Introduction
Nematodes have evolved several different lifestyles. Plant-parasitic nematodes have been responsible for $US80 billion in annual economic losses of agricultural crops (Jones et al. Reference Jones, Haegeman, Danchin, Gaur, Helder, Jones, ikuchi, Manzanilla-López, Palomares-Rius, Wesemael and Perry2013); free-living C. elegans is a popular model organism; animal parasitic nematode poses a significant risk to the safety of domestic animals and human health (Colella et al. Reference Colella, Bradbury and Traub2021; Zajac & Garza. Reference Zajac and Garza2020). Free-living nematodes including fungivorous, bacteriovorous, predatory, and omnivorous nematodes play an important role in the soil ecosystem (Bardgett & van der Putten Reference Bardgett and van der Putten2014). Relative to parasitic species, very little is known about free-living nematodes, especially with respect to their genome and transcriptome (Viney Reference Viney2017). This limited information hampers our understanding of their functional biology and the genetic mechanisms that drive their parasitic evolution.
The species belonging to the genus Cruznema are free-living bacterivores that contribute to nitrogen mineralization by consumption of bacteria and excretion of excess nitrogen (Ferris et al. Reference Ferris, Venette and Lau1997). Phylogenetically, this genus is closely related to C. elegans (Du et al. Reference Du, Guo, Gao, Wang, Qing and Li2022). In respect to soil ecology, they play a major role in the cave’s food web, which can be an indication of soil quality (Lau et al. Reference Lau, Fuller, Ferris, Venette and Scow1997). Among them, C. tripartitum (von Linstow, Reference Von Linstow1906) Sudhaus, Reference Sudhaus1974 is the most common species, primarily isolated from soil associated with putrid vegetal tissue and rotten meat, but also found in the larvae of some insects and slugs (Doucet Reference Doucet1994, Grewal et al. Reference Grewal, Grewal, Tan and Adams2003).
In this study, a population of genus Cruznema Artigas, 1927, which was initially identified as C. tripartitum (Du et al. Reference Du, Guo, Gao, Wang, Qing and Li2022), was characterised as C. velatum Brzeski, Reference Brzeski1989 by morphological and molecular data. Biological characters including generation time, reproduction type, and fecundity were also studied. Apart from species description, we conducted the first RNA-seq of this species. The obtained transcriptome was used for gene prediction and their putative functions were annotated.
Materials and methods
Morphological and biological characterization
The nematodes were extracted from soil samples using the modified Baermann tray method (Whitehead & Hemming Reference Whitehead and Hemming1965). A gravid female packed with eggs was placed on a nematode growth medium (NGM) plate for pure culture, using the method described by Ferris et al. (Reference Ferris, Venette and Lau1997). One milliliter of Escherichia coli strain OP50 with an OD value of 0.6–0.8 was inoculated using the NGM plate as a food source and subsequently incubated at 20°C for 10 days.
Fresh nematodes were killed by adding 4% formaldehyde solution at 85°C, and permanent slides were made following the method described by Sohlenius & Sandor (Reference Sohlenius and Sandor1987). Microphotographs and measurements were made based on permanent slides using an Olympus BX51 microscope equipped with an Olympus DP72 camera (Olympus Corporation, Tokyo, Japan).
To study the life cycle of C. velatum, a single egg was put on the NGM plate (4 cm diameter) supplied with E. coli strain OP50. Twenty replicates were set, and the egg-to-female/male time was recorded. The reproduction pattern was examined by inoculating one young female and male on the NGM plate incubated at 20°C, and the number of offspring was counted every 24 h. A single young female was also inoculated to examine if they can reproduce by parthenogenesis. All inoculations had ten replicates.
DNA extraction and genome sequencing
Approximately 12,000 mixed-staged nematodes were collected from NGM medium using the Baermann funnel. The nematodes were processed by freezing-thawing to break cuticles for three repeats. They were subsequently extracted for DNA using the Ezup Column Animal Genomic DNA Purification Kit (Sangon Biotech, Shanghai, China). The quantity and quality of extracted DNA were checked using the Qubit® 1x dsDNA HS Kit (Yeasen Biotech, Shanghai, China). A genomic library was constructed using an Illumina TruSeq DNA Sample Preparation Kit, and 2×150 bp pair-ends sequencing was performed on the Illumina NovaSeq platform (Personalbio, Shanghai, China).
