Background
Altered reward processing has been proposed to underlie the challenges that individuals with autism spectrum disorder (ASD) face in social interactions. The social motivation hypothesis postulates that individuals with ASD from early in development do not perceive social stimuli to be as rewarding as typically developing individuals, which may have an impact on the development of social learning and social skills.Reference Chevallier, Kohls, Troiani, Brodkin and Schultz1 Newer models extending atypicalities beyond social stimuli to restricted and repetitive behaviours and interests stimuli have recently been proposed.Reference Kohls, Yerys and Schultz2,Reference Tschida and Yerys3
Neurobiological evidence in favour of a social motivation atypicality is, however, mixed.Reference Tschida and Yerys3–Reference Janouschek, Chase, Sharkey, Peterson, Camilleri and Abel5 To assess atypical motivation, reward processing is commonly investigated during the anticipation of a potential reward (‘wanting’), the delivery of the reward (‘liking’) or during both phases. Further, different types of rewards can be assessed, with non-social (usually monetary) rewards being most commonly investigated across psychiatric conditions, and it has been postulated that there is a specific impact on social rewards, as detailed in the social motivation hypothesis. Supporting the concept of atypical social reward processing in ASD, one study showed reduced activation in the ventral striatum,Reference Scott-Van Zeeland, Dapretto, Ghahremani, Poldrack and Bookheimer6 a key region for reward processing comprising the nucleus accumbens and caudate head, compared with control participants when receiving social rewards. A similar effect was observed in another study in more dorsal parts of the striatum.Reference Delmonte, Balsters, McGrath, Fitzgerald, Brennan and Fagan7 However, other studies did not find functional striatal differences between ASD and typically developing individuals for social rewards during deliveryReference Dichter, Richey, Rittenberg, Sabatino and Bodfish8,Reference Kohls, Antezana, Mosner, Schultz and Yerys9 or anticipation.Reference Delmonte, Balsters, McGrath, Fitzgerald, Brennan and Fagan7,Reference Dichter, Richey, Rittenberg, Sabatino and Bodfish8 Similarly mixed results exist for non-social rewards: although some previous studies report ventral striatum hypoactivation in individuals with ASD when receiving monetary rewards,Reference Kohls, Schulte-Ruther, Nehrkorn, Muller, Fink and Kamp-Becker10–Reference Dichter, Felder, Green, Rittenberg, Sasson and Bodfish12 this has not been foundReference Dichter, Richey, Rittenberg, Sabatino and Bodfish8 or only at uncorrected thresholdsReference Kohls, Thonessen, Bartley, Grossheinrich, Fink and Herpertz-Dahlmann13 elsewhere. Results for the anticipation of monetary rewards are also inconsistent with some studies suggesting ventral striatum hypoactivation in ASD individuals see for example references Reference Dichter, Richey, Rittenberg, Sabatino and Bodfish8,Reference Dichter, Felder, Green, Rittenberg, Sasson and Bodfish12 , whereas another showed no difference between participants with ASD and typically developing participants.Reference Delmonte, Balsters, McGrath, Fitzgerald, Brennan and Fagan7
Some of the inconsistency of previous findings is likely because of the heterogeneity of ASD itself, but also the relatively small sample sizes examined (ranging between 13 and 39 individuals per group). Two recent meta-analyses have partly addressed the latter issue by summarising the current literature.Reference Clements, Zoltowski, Yankowitz, Yerys, Schultz and Herrington4,Reference Janouschek, Chase, Sharkey, Peterson, Camilleri and Abel5 Comparing individuals with ASD to typically developing individuals, the authors reported striatal hypoactivation during social as well as non-social rewards in ASD. Only Clements et alReference Clements, Zoltowski, Yankowitz, Yerys, Schultz and Herrington4 investigated anticipation and delivery phases separately. They report hypoactivation of the left caudate during anticipation of social rewards, and hyperactivation during the anticipation of non-social rewards. In contrast, during reward delivery, striatal (left nucleus accumbens and caudate) hyperactivation to social rewards and right caudate hypoactivation to non-social rewards were observed in ASD. These findings suggest opposing atypicality patterns for social and monetary reward types between reward phases and do not imply typical non-social reward processing in ASD.
Across the seven studies assessing social reward processing, caudate hypoactivation was linked (albeit only at trend level) to the severity of autistic traits as measured with the Social Responsiveness Scale (SRS). This meta-analysis was an important first step to provide a more comprehensive insight into atypical reward processing in ASD. However, the number of included studies is still small (for example only three studies allowed for the differentiation between reward phases for social reward) and should be regarded as exploratory. Further, task designs were heterogeneous, which might have increased variability in brain responses and influenced task-specific effects. Finally, although some studies included in the meta-analysis administered social and non-social reward conditions in the same sample, some only assessed one type of reward, limiting direct comparability.
On top of these, another challenge is the fact that ASD and attention-deficit hyperactivity disorder (ADHD) frequently co-occur and atypical reward processing with ventral striatum hyporesponsiveness for monetary rewards is often reported in individuals with ADHD.Reference Plichta and Scheres14,Reference Grimm, van Rooij, Hoogman, Klein, Buitelaar and Franke15 However, co-occurring ADHD or symptoms have not been examined in the majority of studies exploring reward processing in ASD (for exceptions, see references Reference Kohls, Thonessen, Bartley, Grossheinrich, Fink and Herpertz-Dahlmann13,Reference Chantiluke, Christakou, Murphy, Giampietro, Daly and Ecker16 ).
Aims
Hence, the brain functional mechanisms underpinning reward processing in ASD remain unclear. We therefore investigated reward-related brain responses in a large, well-powered sample of individuals with ASD. The Longitudinal European Autism Project (LEAPReference Loth, Charman, Mason, Tillmann, Jones and Wooldridge17) provides a deeply phenotyped data-set of children, adolescents and adults with and without ASD who performed a social and a monetary reward task. The task was chosen based on its ability to reliably elicit ventral striatum reward signallingReference Plichta, Schwarz, Grimm, Morgen, Mier and Haddad18 and allows for the analysis of both reward anticipation and reward delivery phases. We comprehensively assessed differences in reward signalling based on clinical diagnosis as well as dimensional autistic traits. Based on the recent meta-analysis,Reference Clements, Zoltowski, Yankowitz, Yerys, Schultz and Herrington4 compared with typically developing individuals, we hypothesised that neurofunctional responses in the ventral striatum would show a pattern of increased activity in ASD during monetary reward anticipation and reduced activity during social reward anticipation – and the opposing pattern during reward delivery. We expected to observe this pattern in categorical case–control comparisons as well as in dimensional analyses based on autism traits. Further, based on previous findings in the, to our knowledge, only study exploring reward processing in individuals with autism, ADHD, and co-occurring autism and ADHD,Reference Chantiluke, Christakou, Murphy, Giampietro, Daly and Ecker16 we hypothesised an additive effect of ADHD co-occurrence, with reward processing being most severely altered in autistic individuals with elevated ADHD symptoms.
