INTRODUCTION
The radiocarbon (14C) marine reservoir effect, the age difference between the ocean 14C and the contemporaneous atmosphere, is indispensable information for chronological control of geological and archaeological marine samples (e.g. Stuiver et al. Reference Stuiver, Pearson and Braziunas1986; Jull et al. Reference Jull, Burr and Hodgins2013; Marine Reservoir Correction Database). This effect varies in time and location associated with changes in surface ocean circulation and geomorphologic environment. To address this problem, the relationship between the reservoir effect and paleoenvironmental change has been investigated using Holocene coastal sediments in the East Asia region (Nakanishi et al. Reference Nakanishi, Hong, Sung and Lim2013, Reference Nakanishi, Hong, Sung, Sung and Nakashima2015, Reference Nakanishi, Hong, Shimoyama, Sato, Park and Lee2017a, Reference Nakanishi, Hong, Sung, Nakashima, Nahm, Lim and Katsuki2017b, Reference Nakanishi, Takemura, Matsuyama, Shimoyama, Hong and Okuno2017c, Reference Nakanishi, Tsuji, Nanayama, Yamaguchi, Kondo, Ikeda and Hong2019; Yang et al. Reference Yang, Wang, Burr, Liu and Fan2018). The reservoir ages of these areas from 100 cal BP to 10,200 cal BP were found to range between 60 and 1100 years. To identify the chronological changes in the reservoir effect and paleoenvironment of the Miyazaki Plain southeast of Kyushu Island (Figure 1A), the 14C ages of marine bioclasts and terrestrial plants from the same horizons of one core were measured. The results were then compared to values from the adjacent alluvial plains and inner bay (Nakanishi et al. Reference Nakanishi, Hong, Shimoyama, Sato, Park and Lee2017a, Reference Nakanishi, Takemura, Matsuyama, Shimoyama, Hong and Okuno2017c, Reference Nakanishi, Tsuji, Nanayama, Yamaguchi, Kondo, Ikeda and Hong2019), the Korean Peninsula (Nakanishi et al. Reference Nakanishi, Hong, Sung and Lim2013, Reference Nakanishi, Hong, Sung, Sung and Nakashima2015, Reference Nakanishi, Hong, Sung, Nakashima, Nahm, Lim and Katsuki2017b), the South China Sea (Yu et al. Reference Yu, Hua, Zhao, Hodge, Fink and Barbetti2010), and Taiwan (Yang et al. Reference Yang, Wang, Burr, Liu and Fan2018).

Figure 1 A. Study sites and modern marine reservoir ages (R) in East Asia (modified after Nakanishi et al. Reference Nakanishi, Hong, Sung, Sung and Nakashima2015). Data from Marine Reservoir Database. The contour lines were drawn using the Kriging method with the Surfer 8 code (Golden Software, Colorado, USA). 1—Kuroshio Current, 2—Tsushima Current, 3—Oyashio Current, 4—Liman Current. B. Drilling site of MIK (Miyazaki Ikime) core and geomorphologic interpretaion of the Miyazaki Plain (modified after Koike and Machida Reference Koike and Machida2001). Marine terraces of Stage 5e and Stage 1 clearly indicate that drilling site is located on an uplifting area (Nagaoka Reference Nagaoka1986; Nagaoka et al. Reference Nagaoka, Maemoku and Matsushima1991; Hasegawa et al. Reference Hasegawa, Shimoyama and Takemura2018).