Sequence assembly and extraction of nematode barcoding genes
Raw reads were quality filtered by fastp (Chen et al. Reference Chen, Zhou, Chen and Gu2018) and FastQC (https://www.bioinformatics.babraham.ac.uk/projects/fastqc/). Ribosomal and mitochondrial COI contigs were extracted from high quality reads through NextGenMap (Sedlazeck et al. Reference Sedlazeck, Rescheneder and von Haeseler2013) mapping against the references (rRNA sequences: JN636136, JN636139, and JN636138; mitochondrial genome: EF043402, MH104864, MH119603, and MH119604), then formatted by SAMtools (Li et al. Reference Li, Handsaker, Wysoker, Fennell, Ruan, Homer, Marth, Abecasis and Durbin2009), and assembled by NOVOPlasty (Dierckxsens et al. Reference Dierckxsens, Mardulyn and Smits2017). The resulting contigs were mapped to the references mentioned above to locate ITS, 18S rRNA, 28S rRNA, and the mitochondrial COI region by using Geneious v 7.13 (Biomatters Ltd, Auckland, New Zealand).
To analyse intraspecific molecular variability, the near full length of 18S rRNA genes were amplified with primer pair SSU18A (5’-AAA GAT TAA GCC ATG CAT G-3’) and SSU26R (5’-CAT TCT TGG CAA ATG CTT TCG-3’) (Blaxter et al. Reference Blaxter, De Ley, Garey, Liu, Scheldeman, Vierstraete, Vanfleteren, Mackey, Dorris, Frisse, Vida and Thomas1998); the 28S was amplified with D2A (5’-ACA AGT ACC GTG AGG GAA AGT TG-3’) and D3B (5’-TCC TCG GAA GGA ACC AGC TAC TA-3’) (De Ley et al. Reference De Ley, Félix, Frisse, Nadler, Sternberg and Thomas1999). The DNA template was extracted from a single individual nematode using the worm lysis buffer described by Singh et al. (Reference Singh, Couvreur, Decraemer and Bert2019). The reaction system was 2 μl of each primer, 7.5 μl double-distilled water, 12.5 μl of Ex Taq DNA polymerase mix (Bioscience, Shanghai, China), and 1 μl of DNA template. The thermal cycle program started at 95°C for 4 min, followed by 35 cycles of 95°C for 30 s, 54°C for 30 s, 72°C for 1 min, and finished at 72°C for 10 min. The amplified products were purified and subsequently sent for sequencing in the Sangon Corporation (Sangon Biotech, Shanghai, China).
Phylogenetic analysis based on barcoding genes
The four barcode genes were subjected to a Basic Local Alignment Search Tool (BLAST) search to check for closely related species in GenBank. DNA sequences retrieved from the database were aligned by using MAFFT v. 7.205 with the G-INS-i algorithm (Katoh & Stanley Reference Katoh and Standley2013), except the COI gene was aligned using TranslatorX (Abascal et al. Reference Abascal, Zardoya and Telford2010) under the invertebrate mitochondrial genetic code. Phylogenetic analyses of the sequence data sets were performed with MrBayes 3.2.7 (Ronquist et al. Reference Ronquist, Teslenko, van der Mark, Ayres, Darling, Höhna, Larget, Liu, Suchard and Huelsenbeck2012) and RAxML8.1.12 (Stamatakis et al. Reference Stamatakis, Hoover and Rougemont2008) on the CIPRES Science Gateway (Miller et al. Reference Miller, Pfeiffer and Schwartz2010). Bayesian inference (BI) analysis was performed using the GTR + I + G evolutionary model. The Markov chains were set with 1 × 106 generations, 4 runs, 25% burn-in, and sampling frequency was 100 generations. Maximum-likelihood (ML) analysis was conducted with 1,000 bootstrap (BS) replicates under the GTRCAT model. Trees were visualized and polished by using FigTree v. 1.4.3 (Rambaut Reference Rambaut2016) and Adobe Illustrator 2020 (Adobe, California, USA).