Method
Experimental procedure
Participants
In the LEAP study, 437 individuals with ASD and 300 typically developing individuals, aged between 6 and 30 years, underwent comprehensive clinical, cognitive and magnetic resonance imaging (MRI) assessment at one of six study sites: Institute of Psychiatry, Psychology and Neuroscience, King's College London, UK (KCL); Autism Research Centre, University of Cambridge, UK (UCAM); Radboud University Nijmegen Medical Centre, the Netherlands (RUNMC); University Medical Centre Utrecht, the Netherlands (UMCU); Central Institute of Mental Health, Mannheim, Germany (CIMH); University Campus Bio-Medico of Rome, Italy (UCBM).Reference Loth, Charman, Mason, Tillmann, Jones and Wooldridge17
The authors assert that all procedures contributing to this work comply with the ethical standards of the relevant national and institutional committees on human experimentation and with the Helsinki Declaration of 1975, as revised in 2008. All procedures involving patients were approved by the local ethical committees of participating centres and written informed consent was obtained from all participants or their legal guardians (for participants <18 years). For further details about the study design we refer the reader to Loth et al,Reference Loth, Charman, Mason, Tillmann, Jones and Wooldridge17 and for a comprehensive clinical characterisation of the LEAP cohort we refer the reader to Charman et al.Reference Charman, Loth, Tillmann, Crawley, Wooldridge and Goyard19 For this study, the final sample consisted of n = 212 participants with ASD and n = 181 typically developing participants (see Table 1 and Supplementary Table 1 available at https://doi.org/10.1192/bjp.2022.157). Standard operating and quality control procedures leading to the final sample are detailed in the Supplementary material.
Table 1 Participant characteristicsa

ADHD, attention-deficit hyperactivity disorder; ASD, autism spectrum disorder; CIMH, Central Institute of Mental Health, Mannheim, Germany; FD, framewise displacement; fMRI, functional magnetic resonance imaging; KCL, Institute of Psychiatry, Psychology and Neuroscience, King's College London, UK; MID monetary incentive delay task; RUNMC, Radboud University Nijmegen Medical Centre, the Netherlands; SID social incentive delay task; UCAM, Autism Research Centre, University of Cambridge, UK; UCBM, University Campus Bio-Medico of Rome, Italy; UMCU, University Medical Centre Utrecht, the Netherlands.
a. ADI-R: Autism Diagnostic Interview-Revised. Scores were computed for reciprocal interaction (social interaction), communication, and restrictive, repetitive stereotyped behaviours and interests (RRB). ADOS-2 (CSS): Autism Diagnostic Observation Schedule 2. Calibrated severity scores were computed for social affect, restricted and repetitive behaviours (RRB) and the overall total score. SRS-2: Social Responsiveness Scale-2. Total raw and total T-scores (gender + age normalised) are reported. The raw SRS-2 scores were used in our analyses. Self-rated scores were used when parent-rated scores were not available. Symptoms of ADHD, depression and anxiety were assessed with the Development and Well Being Assessment (DAWBA), generating six levels (ordinal scores 0 to 5) of prediction of the probability of a disorder (~0.1%, ~0.5%, ~3%, ~15%, ~50%, >70%).
b. ADHD research diagnosis was based on applying DSM-V criteria to symptom scores in the parent- and self-rated ADHD rating scale.
Clinical measures
Participants in the ASD group had an existing clinical diagnosis of ASD according to the DSM-IV/ICD-10 or DSM-5 criteria. ASD symptoms were comprehensively assessed using the Autism Diagnostic Interview-Revised (ADI-RReference Lord, Rutter and Le Couteur20) and Autism Diagnostic Observation Schedule 2nd edition (ADOS-2Reference Lord, Risi, Lambrecht, Cook, Leventhal and DiLavore21) within the ASD group. We used the total raw score on the Social Responsiveness Scale 2nd edition (SRS-2Reference Constantino, Davis, Todd, Schindler, Gross and Brophy22) to assess continuous autism traits, which was available across the study sample. Parent-rated scores were collected for ASD and typically developing individuals, except for typically developing adults where only the self-report was assessed. We used self-rated scores wherever parent-rated scores were not available. Parent- or self-report of a psychiatric disorder was an exclusion criterion for the typically developing group. Information on the presence of a confirmed diagnosis of ADHD was not available in our sample. As a proxy, we estimated diagnostic status by applying DSM-5 criteria based on symptom scores collected with the parent- and self-rated ADHD DSM-5 rating scales.Reference DuPaul, Power, Anastopoulos and Reid23
Experimental paradigm
We adapted a social incentive delay (SID) and a monetary incentive delay (MID) taskReference Delmonte, Balsters, McGrath, Fitzgerald, Brennan and Fagan7 as part of a reliable task battery.Reference Plichta, Schwarz, Grimm, Morgen, Mier and Haddad18,Reference Moessnang, Schafer, Bilek, Roux, Otto and Baumeister24,Reference Plichta, Grimm, Morgen, Mier, Sauer and Haddad25 For details see Fig. 1 and Supplementary material. SID and MID were collected as separate paradigms and combined during data analysis. SID was always presented first, followed by MID. The functional magnetic resonance imaging (fMRI) scanning session was preceded by a training session outside the MRI to ensure that all participants understood the task.
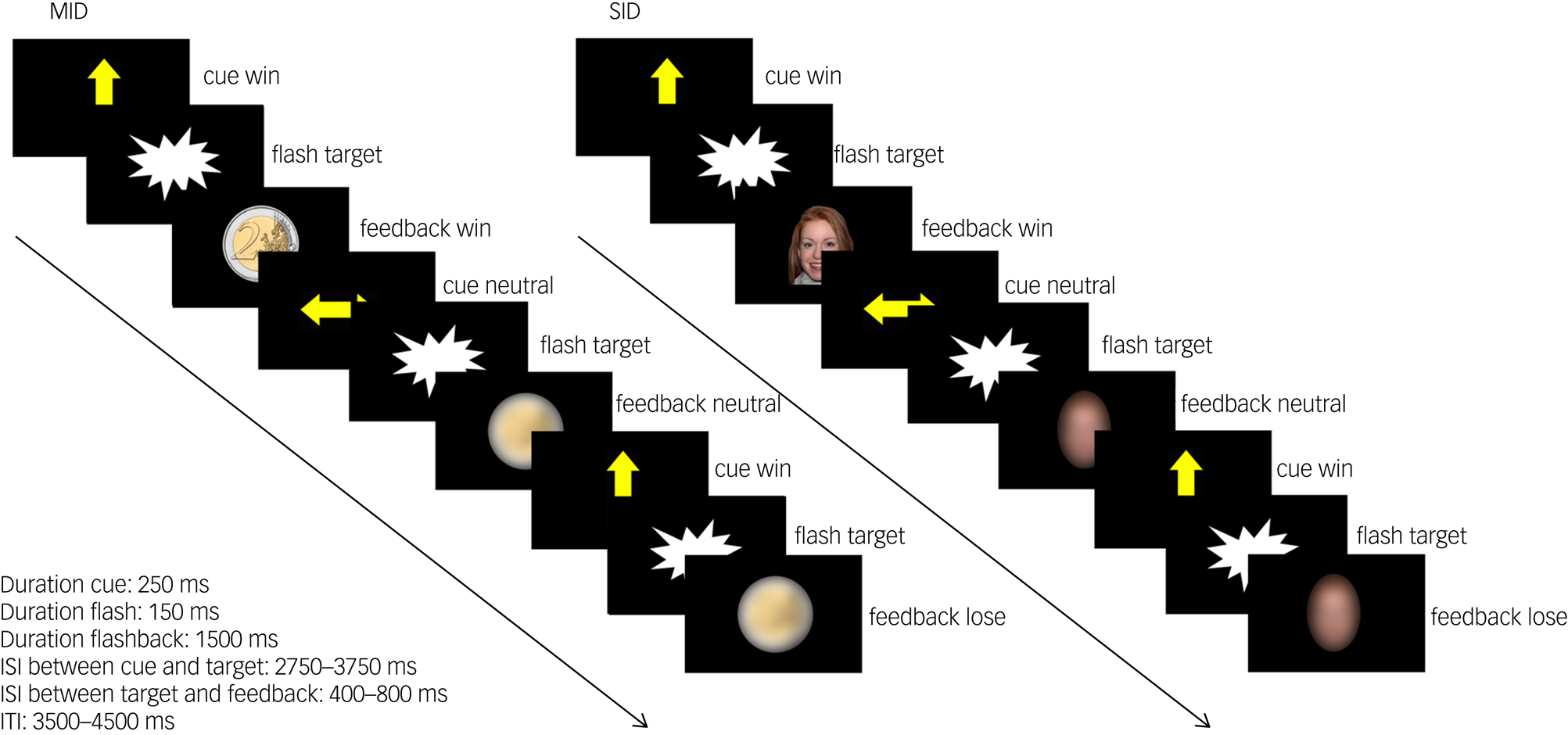
Fig. 1 Task design of the monetary incentive delay task (MID) and social incentive delay task (SID). Participants were asked to give a speeded response (button press) to a visual target (screenflash). A cue arrow pointing upwards indicated the possibility to obtain a reward if responses were given within a predefined response time window (win trial). No reward option was given in trials preceded by a horizontal cue arrow (neutral trial). Sufficiently fast responses on win trials were followed by the presentation of a 2€/£2 coin in the MID task and a smiling female face in the SID task as feedback. Blurred control stimuli were presented in neutral trials and as feedback following slow responses in win trials. Cue presentation represents reward anticipation phase, and feedback presentation represents reward delivery phase. Note that the feedback presentation was temporally decoupled from the target presentation but not from the button press. A black screen was presented during interstimulus intervals (ISI) and intertrial intervals (ITI). In total, 15 win trials and 15 neutral trials were presented in a pseudorandomised order during each task. Total task duration was 5 min per task.