STUDY SITE AND SAMPLE COLLECTION
The Miyazaki Plain is located on the southeastern side of Kyushu Island in western Japan (Figure 1A). This region is located on an uplift area associated with the convergence of the Philippine Sea Plate, because marine terraces formed in the late Pleistocene and Holocene are well distributed (Figure 1B, Fitch and Scholz Reference Fitch and Scholz1971; Nagaoka Reference Nagaoka1986; Nagaoka et al. Reference Nagaoka, Maemoku and Matsushima1991; Hasegawa et al. Reference Hasegawa, Shimoyama and Takemura2018). The coastal water around the plain is influenced by the warm Kuroshio Current, which originates from the southern area of the Northern Pacific Ocean. The Miyazaki alluvial plain was mainly formed by the Oyodo, Omaru, and Hitotsuse Rivers flowing from south to north. A melange matrix of accretionary complexes of the Early Cretaceous and Early Oligocene and volcanic rocks of the Early Pleistocene and Holocene form the bedrock of this catchment, in which no carbonate rocks are present (Kino et al. Reference Kino, Kageyama, Okumura, Endo, Fukuta and Yokoyama1984). A sediment core (MIK: Miyazaki Ikime) with a length of approximately 9 m was obtained from the southern part of the plain (31°56'42.2"N, 131°22'280"E; elevation +14.08 m). Ancient tombs (Ikime Kofun gun) are distributed on marine terraces of the Late Miocene and Holocene, and non-alkaline pyroclastic flow deposit is distributed around the drilling site (Kino et al. Reference Kino, Kageyama, Okumura, Endo, Fukuta and Yokoyama1984; Miyazaki City Board of Education 2010). The sample collection was started from a depth of 6.60 m because the drilling site occupied a thick embankment to construct a playground. MIK cores with a depth of 6.60–15.55 m were collected with 1.0-m intervals using a double-walled tube sampler. The total recovery was almost 96%.
METHODS
Sedimentological Analysis
The MIK cores were split lengthwise into two halves and photographed. Their lithologies, colors, sedimentary structures, textures, contract characteristics, fossil components, and grain sizes were investigated. In addition, for a better understanding of the sedimentary environments, mollusk assemblages were identified based on the classification of Okutani (Reference Okutani2000). To determine the physical properties of the sediments, sand contents and bulk densities were measured for 17 samples with lengths of 3 to 5 cm. Using the separated sand particles, assemblages of foraminifera and ostracoda were also identified.
AMS 14C Dating
Ten terrestrial macrofossils and ten carbonate marine samples in good condition were collected from the cores for accelerator mass spectrometry (AMS) 14C dating. The sampling depths are listed in Table 1. Fragile samples such as leaves and thin bivalves as well as articulated shells of dominant species were selected as far as possible because they are less likely to have been reworked. Samples were washed repeatedly in an ultrasonic cleaner and then organic samples were cleaned chemically using acid-alkali-acid or acid treatment to remove secondary contaminants. Samples of radiocarbon-free wood and the IAEA C-1 reference material were treated using the same procedure for blank-control measurements. The carbonate samples were powdered by mortar and pestle. All the samples including NIST OxII, IAEA C-7, and C-8 were combusted in an elemental analyzer, and the CO2 gas was purified cryogenically in a high-vacuum automatic preparation system (Hong et al. Reference Hong, Park, Kim, Woo, Kim, Choi and Kim2010a; Park et al. Reference Park, Hong, Woo, Choi, Kim and Kim2010) and then converted into graphite by reduction with Fe powder and hydrogen gas in a quartz tube. The 14C ages of the samples were measured with the standard samples at the AMS facility at the Korea Institute of Geosciences and Mineral Resources (KIGAM) (Hong et al. Reference Hong, Park, Sung, Woo, Kim, Choi and Kim2010b). The isotopic fractionations were corrected by δ13C values, measured at the AMS facility. The 14C ages of the terrestrial samples were converted into calendar dates using IntCal13 (Reimer et al. Reference Reimer, Bard, Bayliss, Beck, Blackwell, Bronk Ramsey, Buck, Cheng, Edwards, Friedrich, Grootes, Guilderson, Haflidason, Hajdas, Hatté, Heaton, Hoffmann, Hogg, Hughen, Kaiser, Kromer, Manning, Niu, Reimer, Richards, Scott, Southon, Staff, Turney and van der Plicht2013) and CALIB 7.1 (Stuiver et al. Reference Stuiver, Reimer and Reimer2018).
Table 1 Radiocarbon ages from MIK core. Errors are 1 sigma ranges. Medium depths with an asterisk (*1–8) are those used to measure the marine reservoir age.

RESULTS
Based on our core analysis, four sedimentary facies were identified, namely tidal flat, inner bay, Kikai-Akahoya (K-Ah: Machida and Arai Reference Machida and Arai2003) volcanic ash, and delta plain, as illustrated in Figure 2. These lithofacies along with remarks; depositional environments; assemblages of mollusk, ostracoda, and foraminifera; sand content; bulk density; δ13C values of bioclasts measured on the AMS; and 14C ages of the facies are described below. The 14C ages are listed in Table 1, and an age/depth diagram is provided in Figure 3.