RNA extraction and transcriptomic analysis
To investigate the molecular basis of Cruznema, high quality RNA was extracted from approximately 30,000 individuals using TRIzol (Invitrogen, Carlsbad, CA, USA), subsequently fragmented and generated to first strand cDNA using random hexamer primers (Invitrogen, Carlsbad, CA, USA), and finally synthesized the double strand cDNA with the cDNA Synthesis System (Roche, Indianapolis, IN, USA). The cDNA was purified, terminally repaired, and poly A-tailed to connect the adaptor for the construction of the RNA-seq library and 2×150 bp paired-end sequencing on the Illumina HiSeq platform according to the manufacturer’s instructions (Grandomics, Wuhan, China). The raw RNAseq data was submitted to the NCBI Sequence Read Archive database with the accession number SRR23934717.
Clean reads produced from the raw data by fastp and FastQC were de novo assembled, filtered, and redundancy removed to create unigenes for subsequent analysis following the Trinity pipeline (Grabherr et al. Reference Grabherr, Haas, Yassour, Levin, Thompson, Amit, Adiconis, Fan, Raychowdhury, Zeng, Chen, Mauceli, Hacohen, Gnirke, Rhind, di Palma, Birren, Nusbaum, Lindblad-Toh, Friedman and Regev2011). The coding sequences and protein sequences of unigenes were determined using a transdecoder (https://github.com/TransDecoder/TransDecoder). Unigenes were aligned against publicly accessible databases including the NCBI Non-redundant protein sequence database (NR, ftp.ncbi.nlm.nih.gov/blast/db/FASTA/nr.gz), Eukaryotic orthologous groups of proteins (KOG, http://www.ncbi.nlm.nih.gov/COG/), SwissProt databases, gene ontology (GO, http://www.geneontology.org/), and the Kyoto encyclopedia of genes and genomes (KEGG, http://www.genome.jp/kegg/) for function annotation, by using BLAST 2.2.29+ (McGinnis & Madden Reference McGinnis and Madden2004) with E-value 10-5.
Results
Redescription of Cruznema velatum
Measurements, see Table 1. Morphological characters, see Figures 1–3
Table 1. Morphometrics of Cruznema velatum. All measurements are in μm and in the form: mean ± sd (range)

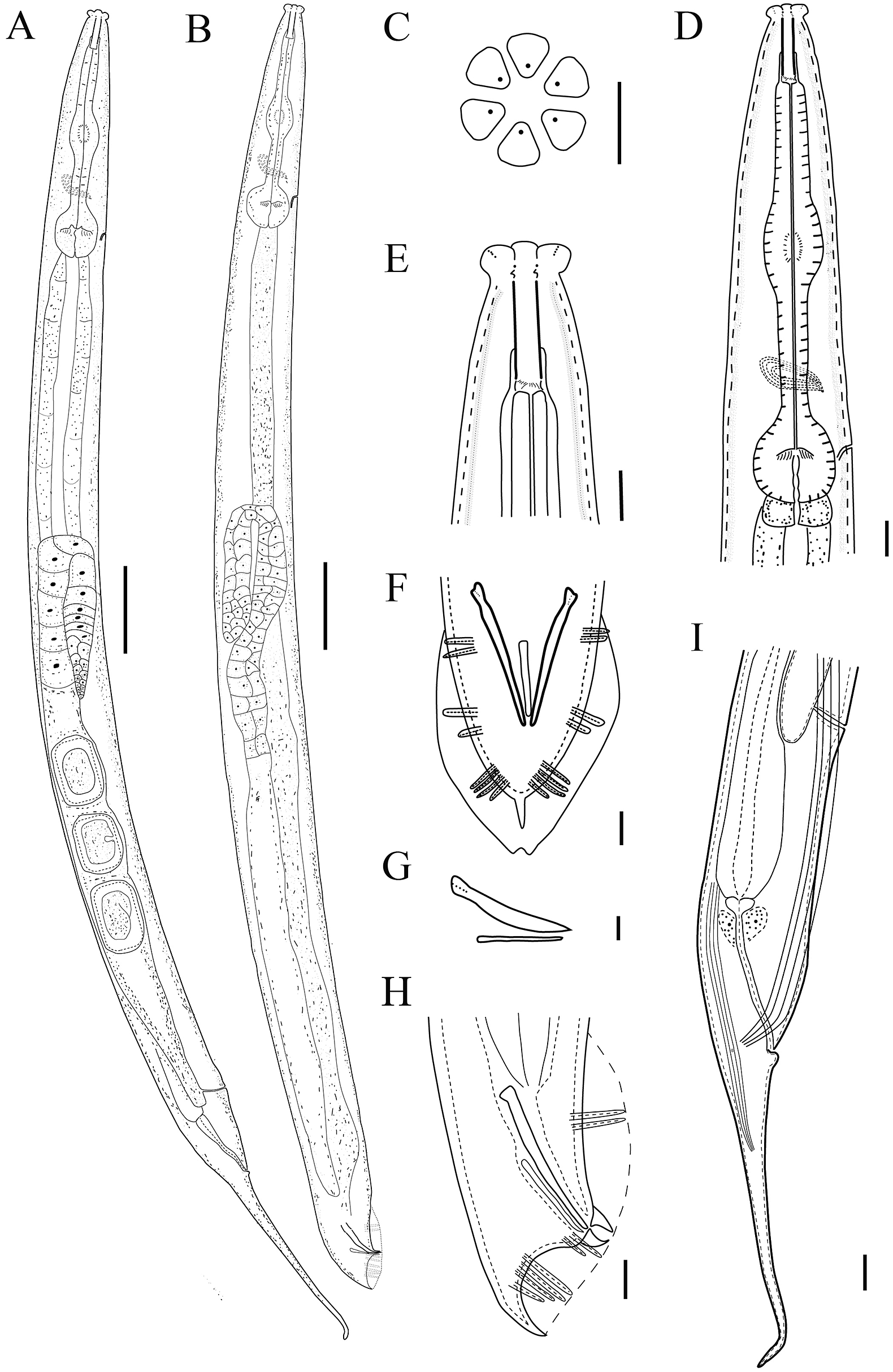
Figure 1. Line drawing of Cruznema velatum. A: Entire female; B: Entire male; C: En-face view; D: Female pharyngeal region; E: Female head region; F: Male tail region in ventral view; G: Spicule and gubernaculum; H: Male tail region in lateral view; I: Female posterior body region showing four lateral lines (Scale bars: A, B = 50 μm; C–H = 10 μm).