fMRI data acquisition
fMRI data were acquired on 3T scanners from different manufacturers (General Electric, Philips, Siemens) and harmonised as much as possible across sites (for details see Supplementary material). Functional images were acquired using a blood-oxygen-level-dependent (BOLD)-sensitive T2*-weighted echo-planar imaging sequence (repetition time (TR) = 2 s, echo time (TE) = 30 ms, flip angle = 80°, matrix: 64 × 64, FOV: 192 × 192 mm, in-plane resolution: 3 × 3 mm, slice thickness: 4 mm, gap: 1 mm, 28 axial slices). A total of 151 volumes were obtained for each task, oriented approximately 20° steeper than the AC-PC plane.
Data analysis
fMRI data preprocessing
Image preprocessing followed standard processing routines in SPM12 (http://www.fil.ion.ucl.ac.uk/spm/), including a two-pass realignment procedure, slice time correction, registration of the functional mean image to the Montreal Neurological Institute template and spatial normalisation into standard stereotactic space, application of resulting normalisation parameters to the functional time series, resampling to 3 mm isotropic voxels and smoothing with an 8 mm full-width at half-maximum Gaussian Kernel.
Whole-brain level fMRI data analysis
SID and MID tasks were combined as two sessions in a general linear model (GLM) at the single-subject level (see Supplementary material for details). Within-subject effects were addressed at the subject-level by quantifying within-subject effects of condition as differential response to win cues as compared with neutral cues for reward anticipation and differential response to successful win compared with neutral feedback for reward delivery. Note that n = 7 individuals with ASD and n = 7 typically developing participants were excluded from analysis of reward delivery as they withheld responses to neutral trials. Additionally, to quantify differential reward-specific responses between tasks, a contrast image for the interaction between condition (win, neutral) and task (SID, MID) was calculated.
Based on within-subject contrasts we assessed reward-specific brain activation (within-subject effect of condition) and differential reward-specific responses between tasks (within-subject interaction condition × task) across the entire sample and tested for between-group differences. Contrast images were subjected to second-level GLMs with between-subject factor group (ASD versus typically developing) and covariates age, gender assigned at birth and site.
The impact of ADHD co-occurrence was explored in a separate model, where the ASD group was split into subgroups with (n = 69) and without (n = 118) co-occurring ADHD based on estimated diagnostic status (ASD+ADHD and ASD–ADHD, respectively) and compared with typically developing participants while controlling for age, gender assigned at birth and site (for group characteristics, see Table 2). Typically developing individuals with elevated ADHD scores based on DSM-5 criteria were excluded from this analysis. To assess the dimensional effect of autism and ADHD traits, SRS-2 raw scores and ADHD rating scale scores were added as an additional covariate of interest in separate models. Note that the diagnostic group was accounted for in this model, ensuring that effects were not driven by differences in group means. To explore group differences on a whole-brain level, significance was defined as P FWE < 0.05, peak-level corrected across the whole brain with a cluster threshold of k ≥ 5. Family-wise error correction (FEW) implemented in SPM12 is based on random field theory.
Table 2 Subgroup characteristicsa

ADHD, attention-deficit hyperactivity disorder; ASD, autism spectrum disorder; CIMH, Central Institute of Mental Health, Mannheim, Germany; FD, framewise displacement; fMRI, functional magnetic resonance imaging; KCL, Institute of Psychiatry, Psychology and Neuroscience, King's College London, UK; MID monetary incentive delay task; RUNMC, Radboud University Nijmegen Medical Centre, the Netherlands; SID social incentive delay task; UCAM, Autism Research Centre, University of Cambridge, UK; UCBM, University Campus Bio-Medico of Rome, Italy; UMCU, University Medical Centre Utrecht, the Netherlands.
a. ADI-R: Autism Diagnostic Interview-Revised. Scores were computed for reciprocal interaction (social interaction), communication, and restrictive, repetitive stereotyped behaviours and interests (RRB). ADOS-2 (CSS): Autism Diagnostic Observation Schedule 2. Calibrated severity scores were computed for social affect, restricted and repetitive behaviours (RRB) and the overall total score. SRS-2: Social Responsiveness Scale-2. Total raw and total T-scores (gender + age normalised) are reported. The raw SRS-2 scores were used in our analyses. Self-rated scores were used when parent-rated scores were not available. Symptoms of ADHD, depression and anxiety were assessed with the Development and Well Being Assessment (DAWBA), generating six levels (ordinal scores 0 to 5) of prediction of the probability of a disorder (~0.1%, ~0.5%, ~3%, ~15%, ~50%, >70%).
Region of interest (ROI) analysis
To increase sensitivity for putative case–control differences in the ventral striatum, we performed ROI analysis within a well-established a priori defined bilateral mask of the ventral striatum comprising the caudate head and nucleus accumbens.Reference Plichta, Schwarz, Grimm, Morgen, Mier and Haddad18 Mean contrast estimates (contrasts cue win > cue neutral and successful win > neutral) for each participant and both tasks were extracted and analysed using SPSS Software package for Windows(Version 25, IBM Corp. Armonk, NY, USA). Separate repeated measures ANOVAs with the within-subject factor task (MID, SID) and between-subject factor diagnosis (typically developing, ASD) and covariates age (mean centred), gender and study sites (dummy coded), were used to assess group differences for both reward processing phases (anticipation, delivery) in the left and right ventral striatum. To correct for investigating left and right ventral striatum activity separately, the critical alpha threshold was adjusted to P < 0.025 based on the Bonferroni procedure. Additionally, Bonferroni-correction was applied to all post hoc pairwise comparisons. To assess the effect of autism and ADHD traits, SRS-2 raw scores and ADHD rating scale scores were added as an additional covariate of interest in separate models. Interaction terms between diagnosis and SRS-2 or ADHD rating scale were added as well. The impact of ADHD co-occurrence was explored in another separate model, where the between-subject factor diagnosis comprised three levels (typically developing, ASD+ADHD and ASD–ADHD).
Results
Functional activation analysis
Reward anticipation
Whole-brain level analysis
Reward-specific brain activation differed between diagnostic groups at the whole-brain level in the right ventral striatum (F (1,384) = 22.84, P FWE = 0.017, k = 8) during reward anticipation. A post hoc T-test showed that activation was reduced in participants with ASD compared with typically developing individuals. See Fig. 2(a) and Table 3 for details.
