Hongo and Kimura2018). K-Ah indicates the Kikai-Akahoya tephra.
Table 1 Radiocarbon ages from the SKM core. Errors are 1σ ranges. Medium depths with an asterisk (*1–8) are those used to measure the marine reservoir age. Radiocarbon ages with a cross (+) and circle (o) were interpreted from reworked samples and dropped samples, respectively.
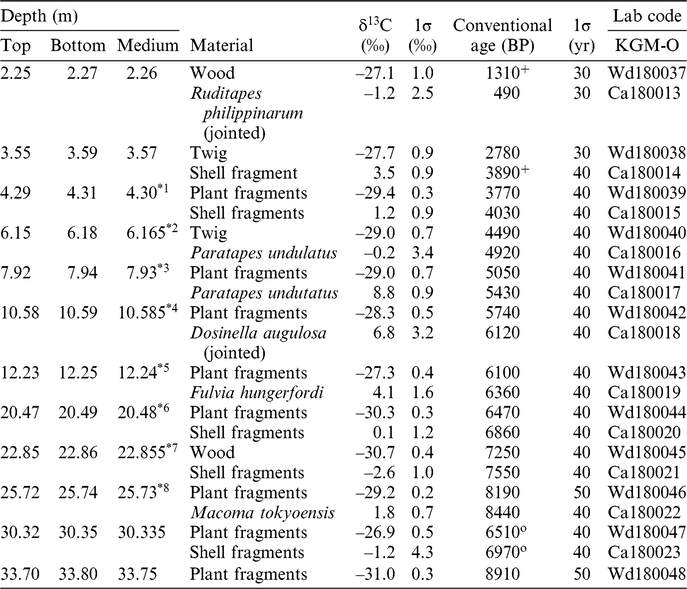
The 14C ages from 11 terrestrial plants (Tsuji et al. Reference Tsuji, Nanayama, Yamaguchi, Ikeda, Nakanishi, Kondo, Maeno, Hongo and Kimura2018) by the Institute of Accelerator Analysis Ltd. (IAA) provided an age model of the sedimentation in this site. Samples of 14C-free wood and the IAEA C-1 reference material were treated using the same procedure for control measurements. The carbonate samples were powdered using a mortar and pestle. The standards NIST OxII, IAEA C-7, and C-8 were combusted in an elemental analyzer, and the CO2 gas was purified cryogenically in a high-vacuum automatic preparation system (Hong et al. Reference Hong, Park, Kim, Woo, Kim, Choi and Kim2010a; Park et al. Reference Park, Hong, Woo, Choi, Kim and Kim2010) and then converted into graphite through the reduction of Fe powder with hydrogen gas in a quartz tube. The 14C ages of the samples were measured along with the standards at a Cockcroft-Walton type 1 MV AMS facility at the Korea Institute of Geosciences and Mineral Resources (KIGAM) (Hong et al. Reference Hong, Park, Sung, Woo, Kim, Choi and Kim2010b). The carbon isotopic fractionations were corrected by δ13C values, measured at the AMS facility. The 14C reservoir ages and the uncertainty were calculated by the paired samples of terrestrial macrofossils and calcareous marine samples from same horizons. The 14C ages and the uncertainty of the terrestrial samples were converted into calendar dates using IntCal13 (Reimer et al. Reference Reimer, Bard, Bayliss, Beck, Blackwell, Bronk Ramsey, Buck, Cheng, Edwards, Friedrich, Grootes, Guilderson, Haflidason, Hajdas, Hatté, Heaton, Hoffmann, Hogg, Hughen, Kaiser, Kromer, Manning, Niu, Reimer, Richards, Scott, Southon, Staff, Turney and van der Plicht2013) and CALIB 7.1 (Stuiver et al. Reference Stuiver, Reimer and Reimer2018).