Figure 2. Light micrographs of Cruznema velatum. A: Female entire body; B: Male entire body; C: Cuticle annulation; D: Female anterior body region; E: Female lateral lines; F–H: Female head region; I, J: Female vulval region; K: Female anal region; L: Female posterior body region (Scale bars: A, B = 100 μm; C, E–K = 10 μm; D = 40 μm; L = 50 μm).

Figure 3. Light micrographs of Cruznema velatum female and male tail region. A–C: Cuticle thickening around anus; D: Lateral lines at posterior end; E, F: Vulva-anal region; G, H: Male tail region in lateral view; I: Spicules and gubernaculum; J, K: Male tail region in ventral view; L: Spicule and gubernaculum in ventral view; Abbreviation: a = anus; l = lateral lines; v = vulva (Scale bars: A–L = 10 μm).
Cruznema velatum Brzeski (Reference Brzeski1989) = C. tripartitum (Du et al. Reference Du, Guo, Gao, Wang, Qing and Li2022)
Female: Body slightly curved when heat killed, 1.0–1.4 mm long, tapering at both extremities. Cuticle with punctations formed by distinct transverse annulations and longitudinal lines. Lateral field with four smoothly equidistant incisures, extending posteriorly to anus ca. 29.3–39.4% of tail length. Labial area truncated, six lips globular and separated by deep grooves. Stoma prismatic, long, narrow, ca 3.5–5.5 times longer than wide. Amphidial apertures, pore-like, on lateral lips. Pharyngeal sleeve surrounding ca. 30.0–35.0% of stoma. Pharynx comprised moderately-swollen 132.0–155.0 μm long corpus, 32.0–50.0 μm long isthmus, and rounded to pyriform basal bulb with small cardia. Nerve ring encircling isthmus at ca. 60–78% of pharyngeal length. Secretory-excretory pore located at level posterior to nerve ring, ca. 80–95% of pharyngeal length. Reproductive system mono-prodelphic, reflexed part of ovary 110.5–173.5 μm long. Oocytes arranged in two rows at distal end of ovary followed by a single row. Spermatheca indistinct. Uterus large, with well-developed glandular and muscular parts, mostly holding 2 to 22 embryonating eggs, sometimes egg segmentation starting in uterus. Vulva close to anus, with a transverse slit. Vulval cuticular membranes formed by lateral field. The posterior uterine sac very short, ca. 14.0–18.0 μm long. Phasmid pore-like, located at level of anus. Cuticle beneath anus thickening to form a rounded ball. Tail extended into a fine filament, ca. 3.5–7.5 times length of body width at anus.