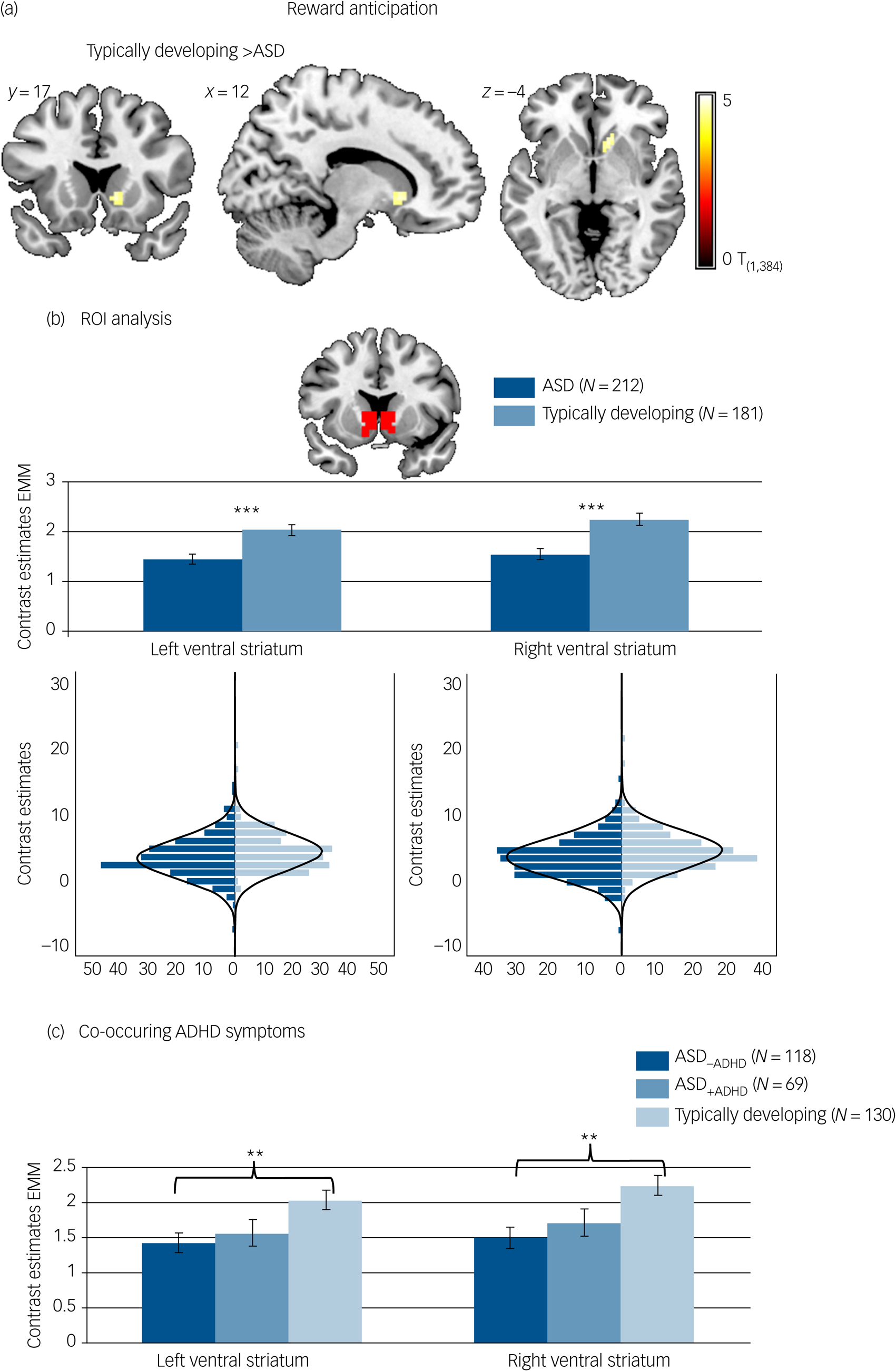
Fig. 2 Brain activation to win compared with neutral cues. (a) Whole-brain family-wise error (FWE) corrected effect of diagnosis in the right ventral striatum. (b) Effect of diagnosis in the region of interest (ROI) analysis of the left and right ventral striatum with corresponding distribution plots. (c) ROI analysis in individuals with autism spectrum disorder (ASD) and elevated attention-deficit hyperactivity disorder (ADHD) symptoms (ASD+ADHD), individuals with ASD without elevated ADHD symptoms (ASD–ADHD) and typically developing individuals without elevated ADHD symptoms. Location and size of ROI mask shown in red. ***P < 0.001, **P < 0.01. Error bars reflect standard error. Whole-brain results thresholded at P FWE < 05. Distributions of ROI activation in case and control participants were compared using the Kolmogorov–Smirnov test, which suggested unequal distributions (left ventral striatum: D (212,181) = 0.156, P = 0.017; right ventral striatum: D (212,181) = 0.193, P = 0.001). EMM, estimated marginal means.
Table 3 Whole-brain and region of interest (ROI) effects of diagnosis on brain activation during reward anticipation and deliverya

ASD, autism spectrum disorder.
a. Table provides test statistic for whole brain and ROI analysis for diagnosis. Voxel-level statistics were family-wise error (FWE) corrected for the number of voxels across the whole brain for each test. Significance was defined as P FWE < 0.05 with a cluster threshold of k ≥ 5. For ROI analysis in the left and right ventral striatum, the critical alpha level was adjusted to P < 0.025 to control for multiple comparisons. Small effect sizes correspond to partial η2 > 0.00995, medium effect sizes correspond to partial η2 > 0.0588 and large effect sizes correspond to partial η2 > 0.1379.
The effect of diagnosis was not significant for differential reward-specific responses between tasks. However, we report differences between ASD and typically developing groups in the SID and MID separately in Supplementary Figure 2 and Supplementary Tables 3 and 4 to allow for a comparison with previous studies. Task effects along with behavioural data are also reported in the Supplementary material.
ROI analysis
Individuals with ASD differed from typically developing individuals on average regarding reward-specific brain activation within the left (F (1,384) = 14.163, P < 0.001, partial η2 = 0.036) and right (F (1,384) = 18.693, P < 0.001, partial η2 = 0.046) ventral striatum ROI (Table 3) with reduced activation in the ASD group (left: mean 1.45, s.d. = 1.53; right: mean 1.54, s.d. = 1.58) compared with the typically developing group (left: mean 2.03, s.d. = 1.53, d = −0.39; right: mean 2.25, s.d. = 1.59, d = −0.44). See Fig. 2(b). There was no significant interaction between diagnosis and task (left ventral striatum: F (1,384) = 2.754, P = 0.098, partial η2 = 0.007; right ventral striatum: F (1,384) = 2.999, P = 0.084, partial η2 = 0.008).
Reward delivery
Whole-brain level analysis
There was no significant effect of diagnostic group on reward-specific brain activation at the whole-brain level. See Fig. 3(a) and Table 3 for details.