RESULTS
Seven sedimentary facies were identified on the basis of our core analysis (a braided river channel, a meandering river, an estuary, a transgressive inner bay, Kikai-Akahoya (K-Ah) volcanic ash, a deltaic inner bay, a tidal flat) (Tsuji et al. Reference Tsuji, Nanayama, Yamaguchi, Ikeda, Nakanishi, Kondo, Maeno, Hongo and Kimura2018), as illustrated in Figure 2. The basement was a siltstone of the Oligocene Shimanto Group (Tanaka Reference Tanaka1980). These lithofacies are described below, along with remarks, depositional environments, and ages of the facies. The 14C ages are listed in Table 1, and an age/core depth diagram is provided in Figure 2.
Braided River Facies (core depths of 33.90–37.46 m)
Description
This sediment facies consisted of alternating beds of sandy gravel layers (10–40 cm thick) and gravel-supported silty sand layers (10–90 cm thick). The gravel clasts were subangular to subrounded, and the clast diameters were predominantly 5–90 mm. The matrix portion comprised a mixture of silt and medium to coarse sand. No samples for 14C dating were found in this sediment.
Interpretation
We interpreted this sediment as a braided river channel sediment because such alternating beds of sandy gravel and gravel-supported sand were commonly reported in bedload environments (Miall Reference Miall1977, Reference Miall, Walker and James1992).
Meandering River Facies (core depths of 31.00–33.90 m)
Description
This sediment consisted of a lower, gravelly sand bed and an upper gravel bed. The matrix portion was mainly composed of cross-laminated medium to coarse sand. Plant remains were found throughout this facies. The 14C age of plant fragments from 33.75 m depth was 8910 ± 50 BP (9795–10,203 cal BP, 2σ ranges). No calcareous samples for 14C dating were found in this sediment.
Interpretation
The terrestrial plant remains and lack of marine mollusks indicated that this facies formed in a freshwater environment. The difference in grain size implies that the flow velocity was stronger toward the upper portion, associated with a migration of the river channel.
Estuary Facies (core depths of 27.25–31.00 m)
Description
This sediment consisted of a lower, gravelly sand bed and upper alternating beds of sandy gravel and mud. The matrix portion mainly consisted of cross-laminated medium- to coarse-grained sand. Plant remains and shell fragments were scattered within this sediment. The 14C ages of plant and shell samples from 30.335 m depth were 6510 ± 40 BP (7323–7492 cal BP, 2σ ranges) and 6970 ± 40 BP, respectively.
Interpretation
The combination of terrestrial plant remains and marine mollusks indicates that this facies formed in an environment with freshwater from a terrestrial source and saline water from a marine source, such as an estuary. The increase in grain size with decreasing depth indicates a stronger flow velocity toward the upper portion, associated with a change in sea level and/or transportation of sediments. The absence of rootlets in this sediment implies that it was likely deposited in an area deeper than the intertidal zone.
Transgressive Inner Bay Facies (core depths of 20.07–27.25 m)
Description
This facies consisted of fine, soft mud with plants, shells, and a few layers of fine sand. The concentration of plant and shell fragments was higher toward the lower portion. The facies included subtidal molluscan species such as Paratapes undulatus (Born 1778), Rhinoclavis sordidula (Gould 1849), Macoma tokyoensis Makiyama, 1927, and intertidal species such as Pirenella pupiformis Ozawa and Reid, 2016. Bioturbation, e.g., burrows, and concretions were also found throughout this sediment. In the depth interval of 20.28–20.30 m, the ostracod taxa Loxoconcha uranouchiensis, Spinileberis quadriaculeata, Ambtonia obai, and Krithe japonica occurred. The 14C ages of the three pairs of plant and shell samples from 20.48–25.73 m depth were 6470–8440 BP (7293–9285 cal BP, 2σ ranges).