Figure 2 Sediment column, interpretation of paleoenvironments, sand content, bulk density, δ13C values of bioclasts measured on the AMS, and weights of 14C dating samples of the MIK core.

Figure 3 Accumulation curve and reservoir age of the MIK core.
Tidal Flat Facies (core depth of 12.85–15.55 m)
Description
This sedimentary facies consisted of a sandy silt bed with a few sand layers, terrestrial plant fragments, crustaceans such as Brachyura and Pleocyemata, shells, bioturbation, and burrows. This sediment consisted of two units. The upper unit consisted of alternating beds of silty sand and lenticular-bedding medium sand layers with shells, crustaceans, burrows, plant fragments, and pumice at depths from 12.85 to 14.20 m. The lower unit included sandy silt layers with plant fragments, crustaceans, and bioturbation at depths from 14.20 to 15.55 m. Intertidal species of mollusks, such as Cerithium coralium Kiener, 1841 and Estellarca olivacea (Reeve, 1844), which live in environments from subtidal to intertidal, were found in this facies. No ostracoda or foraminifera were identified. The sand content and bulk density were 0.8 to 13% and 1.10 to 1.46 g/cm3, respectively, at depths from 13.28 to 15.53 m. The δ13C values of bioclasts were distributed from –4.7 to –19.3‰. The 14C ages of leaves, Brachyura, and intertidal bivalves from depths of 12.96 to 15.415 m were measured to be 7170 to 7850 BP.
Interpretation
The coexistence of terrestrial plant remains and intertidal mollusks indicates that this sediment was formed in an environment with a mixture of freshwater from a terrestrial source and saline water from a marine source, such as a tidal flat or an estuary. The decrease in the number of crustaceans and the amount of bioturbation with decreasing depth indicates an upward-deeping environments associated with a change in sea level and/or the transportation of sediments. The increase in the δ13C value with decreasing depth clearly supports this interpretation. The absence of rootlets in this sediment implies that it was not deposited in an area shallower than an intertidal zone.
Inner Bay Facies (core depth of 9.70–12.85 m)
Description
This sediment consisted of fine soft mud and sandy silt beds with a few sand layers, marine shells, bioturbation, burrows, terrestrial plant fragments, Euechinoidea, and crustaceans. This sediment was also devided into two units. The upper unit contained a sandy silt bed with parallel-laminated medium sand layers with shells, crustaceans, burrows, plant fragments, and concretions at depths from 9.70 to 11.40 m. The lower unit contained a fine soft mud bed with shells, burrows, and bioturbation at depths from 11.40 to 12.85 m. Subtidal species of mollusks, such as Macoma tokyoensis Makiyama, 1927, E. olivacea living in subtidal and intertidal environments, and C. coralium living in intertidal environments were found in this facies. Benthic foraminifera, such as Ammonia beccarii (Linnaeus, 1758) were recognized at depths from 11.70 to 12.81 m; however, no ostracoda were found in this facies. The sand content and bulk density were 0.7 to 21% and 0.96 to 1.50 g/cm3, respectively, at depths ranging from 10.26 m to 12.79 m. The δ13C values of the bioclast were 2.6 to –8.4‰. The 14C ages of plant fragments, leaves, and subtidal to intertidal mollusks samples from depths of 10.62 to 12.41 m were from 6480 to 7590 BP.
Interpretation
The fine mud with subtidal shells and foraminifera as well as a few terrestrial plant remains suggests that this sediment was deposited in a deeper water environment, such as an inner bay. The decrease in the number of crustaceans and the amount of bioturbation with decreasing depth indicates an upward-deeping environment associated with a change in sea level and/or the transportation of sediments. The increase in the δ13C values of shells clearly supports this interpretation because δ13C level is generally higher in marine water than fresh water. Deeper marine environment has less chance to be affected by fresh water.