Male: Body almost straight when heat-killed. Morphology of anterior body part similar to that of females. Testis reflexed, reflexed part ca. 87.5–148.0 μm long. Spicules free, tubular, straight, anteriorly cephalated, posteriorly not fused, about 43.0–56.5 μm long. Gubernaculum tapered to a point at the proximal end, 29.5–37.0 μm long. Bursa peloderan with an oval outline in ventral view, well-developed. Genital papillae (GP) eight pairs in 2 + 2 + 4 configuration, with GP1 and GP2 just anterior to gubernaculum head, GP3 and GP4 closely located at level of cloacal aperture, and GP5, GP6, GP7, and GP8 usually formed a group. Phasmid similar to females, located at middle of spicules. Cloacal lips projected.
Locality: Type population of C. velatum recovered from soil collected from a chestnut orchard at Guangdong Province, China (GPS coordinates: 23°56’44"N, 114°42’15"E).
Diagnosis and relationships
C. velatum is a gonochoristic species, characterized by a lateral field with four incisures extending to ca. 29.3–39.4% of tail length; phasmid located at the middle of lateral lines at level of anus, lower anal lip prolapsed, filamentous tail ca. 3.5–7.5 times lower anal lip prolapsed, and peloderan bursa having eight pairs (2 + 2 + 4) of genital papillae.
The genus Cruznema contains seven valid species. C. velatum differs from C. campestre Reboredo & Camino, Reference Reboredo and Camino2000 by the female tail shape (filamentous vs. conical), male body length (1.0–1.4 vs. 0.7–0.9 mm), gubernaculum length (29.5–37.0 vs. 21.1–23.5 μm), and number of genital papillae (8 pairs vs. 9 pairs); from C. graciliformis (Goffart, Reference Goffart1935) Sudhaus, Reference Sudhaus1978 by the female tail shape (filamentous vs. conical), female body length (1.0–1.4 vs. 0.8–1.0 mm), spicule length (43.0–56.5 vs. 24.0–29.0 μm), and gubernaculum length (29.5–37.0 vs. 13.0–15.0 μm); from C. helalii Tahseen, Sultana, Khan & Hussain, Reference Tahseen, Sultana, Khan and Hussain2012 by the post uterine sac (present vs. absent), lower anal lip prolapse (present vs. absent), and V value (72.5–82.5 vs. 85.2–90.1%); from C. lincolnense Reboredo & Camino, Reference Reboredo and Camino1998 by the female tail shape (filamentous vs. conical), male body length (1.0–1.4 vs. 0.8–1.0 mm), spicule length (43.0–56.5 vs. 37.6–44.7 μm), gubernaculum length (29.5–37.0 vs. 17.6–23.5 μm), and number of genital papillae (8 pairs vs. 9 pairs); from C. minimus Sultana & Pervez, Reference Sultana and Pervez2019 by the female body length (1.0–1.4 vs. 0.5–0.6 mm), a value (16.0–22.4 vs. 10.3–13.9) and b value (5.0–6.0 vs. 10.3–13.9) of female, cuticle protruding beneath anus (present vs. absent), spicule length (43.0–56.5 vs. 19–23 μm), and gubernaculum (29.4–37.2 vs. 17–18 μm); from C. scarabaeum (Sudhaus, Reference Sudhaus1978) Andrássy, Reference Andrássy1983 by the cuticlular punctations (present vs. absent), b value of female (5.0–6.0 vs. 6.8–6.9), spicule length (43.0–56.5 vs. 61.0–72.0 μm), and genital papillae arrangement (2 + 2 + 4 vs. 2/1 + 4 + 3); from C. tripartitum by the female body length (1.0–1.4 vs. 0.9–2.2 mm), vulval cuticular flaps (present vs. absent), number of eggs in uterus (2–21 vs. up to 50), and gubernaculum length (29.5–37.0 vs. 18–25 μm).
Biological characters
C. velatum took 7–9 days to develop from egg to egg-laying adult at 20°C, and each stage can be easily differentiated by the length of body and gonad (Figure 4). A single gravid female can oviposit 85–131 eggs. The lifespan is about 11 to 14 days. A single female without male fertilization cannot produce any eggs, which indicates the species is amphimix.