Interpretation
The fine mud with its few terrestrial plant remains and intertidal to subtidal molluscan taxa suggests a low-energy condition as deep as the intertidal to subtidal zone. The ostracode taxa dwell in muddy or sandy bottom of an inner bay (e.g. Ishizaki Reference Ishizaki1968). In particular, A. obai and K. japonica flourish in the deep basin of an inner bay (e.g., Yasuhara and Irizuki Reference Yasuhara and Irizuki2001). The decrease of weight in plant and shell detritus with decreasing depth (Figure 2) indicates an upward deepening of water depth associated with a change in sea level and/or transportation of sediments.
K-Ah Volcanic Ash Facies (core depths of 13.56–20.07 m)
Description
This facies was characterized by a basal gravel bed at a depth of 19.68–20.07 m and overlaying sand/mud alternating beds. The basal gravel bed consisted of angular gravels, rock-reef mollusks such as Chama spp. and Crassostrea sp., and coral fragments. The alternating beds of sand and mud had planar lamination with a few burrows and other examples of bioturbation. The facies contained subtidal species of mollusks such as R. sordidula and the subtidal and intertidal dwelling Acar plicatum (Dillwyn 1817). A tephra horizon was identified by the high concentration of volcanic glass with bubble walls and small amounts of brown glass at a depth of 19.65 m.
Interpretation
The volcanic glass was correlated with the widespread K-Ah tephra (Machida and Arai Reference Machida and Arai2003). The age of the K-Ah layer in the terrestrial curve was consistent with the eruption age of 7165–7303 cal BP (Smith et al. Reference Smith, Staff, Blockley, Bronk Ramsey, Nakagawa, Mark, Takemura and Danhara2013). The gravel bed at the base of K-Ah was identified as an event deposit related to the K-Ah eruption. Angular gravel, shells of rock-reef species, and fragments of coral strongly imply strong currents from outside the inner bay. A similar deposit was also reported in the Oita area (Fujiwara et al. Reference Fujiwara, Machida and Shiochi2010; Nakanishi et al. Reference Nakanishi, Takemura, Matsuyama, Shimoyama, Hong and Okuno2017c), northwest of the drilling site, and Yakushima Island (Geshi et al. Reference Geshi, Maeno, Nakagawa, Naruo and Kobayashi2017; Nanayama and Maeno Reference Nanayama and Maeno2018), southwest of the drilling site (Figure 1B). They suggest that a reexamination of 7.3 ka tsunami event (Maeno et al. Reference Maeno, Imamura and Taniguchi2006; Maeno and Imamura Reference Maeno and Imamura2007) will be needed, within 300 km from the caldera. The parallel-laminated sand layers and mud layers with burrows and bioturbation imply a rapid accumulation because they contain almost no plant and shell fossils (Figure 2). This is supported by the 14C ages from the lower and upper facies: 2.2 cm/yr.
Deltaic Inner Bay Facies (core depths of 2.16–13.56 m)
Description
This facies consisted of fine-grained soft mud with plants, and shells intercalated with sand and conglomerate layers. Bioturbation, burrows, and concretions occurred throughout this sediment. At a depth of 6.24–10.49 m, the subtidal species of mollusks such as P. undulatus and Dosinella angulosa (Philippi 1847) were recognized. At a depth of 2.27–5.45 m, the subtidal to intertidal species such as Ruditapes philippinarum (Adams and Reeve 1850) and Anomalodiscus squamosus (Linnaeus 1758), and intertidal species such as Batillaria multiformis (Lischke 1869) and P. pupiformis, were found. In the interval of 12.58–12.60 m depth, ostracod taxa such as L. uranouchiensis, S. quadriaculeata, and Cytheromorpha acupunctata were found. The 14C ages of seven pairs of plant and shell samples from 2.26–12.24 m depth were 490–6360 BP (501–7157 cal BP, 2σ ranges).
Interpretation
The fine mud with few terrestrial plant remains and abundant marine shells also suggests that this sediment was deposited in a deep water environment. The ostracod taxa dwell in sandy or muddy bottom in a bayhead (Ishizaki Reference Ishizaki1968; Yasuhara and Irizuki Reference Yasuhara and Irizuki2001). The change in molluscan assemblages from subtidal to intertidal species indicates that the marine environment became shallower, probably as a result of river-mouth progradation. The increase of weight in plant and shell detritus with decreasing depth (Figure 2) also supports an upward shallowing of water depth. Thus, we concluded that this sediment formed in a deltaic inner bay environment.