Kikai-Akahoya Volcanic Ash Facies (core depth of 8.15–9.70 m)
Description
This facies could be divided into an upper unit having sandy mud with volcanic ash at depths from 8.15 to 9.00 m and a lower unit containing alternating beds of sand and mud at depths from 9.35 to 9.70 m. Unfortunately, the boundary between these units corresponded to the boundary of the sediment cores. The layer of basal sand/mud alternating beds was 35 cm thick, and had mud clasts, terrestrial plant fragments, planar lamination, and no marine carbonate samples. The sand layers mainly consisted of fine to medium sand. A tephra horizon was identified by a high concentration of volcanic glass with bubble walls, small amounts of brown glass, and pumice fragments at a depth of 9.55 m. This was correlated with the widespread K-Ah tephra (Machida and Arai Reference Machida and Arai2003). The upper unit of sandy mud consisted of parallel-laminated abundant volcanic glass, pumice grains 2–3 mm in diameter, plant fragments, and the uppermost rootlets. The sand content and bulk density were 16% and 1.40 g/cm3, respectively, at the depth of 9.67 to 9.70 m. The 14C age of a twig from the depth of 9.565 m was 6100 ± 40 BP.
Interpretation
The sand bed at the base of the K-Ah was identified as a tsunami deposit generated by the K-Ah eruption (Maeno et al. Reference Maeno, Imamura and Taniguchi2006; Maeno and Imamura Reference Maeno and Imamura2007). This tsunami deposit was also reported at the Oita area (Fujiwara et al. Reference Fujiwara, Machida and Shiochi2010; Nakanishi et al. Reference Nakanishi, Takemura, Matsuyama, Shimoyama, Hong and Okuno2017c), north of the drilling site, at the Sukumo area (Tsuji et al. Reference Tsuji, Nanayama, Yamaguchi, Ikeda, Nakanishi, Kondo, Maeno, Hongo and Kumura2018; Nakanishi et al. Reference Nakanishi, Tsuji, Nanayama, Yamaguchi, Kondo, Ikeda and Hong2019), northeast of the drilling site (Figure 1B). The parallel-laminated sandy mud implies that it was formed in an intertidal environment. The uppermost rootlets in this sediment suggest that it had been changed to an area shallower than the intertidal zone.
Delta Plain Facies (core depth of 7.60–8.15 m)
Description
This sediment consisted of parallel-laminated mud with abundant terrestrial plants. The concentration of plant fragments was higher toward the upper portion. The sand content and bulk density were 38 to 88% and 0.33 to 1.18 g/cm3, respectively, at the depth of 7.69 to 8.135 m.
Interpretation
The fine parallel-laminated mud with abundant terrestrial plant remains suggests that this sediment was deposited in a freshwater environment around a coast, such as a delta plain. The increase in the sand content and the decrease in bulk density with decreasing depth reflect the increase of plant remains associated with a change in sea level and/or the transportation of sediments.
Artificial Soil Facies (core depth of 6.60–7.60 m)
Description
This sediment could be divided into an upper unit with alternating beds of sandy mud from the depth of 6.60 to 6.70 m and muddy sand and a lower unit including an organic mud bed with abundant plant remains, such as leaves, wood fragments, and rootlets at depths from 6.70 to 7.60 m. The upper unit had a deformation structure. Gravel layers were found around depths of 7.00 and 7.50 m. The sand content and bulk density were 87% and 0.22 g/cm3, respectively, at depths of 6.96 to 7.00 m. The 14C age of leaves from the depth of 6.955 m was 3050 ± 40 BP.
Interpretation
The upper unit was artificial filler for the construction of a playground according to the land use history. The lower unit could be a paddy field since a similar organic mud bed was interpreted in this way for an archaeological excavation around the drilling site (Miyazaki City Board of Education 2010). Therefore, we concluded that this sediment was formed by human activity.
DISCUSSION
Accumulation Curves
Two accumulation curves were constructed according to 20 14C ages of plant remains and shells from the MIK core, which were interpreted according to the scattering pattern in an age/depth diagram and by stratigraphic study (Figure 3). The terrestrial accumulation curve was consistent with the eruption age of K-Ah, 7165–7303 cal BP (Smith et al. Reference Smith, Staff, Blockley, Bronk Ramsey, Nakagawa, Mark, Takemura and Danhara2013), and the tephra horizon. The age/depth diagram clearly indicated that no samples used in this study were reworked.