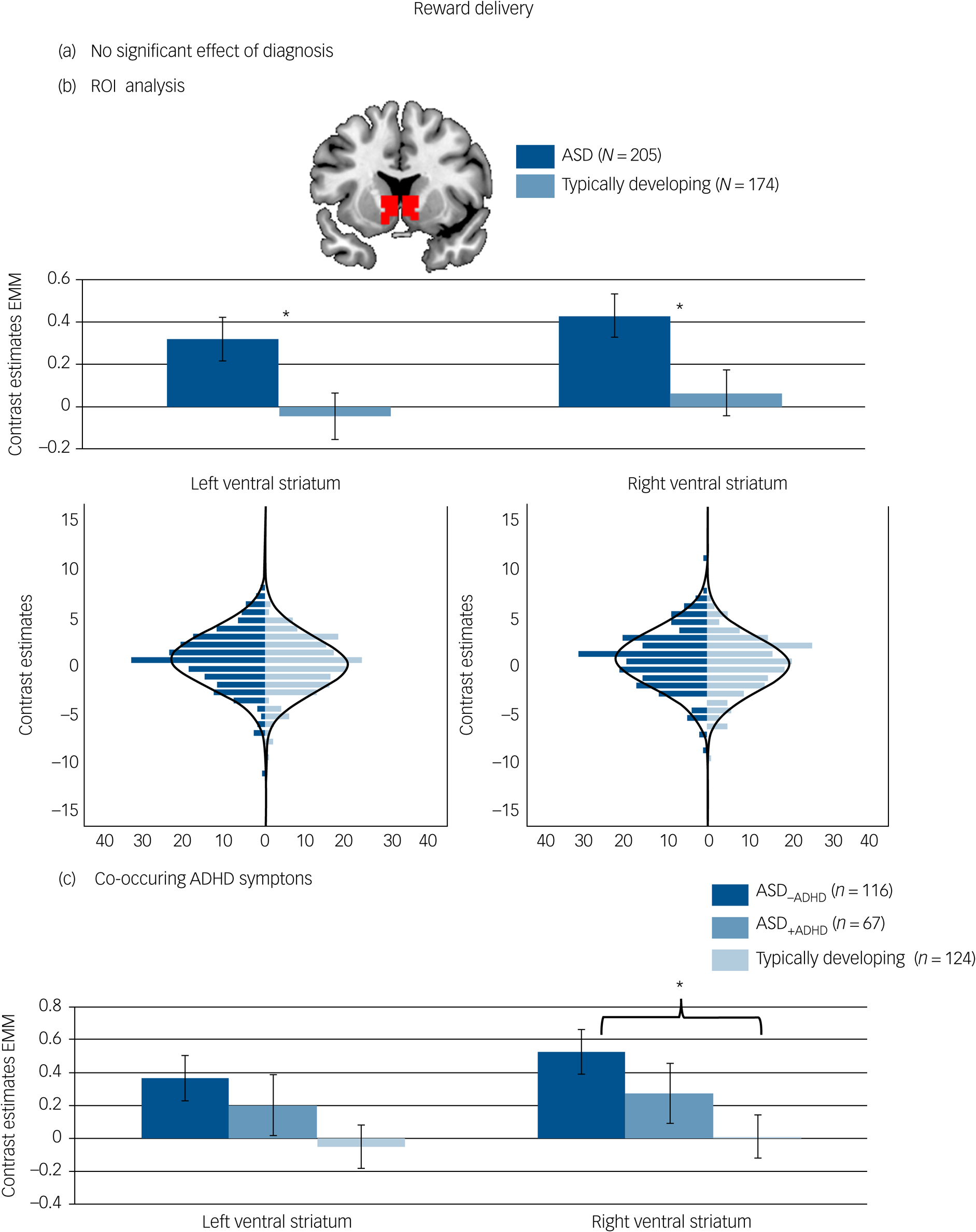
Fig. 3 Brain activation to reward delivery. (a) No significant whole-brain family-wise error (FWE) corrected effect of diagnosis in the right ventral striatum. (b) Effect of diagnosis in the region of interest (ROI) analysis of the left and right ventral striatum with corresponding distribution plots. (c) ROI analysis in individuals with autism spectrum disorder (ASD) and elevated attention-deficit hyperactivity disorder (ADHD) symptoms (ASD+ADHD), individuals with ASD without elevated ADHD symptoms (ASD–ADHD) and typically developing individuals without elevated ADHD symptoms. Location and size of ROI mask shown in red. *P < 0.05. Error bars reflect standard error. Whole-brain results thresholded at P FWE < 05. Distributions of ROI activation in case and control participants were compared using the Kolmogorov–Smirnov test, which suggested no evidence for unequal distributions (left ventral striatum: D (205,174) = 0.120, P = 0.134; right ventral striatum: D (205,174) = 0.112, P = 0.190). EMM, estimated marginal means.
The effect of diagnosis was not significant for differential reward-specific responses between tasks. However, we report differences between the ASD and typically developing group in the SID and MID separately in Supplementary Figure 3 and Supplementary Tables 3 and 4 to allow comparison with previous studies. Task effects along with behavioural data are also reported in the Supplementary material.
ROI analysis
The difference regarding reward-specific brain activation between ASD and typically developing individuals within the left (F (1,370) = 4.829, P = 0.029, partial η2 = 0.013) and right ventral striatum (F (1,370) = 4.719, P = 0.030, partial η2 = 0.013) yielded increased activation in the ASD group (Table 3) (left: mean 0.31, s.d. = 1.46; right: mean 0.42, s.d. = 1.47) compared with the typically developing group (left: mean −0.02, s.d. = 1.46, d = 0.23; right: mean 0.09, s.d. = 1.47, d = 0.23) but this did not survive correction for multiple comparisons (See Fig. 3(b)). There was no significant interaction between diagnosis and task (left ventral striatum: F (1,370) = 1.057, P = 0.304, partial η2 = 0.003; right ventral striatum: F (1,370) = 1.684, P = 0.195, partial η2 = 0.005).
Effect of ADHD co-occurrence
During reward anticipation, ROI analysis comparing the typically developing group, and the ASD–ADHD and ASD+ADHD subgroups yielded a significant effect of group in the left (F (1,307) = 5.172, P = 0.006, partial η2 = 0.032) and right (F (1307) = 6.761, P = 0.001, partial η2 = 0.042) ventral striatum (see Fig. 2(c)). Post hoc pairwise comparisons based on estimated marginal means revealed that this effect was driven by significantly reduced ventral striatum activity in the ASD–ADHD subgroup compared with the typically developing group (left: P = 0.006, d = 0.40; right: P = 0.001, d = 0.46), whereas there was no significant difference between the typically developing group and the ASD+ADHD subgroup (left: P = 0.144, d = 0.30; right: P = 0.099, d = 0.32) or between the two ASD subgroups (left: P = 1.000, d = −0.09; right: P = 1.000, d = −0.13).
For reward delivery, a borderline significant effect of group emerged in the right ventral striatum (F (1,297) = 3.715, P = 0.026, partial η2 = 0.024, see Fig. 3(c)) with significantly increased ventral striatum activity in the ASD–ADHD subgroup compared with the typically developing group (P = 0.020, d = 0.35) and no difference between the typically developing group and the ASD+ADHD subgroup (P = 0.741, d = −0.18) or between the two ASD subgroups (P = 0.810, d = 0.17). Across both reward processing stages, there was no significant effect of group on the whole-brain level and no significant interaction with the type of reward (social, monetary).
Dimensional effects
For both reward anticipation and delivery there was no significant main effect of autism or ADHD trait scores and no interaction between diagnosis and autism or ADHD trait scores in the ventral striatum or at the whole-brain level, see Tables 4 and 5. Autism or ADHD trait scores also showed no significant effect when analysing the typically developing and ASD groups separately.
Table 4 Whole-brain and region of interest (ROI) effects of autism traits on brain activation during reward anticipation and deliverya

SRS-2 Social Responsiveness Scale Second Edition.
a. Table provides test statistic for whole brain and ROI analysis for autism traits. Voxel-level statistics were family-wise error (FWE) corrected for the number of voxels across the whole brain for each test. Significance was defined as P FWE < 0.05 with a cluster threshold of k ≥ 5. For ROI analysis in the left and right ventral striatum, the critical alpha level was adjusted to P < 0.025 to control for multiple comparisons. Small effect sizes correspond to partial η2 > 0.00995, medium effect sizes correspond to partial η2 > 0.0588 and large effect sizes correspond to partial η2 > 0.1379.