Tidal Flat Facies (core depths of 1.36–2.16 m)
Description
This facies could be divided into an upper unit consisting of alternating beds of sandy mud and coarse sand, and a lower unit of organic-rich mud bed. Intertidal species of mollusks such as P. pupiformis, and brackish to freshwater Clithon retropictum (v. Martens, 1878) were recognized at a depth of 2.09–2.13 m.
Interpretation
We inferred that this sediment accumulated on a tidal flat because it contains organic-rich mud and intertidal to freshwater shells.
Embankment (core depths of 0.00–1.36 m)
Description
This facies consisted of a sandy gravel bed. A piece of pottery and a piece of brick were found in the upper part of this sediment.
Interpretation
We concluded that this sediment was an embankment formed by humans because of the artificial products in it and the land-use history.
DISCUSSION
Accumulation Curves
On the basis of 23 radiocarbon ages from the SKM core, two accumulation curves were constructed according to the ages of plant remains and shells, which were interpreted using the scatter pattern in an age/depth diagram and using stratigraphic interpretation (Figure 2). The age/depth diagram indicated that four (4/23 = 15%) samples should be ignored in the interpretation of the accumulation curves and for MRE. These samples included a pair sampled from a depth of 30.335 m that was 580–1680 yr younger than pairs from a depth of 22.855–25.73 m and previous ages. We determined that these samples, KGM-OWd180047 and OCa180023, were dropped from the upper borehole during the drilling process. In contrast, a shell fragment from a depth of 3.57 m and a piece of wood from a depth of 2.26 m were 820–1110 yr older than their paired samples and previous ages. We judged that these samples, OCa180014 and OWd180037, were reworked because no problems were found in the sample treatment and measurement procedures. This sediment reworking would result from the low accumulation rate of the environment (ca. 0.08 cm/yr).
Marine Reservoir Effects of the SKM Core and the Trend
Eight offsets in the 14C ages between pairs of plant remains and shells, induced by the MRE, were interpreted from the same horizons of the SKM core from 4100–9200 cal BP (Figure 2). The reservoir ages (R) from the transgressive inner bay sediment was 310 ± 70 yr (n = 3: nos. 6–8 in Figure 3). The chronological increasing trend of the MRE from 250 ± 60 to 390 ± 60 yr was identified during 7400–9200 cal BP. Similar trend was also recognized in two cores of Hakata Bay during 7500–9000 cal BP (Nakanishi et al. Reference Nakanishi, Hong, Shimoyama, Sato, Park and Lee2017a) and four cores of the west and south coast of Korea during 3000–4000, 6500–7000, and 7500–8000 cal BP (YAR-4 core; Nakanishi et al. Reference Nakanishi, Hong, Sung and Lim2013, S13, S15 and W17 cores; Nakanishi et al. Reference Nakanishi, Hong, Sung, Sung and Nakashima2015a). These changes would be related to the increased marine water influence induced by rising sea levels in this region. On the other hand, the average value from the deltaic inner bay sediment was 340 ± 80 yr (n = 5: nos. 1–5 in Figure 3). These R values are consistent with the one obtained from the transgressive inner bay sediment. The chronological decreasing trend of the MRE from 430 ± 60 to 260 ± 60 yr was identified during 4100–5100 cal BP. This trend was identified from uppermost two pairs (nos. 1–2 in Figure 3) because we could not estimate R values from upper part of the deltaic facies in low accumulation rate. Similar trend was recognized in YAR-4 core from southwest Korea during 4100–5100 cal BP (Nakanishi et al. Reference Nakanishi, Hong, Sung and Lim2013). These changes would be also related to the increased freshwater influence induced by a delta progradation.