Figure 4. Light micrographs for development stages of Cruznema velatum. A: Egg; B: Second-stage juvenile; C: Third-stage juvenile; D: Fourth-stage juvenile; The somatic gonad priordium is indicated by arrow (Scale bars: A = 10 μm; B–D = 50 μm).
Molecular characterization and phylogeny
The sequences of four barcode genes were extracted from the assembly of complete sequences of rRNA and mitochondrial genome of C. velatum, with GenBank accession number ON191470 (1,722 bp) for the 18S rRNA gene, ON191476 (952 bp) for the D2–D3 domain of the 28S rRNA gene, ON191475 (1,057 bp) for the ITS region, and ON190029 (758 bp) for the partial mtCOI gene. The additional sequences of 18S and 28S rRNA genes were obtained from single individuals of the species, with GenBank accession numbers ON191471–ON191474 (867–938 bp) for the 18S rRNA gene and ON191477–ON191479 (609–613 bp) for the 28S rRNA gene.
The phylogenetic relationships of the species with other related species were analysed based on four barcoding genes. The 18S phylogenetic tree (Figure 5) revealed that all five representatives of C. velatum clustered in single branch, forming a monophyletic clade (PP = 1, BS = 100) together with two C. tripartitum populations (EU196012, U73449) and five unidentified Cruznema populations (AY284655–AY284658, MG551688). The Cruznema clade is sistered to two populations of Cephaloboides nidrosiensis (KY119777, EU196020). Nucleotides differences in five 18S sequences of the species were 1–2 bp (99.8–100% identities). The species (GD-1, ON191470) clearly differs from C. nidrosiensis (KY119777) by 174 nucleotides (80.4% identity) and from C. nidrosiensis (EU196020) by 244 nucleotides (85.4% identity).

Figure 5. Bayesian 50% majority-rule consensus tree of Cruznema velatum and other related nematodes inferred from 18S rRNA gene. Dataset aligned with G-INS-I implemented in MAFFT. The values at clade node indicate posterior probability/bootstrap. Newly obtained sequence is indicated in bold. The scale bar indicates expected changes per site.
The tree (Figure 6) showed that the C. velatum is clustered with C. tripartitum (EU195974) and two unknown Cruznema populations (MN108239, MN108240) into a fully supported clade (PP = 1, BS = 100), and subsequently sistered to Cephaloboides nidrosiensis (EU195992). The four 28S sequences of the species have 100% identity. The species (GD-1, ON191476) differs from C. tripartitum (EU195974) by 18 nucleotides (98.2% identity), from Cruznema sp. (MN108239) by 36 nucleotides (94.0% identity), from Cruznema sp. (MN108240) by 33 nucleotides (94.2% identity), and from C. nidrosiensis (EU195992) by 198 nucleotides (79.5% identity).

Figure 6. Bayesian 50% majority-rule consensus tree of Cruznema velatum and other related nematodes inferred from 28S rRNA gene. Dataset aligned with G-INS-I implemented in MAFFT. The values at clade node indicate posterior probability/bootstrap. Newly obtained sequence is indicated in bold. The scale bar indicates expected changes per site.
In the ITS tree (Figure 7A), the C. velatum grouped with two unidentified Cruznema populations (MK156051, MW228469) into a fully supported branch (PP = 1, BS = 100). The species differs from Cruznema sp. (MK156051) by 498 nucleotides (20.2% identity), and from Cruznema sp. (MW228469) by 108 nucleotides (86.0% identity). Finally, in the COI tree (Figure 7B), the C. velatum is sistered to Pristionchus maupasi (LC011450) and two populations of Phasmarhabditis hermaphrodita (OL468731, OL468732). The species differs from P. maupasi (LC011450) by 79 nucleotides (88.0% identity), from P. hermaphrodita (OL468731) by 69 nucleotides (89.0% identity), and from P. hermaphrodita (OL468732) by 362 nucleotides (44.0% identity).

Figure 7. Bayesian 50% majority-rule consensus tree of Cruznema velatum and other related nematodes inferred from ITS (A) and COI (B) genes. ITS dataset aligned with G-INS-I implemented in MAFFT, and COI dataset aligned in TranslatorX. The values at each clade node indicate posterior probability/bootstrap. Newly obtained sequence is indicated in bold. The scale bar indicates expected changes per site.