Table 5 Whole-brain and region of interest (ROI) effects of attention-deficit hyperactivity disorder (ADHD) traits on brain activation during reward anticipation and deliverya

a. Table provides test statistic for whole brain and ROI analysis for ADHD traits. Voxel-level statistics were family-wise error (FWE) corrected for the number of voxels across the whole brain for each test. Significance was defined as P FWE < 0.05 with a cluster threshold of k ≥ 5. For ROI analysis in the left and right ventral striatum, the critical alpha level was adjusted to P < 0.025 to control for multiple comparisons. Small effect sizes correspond to partial η2 > 0.00995, medium effect sizes correspond to partial η2 > 0.0588 and large effect sizes correspond to partial η2 > 0.1379.
Control analyses
Supplemental control analyses showed that results were not systematically explained by head motion, acquisition site, handedness, sex, IQ or medication status. Effects of age (linear and quadratic) were observed during reward delivery in the right superior medial frontal gyrus and the left amygdala, pallidum and (at trend-level) the ventral striatum, respectively. These effects did not differ between the ASD and typically developing groups. For reward delivery, we were not able to replicate the effect of diagnosis when investigating only female participants, only right-handed participants or when excluding participants from RUNMC or KCL. Although this likely reflects decreased statistical power because of reduced subsample sizes, it also warrants further exploration of potential sources of heterogeneity in future studies. Details on the control analyses are provided in the Supplementary material.
Power sensitivity analyses
Post hoc power sensitivity was determined using G*Power.Reference Faul, Erdfelder, Lang and Buchner26 A repeated measures ANOVA with the within-subject factor task (MID, SID) and between-subject factor diagnosis (typically developing, ASD) and n = 393 participants at a critical alpha of 0.025 would be sensitive to effects of partial η2 = 0.0109 with 80% power. This means the study would not be able to reliably detect effects smaller than partial η2 = 0.0109. The slightly reduced sample size for reward delivery with n = 379 participants yields 80% power at partial η2 = 0.0113 and would thus not be able to reliably detect effects smaller than this.
A repeated measures ANOVA with the within-subject factor task (MID, SID) and between-subject factor diagnosis (typically developing, ASD+ADHD and ASD–ADHD) and n = 317 participants at a critical alpha of 0.025 would be sensitive to effects of partial η2 = .012 with 80% power. This means, the study would not be able to reliably detect effects smaller than partial η2 = 0.012. The slightly reduced sample size for reward delivery with n = 307 participants yields 80% power at partial η2 = 0.0124 and would thus not be able to reliably detect effects smaller than this. These partial η2 = values all represent small effects sizes.
Discussion
Main findings regarding autism
In the present study, we assessed functional brain activation during monetary and social reward anticipation and delivery in a well-powered sample comprising individuals with ASD and typically developing individuals. This allowed us to examine effects of reward type during both reward processing phases. We found a reduction of ventral striatum activity during reward anticipation in individuals with ASD that did not differ between social and monetary rewards. In contrast, during reward delivery, we found that increased ventral striatum activity in the ASD group compared with the typically developing group across both social and monetary reward conditions did not survive correction for multiple comparisons.
These results do not support opposing effects of social and monetary reward types, but rather point towards a general hypoactivity of ventral striatum in ASD during anticipation of rewards. This is in contrast to the hypothesis of a predominantly social motivation deficit.Reference Chevallier, Kohls, Troiani, Brodkin and Schultz1 Instead, in line with two recent meta-analyses,Reference Clements, Zoltowski, Yankowitz, Yerys, Schultz and Herrington4,Reference Janouschek, Chase, Sharkey, Peterson, Camilleri and Abel5 our results suggest atypical reward processing in ASD for both social and monetary rewards. However, only one of the meta-analysesReference Clements, Zoltowski, Yankowitz, Yerys, Schultz and Herrington4 explored reward anticipation and delivery separately, and the pattern of atypicalities we observed differs from those reported there. Although the meta-analysis reports opposing patterns of ventral striatum activation for social and non-social rewards when taking into account the reward processing phase (wanting and liking of rewards), we report the same ventral striatum pattern for monetary and social rewards. We conclude that, in ASD, general hypoactivation during the anticipation of rewards reflects attenuated ‘wanting’ of rewards independent of social content. Our finding is in line with a previous study investigating negative social and monetary reinforcementReference Damiano, Cockrell, Dunlap, Hanna, Miller and Bizzell27 and extends beyond ASD and ADHD to other conditions such as schizophrenia and bipolar disorder,Reference Schwarz, Moessnang, Schweiger, Baumeister, Plichta and Brandeis28 pointing towards a potential shared motivational shift in these conditions that need further investigation.
Our results on reward delivery do not show substantial differences between ASD and typically developing individuals. Although this is in contrast to meta-analytic findingsReference Clements, Zoltowski, Yankowitz, Yerys, Schultz and Herrington4 and previous studies showing striatal hypoactivity during monetary reward deliveryReference Kohls, Schulte-Ruther, Nehrkorn, Muller, Fink and Kamp-Becker10–Reference Dichter, Felder, Green, Rittenberg, Sasson and Bodfish12 it is in line with studies showing no significant group differences during social reward,Reference Dichter, Richey, Rittenberg, Sabatino and Bodfish8,Reference Kohls, Antezana, Mosner, Schultz and Yerys9 monetary rewardReference Dichter, Richey, Rittenberg, Sabatino and Bodfish8 or showing differences only at an uncorrected threshold.Reference Kohls, Thonessen, Bartley, Grossheinrich, Fink and Herpertz-Dahlmann13 In summary, our results suggest that both monetary and social rewards are eliciting reward-related brain activity upon delivery that is not strikingly different in individuals with ASD and typically developing individuals. Behaviourally, individuals with ASD did not differ from typically developing individuals regarding reaction times and accuracy (see Supplementary material), which is in line with previous findings, see for example references Reference Kohls, Antezana, Mosner, Schultz and Yerys9,Reference Kohls, Schulte-Ruther, Nehrkorn, Muller, Fink and Kamp-Becker10,Reference Kohls, Thonessen, Bartley, Grossheinrich, Fink and Herpertz-Dahlmann13,Reference van Dongen, von Rhein, O'Dwyer, Franke, Hartman and Heslenfeld29 .
Interpretation of our findings regarding autism
Incentive stimuli, such as the arrows used in the present study, have gained reward value (wanting) through learning processes by linking them to pleasant outcomes (liking). If individuals with ASD perceived social stimuli as less rewarding,Reference Chevallier, Kohls, Troiani, Brodkin and Schultz1 we would expect both reduced ‘liking’ as well as reduced ‘wanting’ of social rewards in ASD, but no alterations for non-social rewards. The generally reduced activation of the reward system in the ASD group compared with the typically developing group during anticipation of rewards strongly suggest reward processing alterations in autism go beyond social stimuli. The lack of a striking difference between the typically developing and ASD groups for reward delivery indicates that alterations in wanting a reward seem to be more substantial than alterations in liking a reward. This might potentially reflect an altered link between ‘liking’ a reward and ‘wanting’ a subsequent reward, irrespective of reward type, and could suggest generally atypical reinforcement-dependent learningReference Wise30 and/or salience processing in ASD, see for example reference.Reference Uddin, Supekar, Lynch, Khouzam, Phillips and Feinstein31 A hypothesis of generally atypical reward processing in ASD is, however, challenged by studies reporting elevated reward system responsivity in ASD to stimuli of high interest for autistic individuals or to food images.Reference Clements, Zoltowski, Yankowitz, Yerys, Schultz and Herrington4,Reference Kohls, Antezana, Mosner, Schultz and Yerys9,Reference Dichter, Felder, Green, Rittenberg, Sasson and Bodfish12 These findings indicate intact, possibly even hyperactive reinforcement-dependent learning when stimuli with high individual interest are involved. Recent models have tried to address this by proposing an imbalanced response for different reward stimuli.Reference Kohls, Yerys and Schultz2,Reference Tschida and Yerys3 Future work is therefore needed, exploring potential changes in feedback loops underlying altered reinforcement-dependent learning in ASD using connectivity metrics and different reward types, as well as exploring links to atypical salience processing in ASD.