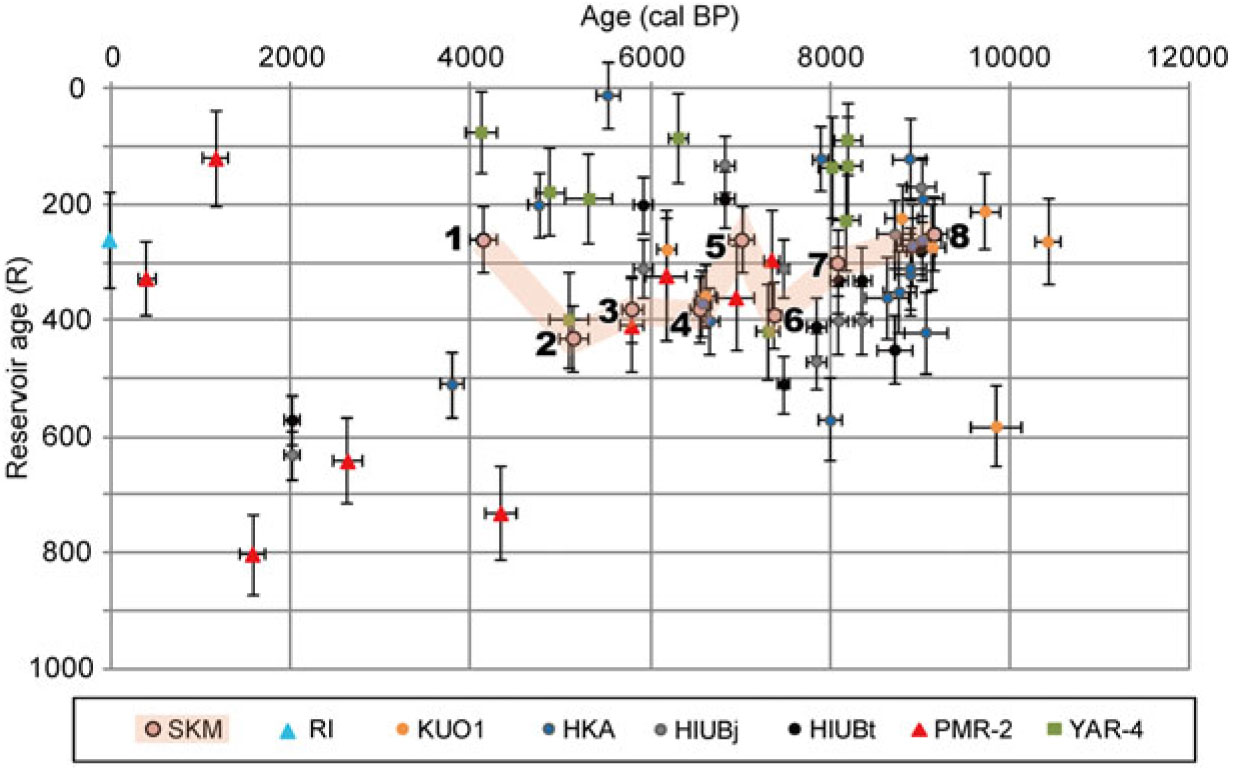
Figure 3 Reservoir ages of the SKM core and from previous studies of southwest Japan and Korea. KUO1 was from the Oita Plain (Nakanishi et al. Reference Nakanishi, Takemura, Matsuyama, Shimoyama, Hong and Okuno2017c). RI was from the Ryukyu Islands (Hirabayashi et al. Reference Hirabayashi, Yokoyama, Suzuki, Miyairi and Aze2017). HKA, HIUBj and HIUBt were from Hakata Bay (Nakanishi et al. Reference Nakanishi, Hong, Shimoyama, Sato, Park and Lee2017a). PMR-2 was from the eastern coast of Korea (Nakanishi et al. Reference Nakanishi, Hong, Sung, Nakashima, Nahm, Lim and Katsuki2017b). YAR-4 was from the southwestern coast of Korea (Nakanishi et al. Reference Nakanishi, Hong, Sung and Lim2013). Sampling sites of these samples are shown in Figure 1.