Tectonic Uplift
The K-Ah tephra and tsunami deposit was identified at the elevation of +4.38 m. This is significantly higher than on the Oita Plain with an elevation of –36 m, 147 km north of the drilling site of the MIK core (Nakanishi et al. Reference Nakanishi, Takemura, Matsuyama, Shimoyama, Hong and Okuno2017c), and the Sukumo Plain with an elevation of –19 m, 167 km northeast of the MIK coring site (Nakanishi et al. Reference Nakanishi, Tsuji, Nanayama, Yamaguchi, Kondo, Ikeda and Hong2019). These horizons were identified at the uppermost part of the transgressive inner bay or prodelta sediments, and then they had been covered by deltaic inner bay or deltaic sediments. The change in sedimentary system would be associated with not only the glacio-hydroisostatic sea-level rise during the Holocene (Yokoyama et al. Reference Yokoyama, Nakada, Maeda, Nagaoka, Okuno, Matsumoto, Sato and Matsushima1996), but also a huge amount (ca. 100 km3) of K-Ah volcanic ash (Machida and Arai Reference Machida and Arai2003) and local tectonic movement due to the convergence of the Philippine Sea Plate (Fitch and Scholz Reference Fitch and Scholz1971). The uplifted K-Ah tephra and tsunami deposit in the Miyazaki Plain was consistent with the geomorphological interpretation of marine terraces and modern geophysical observation (Nagaoka Reference Nagaoka1986; Nagaoka et al. Reference Nagaoka, Maemoku and Matsushima1991; Hasegawa et al. Reference Hasegawa, Shimoyama and Takemura2018).
Marine Reservoir Effects
Eight offsets in 14C ages between plant remains and bioclast pairs from the same horizons of the MIK core were interpreted to evaluate the marine reservoir effect for 7300 to 8200 cal BP (Figure 3). The calculated reservoir ages (R) ranged from 440 ± 60 to 610 ± 60 years, and the total average and standard deviation value was 530 ± 50 years for this time span. The average values from the tidal flat and inner bay sediments were 520 ± 30 (n = 5) and 540 ± 80 (n = 4) years, respectively. In contrast, the average δ13C values of the bioclasts from the tidal flat and inner bay sediments were –12.3 ± 5.7 and –3.6 ± 4.8‰, respectively. The relationship indicates that the R ages were almost constant although their δ13C values were clearly different at the Miyazaki Plain. Similarly, high R values were also reported on the isolated lagoon from marine inputs at the Palavasian lagoon complex on the south coast of France (Sabatier et al. Reference Sabatier, Dezileau, Blanchemanche, Siani, Condominines, Bentaleb and Piques2010) and on the east coast of Korea (Nakanishi et al. Reference Nakanishi, Hong, Sung, Nakashima, Nahm, Lim and Katsuki2017b).
The results for the MIK core were approximately 0 to 200 years larger than the previous values obtained from Hakata Bay, the Oita plain, the Sukumo Plain, and the South China Sea for 7000 to 9000 cal BP (Figure 4, Nakanishi et al. Reference Nakanishi, Hong, Shimoyama, Sato, Park and Lee2017a, Reference Nakanishi, Takemura, Matsuyama, Shimoyama, Hong and Okuno2017c, Reference Nakanishi, Tsuji, Nanayama, Yamaguchi, Kondo, Ikeda and Hong2019; Yu et al. Reference Yu, Hua, Zhao, Hodge, Fink and Barbetti2010). However, the values were approximately 0 to 400 years larger than that those obtained from the Korean Peninsula and off of Taiwan for 7000 to 9000 cal BP (Figure 4, Nakanishi et al. Reference Nakanishi, Hong, Sung and Lim2013, Reference Nakanishi, Hong, Sung, Sung and Nakashima2015, Reference Nakanishi, Hong, Sung, Nakashima, Nahm, Lim and Katsuki2017b; Yang et al. Reference Yang, Wang, Burr, Liu and Fan2018). A significantly large difference (ca. 600 years) in R value was recognized between the Miyazaki Plain and the southwestern coast of the Korean Peninsula for 7800 to 8200 cal BP (Figure 4). This timing corresponds to the 8.2 ka cooling event (Alley et al. Reference Alley, Mayewski, Sowers, Stuiver, Taylor and Clark1997; Park et al. Reference Park, Park, Yi, Kim, Lee and Jin2018) and the early Holocene sea-level rise (Hori and Saito Reference Hori and Saito2007). These sudden paleoenvironmental changes might have caused regional fluctuations in the marine reservoir effect.