Although significant differences between diagnostic groups were found, we did not observe significant associations between autism trait scores (SRS-2) and functional brain activation across the whole sample or within the ASD and typically developing groups separately. In Supplementary analyses (see Supplementary Table 4) we assessed the effects of autism trait scores for MID and SID separately, but observed no significant effect in this separate analysis. Although others have found associations between dimensional autism measures and reward-related brain activity,Reference Clements, Zoltowski, Yankowitz, Yerys, Schultz and Herrington4,Reference Dichter, Richey, Rittenberg, Sabatino and Bodfish8,Reference Kohls, Thonessen, Bartley, Grossheinrich, Fink and Herpertz-Dahlmann13,Reference van Dongen, von Rhein, O'Dwyer, Franke, Hartman and Heslenfeld29 our results are in line with previous studies also finding no association with dimensional autism measures.Reference Kohls, Schulte-Ruther, Nehrkorn, Muller, Fink and Kamp-Becker10,Reference Dichter, Felder, Green, Rittenberg, Sasson and Bodfish12 Previous studies argued that their null findings might be because of insufficient power and insufficient range of scores in the ASD group,Reference Kohls, Schulte-Ruther, Nehrkorn, Muller, Fink and Kamp-Becker10,Reference Dichter, Felder, Green, Rittenberg, Sasson and Bodfish12 which is less of a concern in the present findings. Recent electrophysiological findings might point towards a diverging trajectory of building reward anticipation for high versus low autistic traits, which might mask associations with autistic traits based on the time point when reward anticipation is measured.Reference Matyjek, Bayer and Dziobek32
Main findings regarding co-occurring ADHD
Elevated ADHD symptoms did not have an additive effect on reward system atypicality in ASD, in contrast to our hypothesis. Dimensional analysis of ADHD symptoms yielded no significant results. During reward anticipation, post hoc pairwise comparisons revealed that ventral striatum activity was reduced only in those individuals with ASD that had subthreshold levels of ADHD (ASD–ADHD) compared with typically developing participants, whereas those individuals with ASD that had elevated ADHD levels (ASD+ADHD,) did not differ significantly from the typically developing group or the ASD–ADHD subgroup. During reward delivery, differences between the three groups were not strong enough to reach statistical significance when correcting for multiple comparisons. However, the direction of the effect also suggested the largest deviation for the ASD–ADHD group. These results support an alternative hypothesis of ADHD symptoms partly countering ASD-related motivational atypicalities. This would be in line with a previous finding, where individuals with ASD showed generally low ventral striatum reactivity, and individuals with ADHD showed high ventral striatum reactivity to both social and monetary reward types.Reference Kohls, Thonessen, Bartley, Grossheinrich, Fink and Herpertz-Dahlmann13 However, this study did not differentiate between reward anticipation and delivery.
Interpretation of our findings regarding co-occurring ADHD
Although during monetary reward anticipation, ventral striatum hypoactivation is discussed as a fairly consistent finding in adults and adolescents with ADHD (see reference Reference Plichta and Scheres14, but see also reference Reference von Rhein, Cools, Zwiers, van der Schaaf, Franke and Luman33), findings are more inconsistent in children (for example see reference Reference van Hulst, de Zeeuw, Bos, Rijks, Neggers and Durston34). For monetary reward delivery, increased ventral striatum activity in ADHD has been reported (see for example references Reference von Rhein, Cools, Zwiers, van der Schaaf, Franke and Luman33,Reference Paloyelis, Mehta, Faraone, Asherson and Kuntsi35,Reference Furukawa, Bado, Tripp, Mattos, Wickens and Bramati36 but see also reference Reference van Dongen, von Rhein, O'Dwyer, Franke, Hartman and Heslenfeld29). Importantly, information on social reward processing in ADHD is scarce. To our knowledge, the present study is the first to address co-occurring ADHD symptoms during reward anticipation and delivery separately. Instead of an additive effect of previously described atypicalities in these two conditions, our findings suggest ASD with co-occurring ADHD might represent a subgroup of individuals that, on average, shows no statistically distinct neuronal deviation from typically developing individuals nor those with ASD. This is especially important in light of the ongoing search for biomarkers underlying these neurodevelopmental conditions. It further refutes a potential concern that reward system alterations in ASD could, in truth, be driven by co-occurring ADHD. However, information on the presence of a confirmed diagnosis of ADHD was not available in our sample, and a questionnaire-derived proxy was used instead. This might have a significant impact on our findings, as ADHD-like behaviours might have been misclassified. Consequently, our finding requires further investigation using clinically confirmed information on ASD–ADHD co-occurrence.
Limitations
Although the present study provides important insights into group-level on-average reward processing alterations in autism, a number of limitations need to be considered. First, although our findings of differences in reward processing between the ASD and typically developing groups were significant for the anticipatory phase, effect sizes were small. This likely reflects substantial between-subject heterogeneity partly attributable to the multicentre design of the study and to the intention of collecting a representative data-set reflecting the heterogeneity of ASD. We aim to further explore this heterogeneity within the LEAP sample using classification and stratification approaches in future studies.
Second, the task design did not allow for a clear separation between feedback presentation and motor response (short interstimulus interval, no jitter). Thus, we cannot rule out that findings in the delivery phase were influenced by motor activity. Third, we used static images for social reward, which likely reduced ecological validity. Fourth, task order was fixed across all participants. Thus, task order might have had an impact on the effect of stronger differential brain activation in the MID compared with the SID task. Finally, although incentive delay tasks are widely used to study reward processing, they do not allow us to pinpoint brain activity in relation to just one reward-processing aspect, but comprise multiple additional subconstructs such as reinforcement-based learning, reward valuation and reward prediction.
Implications
In summary, the present study demonstrates a significant reduction of ventral striatum activity during the anticipation of rewards in individuals with ASD irrespective of the type of reward, and subthreshold hyperactivity of ventral striatum during the delivery of these rewards. In contrast to our hypothesis, altered reward processing was not exacerbated by elevated ADHD symptoms. This might suggest generally atypical reward processing in ASD that is partly countered by co-occurring ADHD. This provides important insights, specifically as the impact of co-occurring ADHD has not been consistently assessed in previous studies on reward processing alterations in ASD and might contribute towards the heterogeneity of findings. It might further explain discrepancies between our findings and the two meta-analyses,Reference Clements, Zoltowski, Yankowitz, Yerys, Schultz and Herrington4,Reference Janouschek, Chase, Sharkey, Peterson, Camilleri and Abel5 given the similar sample size while both meta-analyses did not address ADHD symptoms. Although further exploration of the underlying mechanisms is needed, the present study advances our understanding of the neural underpinnings of ASD by suggesting attenuated reward seeking independent of social content that is partly countered by co-occurring ADHD.
Future studies on reward processing, especially on reward anticipation, in ASD should thus collect and report data on ADHD symptoms and diagnosis effects. The current study presents just one step towards a broader and deeper understanding of reward processing alterations in autism, which should ultimately improve services for autistic individuals, for example by establishing alternatives to reinforcement-based interventions where needed or by including personalised rewards as alternatives to generic rewards when employing established reinforcement-based interventions.
Supplementary material
To view supplementary material for this article, please visit https://doi.org/10.1192/bjp.2022.157
Data availability
The data that support the findings of this study are currently not publicly available due to an embargo period but are available from the corresponding author upon reasonable request.