Correlation with Other East Asian Results
The calculated R values of the SKM core ranged from 260 ± 70 to 430 ± 70 yr, and the total average and standard deviation value was 330 ± 70 yr. These results were consistent with previously determined values, such as an average of 310 ± 130 yr (n = 7), ranging from 210 ± 70 to 580 ± 70 yr, obtained from the Oita Plain from 6180–10,410 cal BP (KUO1 in Figure 3; Nakanishi et al. Reference Nakanishi, Takemura, Matsuyama, Shimoyama, Hong and Okuno2017c). Moreover, these values were similar to an average of 310 ± 150 yr (n = 33), ranging from 60 ± 140 to 800 ± 150 yr, obtained from Hakata Bay during 2000–9000 cal BP (HKA, HIUBj and HIUBt in Figure 3; Nakanishi et al. Reference Nakanishi, Hong, Shimoyama, Sato, Park and Lee2017a). They were also consistent with previous values from prebomb coral skeletons under the Kuroshio environment: an average of 250 ± 70 yr (n = 11), ranging from 140 ± 40 to 350 ± 30 yr, that was obtained from Kikai Island and Ishigaki Island, from AD 1901–1947 and 1947–1950, respectively (RI in Figure 3; Hirabayashi et al. Reference Hirabayashi, Yokoyama, Suzuki, Miyairi and Aze2017). However, these values were approximately 100–180 yr less than those reported for the South China Sea and offshore from Taiwan, from 1000–8000 cal BP (Yu et al. Reference Yu, Hua, Zhao, Hodge, Fink and Barbetti2010; Yang et al. Reference Yang, Wang, Burr, Liu and Fan2018). It might reflect the variations of reservoir age affected by different branches of the Kuroshio Current. Similar differences were recognized when the average reservoir values from southwestern Japan were slightly smaller than the values from the Korean Peninsula, 380 ± 190 yr (n = 48), from 60 ± 60 to 1000 ± 60 yr, as determined from seven sediment cores from the western and southern coast dating to 100–8200 cal BP (YAR-4 in Figure 3; Nakanishi et al. Reference Nakanishi, Hong, Sung and Lim2013, Reference Nakanishi, Hong, Sung, Sung and Nakashima2015); and 450 ± 230 yr (n = 9), from 120 ± 80 to 800 ± 70 yr, as determined from a core from the east coast dating to 300–7300 cal BP (PMR-2 in Figure 3; Nakanishi et al. Reference Nakanishi, Hong, Sung, Nakashima, Nahm, Lim and Katsuki2017b). They might reflect the variations of reservoir age between the Kuroshio Current and the Tsushima Current and/or the Liman Current.
CONCLUSIONS
Seven sedimentary facies were identified (a braided river channel, a meandering river, an estuary, a transgressive inner bay, K-Ah volcanic ash, a deltaic inner bay, and a tidal flat), beneath the artificial soil of the Sukumo Plain, in the southwest coast of Shikoku Island. Reservoir ages were determined from eight marine shell and terrestrial plant pairs in sediments of the transgressive inner bay and deltaic inner bay facies, and the average value during 4100–9200 cal BP was 330 ± 70 14C yr, within 260 ± 70 to 430 ± 70 14C yr. This value measured from beneath the Kuroshio Current is similar to those measured from the Hakata Bay, Oita Bay, Ryukyu Islands, and Korean Peninsula; however, it is approximately 100 years younger than the values offshore from Taiwan and the South China Sea.
ACKNOWLEDGMENTS
The authors thank Drs. Junghun Park and Gyujun Park for their support in the AMS 14C measurement at KIGAM. This manuscript was improved by constructive comments from two anonymous referees and two editors. The study was partially funded by the Japan Society for the Promotion of Science Kakenhi grant number JP18H01310.