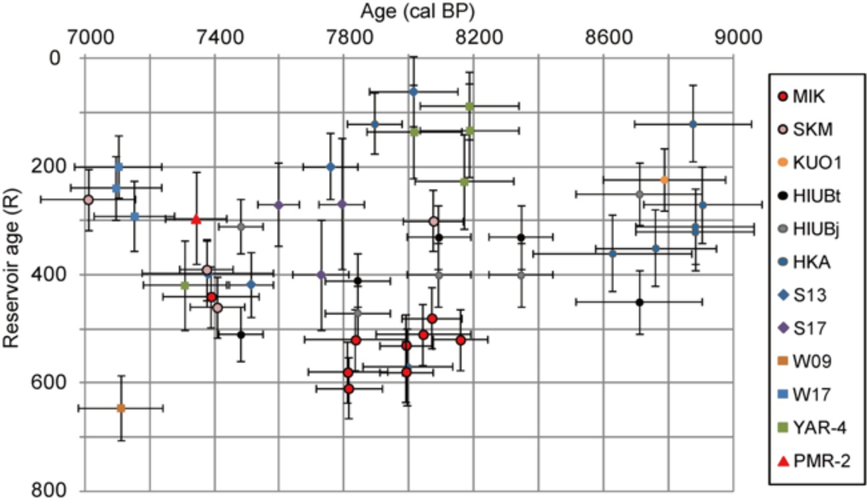
Figure 4 Reservoir ages of the MIK core and cores in previous studies. SKM was from the Sukumo Plain (Nakanishi et al. Reference Nakanishi, Tsuji, Nanayama, Yamaguchi, Kondo, Ikeda and Hong2019). KUO1 was from the Oita Plain (Nakanishi et al. Reference Nakanishi, Takemura, Matsuyama, Shimoyama, Hong and Okuno2017c). HKA and HIUB were from Hakata Bay (Nakanishi et al. Reference Nakanishi, Hong, Shimoyama, Sato, Park and Lee2017a). S13, S17, W09 and W17 were from the south and west coasts of Korea (Nakanishi et al. Reference Nakanishi, Hong, Sung, Sung and Nakashima2015). YAR-4 was from the southwest coast of Korea (Nakanishi et al. Reference Nakanishi, Hong, Sung and Lim2013). PMR-2 was from the east coast of Korea (Nakanishi et al. Reference Nakanishi, Hong, Sung, Nakashima, Nahm, Lim and Katsuki2017b).
CONCLUSIONS
Four sedimentary facies were identified, namely, tidal flat, inner bay, K-Ah volcanic ash, and delta plain beneath the Miyazaki Plain off the southeastern coast of Kyushu Island. Reservoir ages were determined from nine marine bioclast and terrestrial plant pairs in the sediments of the transgressive tidal flat and inner bay facies, and the average value for 7300 to 8200 cal BP was 530 ± 60 years, ranging from 370 ± 70 to 610 ± 70 14C years. This value is approximately 0 to 200 years larger than values obtained from Hakata Bay, the Oita Plain and the Korean Peninsula for 7000 to 9000 cal BP, and 0 to 400 years larger than the values obtained off of Taiwan and the South China Sea for 7000 to 9000 cal BP.
ACKNOWLEDGMENTS
This manuscript was improved by costructive comments from Prof. Alessandro Amorosi and an anonymous referee. This core was obtained by the Grants-in-Aid for Scientific Research (Kakenhi 16K03159) funded by the Japan Society for the Promotion of Science (JSPS). This study was also partially funded by the JSPS Kakenhi grant number 18H01310 and supported by the Basic Research Project of the Korean Institute of Geoscience and Mineral Resouces (KIGAM) funded by the Ministry of Science and ICT of Korea.