Acknowledgements
We gratefully acknowledge Dr Will Spooren’s invaluable contribution to the study design and editing of the original manuscript. The manuscript has since been through revisions which Dr Spooren was unable to approve, along with this final version which has been accepted for publication. Thus, Dr Spooren unfortunately does not fulfil the requirements for authorship. A prior version of present manuscript has been uploaded as a preprint to www.biorxiv.org. We gratefully acknowledge the contributions of the EU-AIMS LEAP Group: Jumana Ahmad, Sara Ambrosino, Bonnie Auyeung, Tobias Banaschewski, Simon Baron-Cohen, Sarah Baumeister, Christian F. Beckmann, Sven Bölte, Thomas Bourgeron, Carsten Bours, Michael Brammer, Daniel Brandeis, Claudia Brogna, Yvette de Bruijn, Jan K. Buitelaar, Bhismadev Chakrabarti, Tony Charman, Mario Cirillo, Ineke Cornelissen, Daisy Crawley, Flavio Dell'Acqua, Francesco Di Salle, Guillaume Dumas, Sarah Durston, Christine Ecker, Fabrizio Esposito, Jessica Faulkner, Vincent Frouin, Pilar Garcés, David Goyard, Lindsay Ham, Hannah Hayward, Joerg Hipp, Rosemary Holt, Mark H. Johnson, Emily J.H. Jones, Prantik Kundu, Meng-Chuan Lai, Xavier Liogier D'ardhuy, Michael V. Lombardo, Eva Loth, David J. Lythgoe, René Mandl, Andre Marquand, Luke Mason, Maarten Mennes, Andreas Meyer-Lindenberg, Carolin Moessnang, Nico Mueller, Declan G.M. Murphy, Bethany Oakley, Laurence O'Dwyer, Marianne Oldehinkel, Bob Oranje, Gahan Pandina, Antonio M. Persico, Barbara Ruggeri, Annika Rausch, Amber Ruigrok, Jessica Sabet, Roberto Sacco, Antonia San José Cáceres, Emily Simonoff, Will Spooren, Giacchino Tedeschi, Julian Tillmann, Roberto Toro, Heike Tost, Jack Waldman, Steve C.R. Williams, Caroline Wooldridge, and Marcel P. Zwiers. The primary contact for the EU-AIMS LEAP Group is Declan G. Murphy (email: [email protected]).
Author contributions
T.C., S.B.C., C.B., S.Bö., T.Bo., F.D.A., S.D., C.E., M.H.J., E.J.H.J., M.V.L., L.M., E.L., D.G.M.M., J.K.B., H.T., A.M.L., T.Ba., D.B. designed the study. S.Ba., N.B., S.H., J.T., D.G., S.A., A.R., D.C., V.F., H.H., R.H., A.S.J.C. participated in data acquisition. J.T., D.G., T.C., S.B.C., S.Bö., T.Bo., F.D.A., G.D., S.D., C.E., D.L.F., V.F., M.H.J., E.J.H.J., M.C.L., M.V.L., L.M., B.O., M.O., A.M.P., T.W., E.L., D.G.M.M., J.K.B., T.Ba., D.B. supervised data acquisition and/or performed quality control. S.Ba. performed data analyses. C.M. assisted with data analyses. S.Ba., C.M., P.A., A.K., E.L., D.G.M.M., J.K.B., H.T., A.M.L., T.Ba., D.B. interpreted the findings. S.Ba. wrote the manuscript. C.M., N.B., P.A., A.K., T.C., S.A., S.Bö., F.D.A., G.D., S.D., C.E., D.L.F., H.H., R.H., E.J.H.J., M.C.L., M.V.L., L.M., B.O., M.O., A.S.J.C., T.W., E.L., D.G.M.M., J.K.B., H.T., A.M.L., T.Ba., D.B. critically edited the manuscript. All authors approved the manuscript and agree to ensure that questions regarding the accuracy or integrity of any part of the work are appropriately investigated and resolved.
Funding
This work was supported by EU-AIMS (European Autism Interventions), which received support from the Innovative Medicines Initiative Joint Undertaking under grant agreement no. 115300, the resources of which are composed of financial contributions from the European Union’ s Seventh Framework Programme (grant FP7/2007-2013), from the European Federation of Pharmaceutical Industries and Associations companies’ in-kind contributions, and from Autism Speaks. The results leading to this publication have received funding from the Innovative Medicines Initiative 2 Joint Undertaking under grant agreement No 777394 for the project AIMS-2-TRIALS. This Joint Undertaking receives support from the European Union's Horizon 2020 research and innovation programme and EFPIA and AUTISM SPEAKS, Autistica, SFARI. The funders had no role in the design of the study; in the collection, analyses, or interpretation of data; in the writing of the manuscript, or in the decision to publish the results. Any views expressed are those of the author(s) and not necessarily those of the funders.
Declaration of interest
A.M.L. has received consultant fees from Agence Nationale de la Recherche, Catania International Summer School of Neuroscience (CISSN), Daimler und Benz Stiftung, EPFL Brain Mind Institute, Fondation FondaMental, Hector Stiftung II, Invisio, Janssen-Cilag GmbH, Lundbeck A/S, Lundbeckfonden, Lundbeck Int. Neuroscience Foundation, MedinCell, Sage Therapeutics, Techspert.io, The LOOP Zürich, University Medical Center Utrecht, University of Washington, von Behring Röntgen Stiftung. He has received speaker fees from Ärztekammer Nordrhein, BAG Psychiatrie Oberbayern, Biotest AG, Forum Werkstatt Karlsruhe, International Society of Psychiatric Genetics, Brentwood, Klinik für Psychiatrie und Psychotherapie Ingolstadt, Lundbeck SAS France, med Update GmbH, Merz-Stiftung, Siemens Healthineers, Society of Biological Psychiatry. A.M.L. has received editorial fees from American Association for the Advancement of Science, Elsevier, ECNP, Thieme Verlag and author fees from Thieme Verlag. D.M. has served on advisory boards for Roche and Servier, and has received research grants from Roche and J&J. W.M. has received lecture or travel fees from Pfizer, Grünenthal, University of Zürich, International Association for the Study on Pain (IASP) and European Federation of IASP Chapters (EFIC). S.B. discloses that he has in the past 3 years acted as an author, consultant or lecturer for Medice and Roche. He receives royalties for textbooks and diagnostic tools from Huber/Hogrefe (German/Swedish versions of ADI-R, ADOS-2, SRS, SCQ), Kohlhammer and UTB. T.B. served in an advisory or consultancy role for ADHS digital, Infectopharm, Lundbeck, Medice, Neurim Pharmaceuticals, Oberberg GmbH, Roche, and Takeda. He received conference support or speaker's fee by Medice and Takeda. He received royalities from Hogrefe, Kohlhammer, CIP Medien, Oxford University Press. D.B. served as an unpaid scientific advisor for an EU-funded ADHD neurofeedback trial. A.S.J.C. received consultant fees and participated in several advisory boards from Roche and Servier. T.C. has received consultancy fees from Roche and Servier and royalties from SAGE Publications and Guilford Publications. J.K.B. has been in the past 3 years a consultant to/member of advisory board of/and/or speaker for Takeda/Shire, Roche, Medice, Angelini, Janssen, and Servier. He is not an employee of any of these companies, and not a stock shareholder of any of these companies. He has no other financial or material support, including expert testimony, patents, royalties. D.L.F. is supported by funding from the European Union's Horizon 2020 research and innovation programme under the Marie Skłodowska-Curie grant agreement No 101025785. All other authors report no potential conflict of interest. The present work is unrelated to the above grants and relationships.
eLetters
No eLetters have been published for this article.