Transcriptome assembly and gene function prediction
The RNA-seq generated a total 71,017,326 clean reads, with 97.8 % of Q20, 94.0 % of Q30, and 51.0 % of GC content (Table 2). After assembly and removal of redundant contigs, we obtained a total of 45,366 unigenes with average length 928 bp. These unigenes were annotated to 72,031 putative protein coding sequences (CDs) (Figure 8A). The NCBI NR database assigned unigenes mostly as vertebrate parasites Ancylostoma ceylanicum (25.0%), Heamonchus contortus (14.8%), and free-living Caenorhabditis remanei (6.7%) (Figure 8B).
Table 2. Statistics of de novo assembly of Cruznema velatum transcriptome.


Figure 8. Length distribution of predicted coding sequences (CDs) and protein sequences from unigenes (A) and their corresponding species annotation in NCBI NR database (B).
Functional analyses were performed using unigenes. In general, 29.9%, 31.3%, 24.8%, and 18.6% of unigenes were annotated in KOG, SwissProt, GO, and KEGG, respectively (Table 3). For the KOG, a total of 13,545 unigenes were annotated and assigned to 26 categories (Figure 9A). The most abundant category was associated with signal transduction mechanisms (2,675), followed by general function prediction (2,440), and posttranslational modification, protein turnover, chaperones (1,405). Most proteins annotated in SwissProt (6,835) have homologs to those species belonging Caenorhabditis.
Table 3. Number of Cruznema velatum annotated unigenes against the public databases.


Figure 9. Functional annotations of Cruznema velatum transcriptomics unigenes. A: KOG functional annotation; B: GO functional annotation; C: KEGG pathway classifications of Cruznema velatum transcriptomics unigenes.
GO ontology was comprised of three domains, with biological processes (6,101) most abundant, followed by cellular components (3,387), and molecular functions (9,815) (Figure 9B). Within biological processes, the top three categories were transmembrane transport (566), proteolysis (542), and protein phosphorylation (513). The most common protein functions in cellular components were protein binding (1,966), ATP binding (1,071), and nucleic acid binding (512). Unigenes 1,058, 665, and 174 were most annotated in the molecular functions: integral component of membrane, membrane, and nucleus. In KEGG annotation (Figure 9C), metabolism (3,870) was the most annotated first-level pathway, followed by organismal systems (3,492), cellular processes (2016), environmental information processing (1,443), and genetic information processing (1,389). Within each pathway, the global and overview maps (1,495) for the endocrine system (794), transport and catabolism (930), signal transduction (1,195), and translation (504) were most abundant second-level pathways.
We further compared the third-level KEGG pathway with model species Caenorhabditis elegans and Pristionchus pacificus, including the metabolism of riboflavin, lipoic acid, and vitamin B6 (Supplementary Figures S1-S7). A similar pattern was also found for thiamine metabolism, except that alkaline phosphatase (K01077) was absent in C. elegans and cysteine desulfurase (K04487) was absent in P. pacificus. The gene synthesizing the pantothenate and CoA, type I pantothenate kinase (K00867) and phosphopantothenate cysteine ligase (K01922) were missing in P. pacificus while they were present in C. velatum and C. elegans. Like P. pacificus, C. velatum lacks the biotin protein ligase (K01942) that was present in C. elegans. For folate biosynthesis, the cyclic pyranopterin monophosphate synthase (K03637) and molybdopterin synthase catalytic subunit (K03635) were found in both C. elegans and C. velatum, but not in P. pacificus. Conversely, alkaline phosphatase (K01077) and sepiapterin reductase (K00072) were absent in C. elegans, but present in C. velatum and P. pacificus.
Discussion
Members of the genus Cruznema are widely presented in soil, yet molecular data is scarce. C. velatum was originally described more than 30 years ago (Brzeski Reference Brzeski1989) without any images or molecular sequences. In the present study, we redescribe this species based on biology, morphology, and molecular data. Based on the 18S, 28S, and ITS of rRNA sequences, our phylogenetic analysis support the genus Cruznema as monophyetic, in line with a previous study (van Megen Reference van Megen, van den Elsen, Holterman, Gerrit, Mooijman, Bongers, Holovachov, Bakker and Helder2009). More recently, the mitogenome of C. tripartitum was sequenced, and the phylogeny placed this species as sister to the clade containing C. elegans and Oscheius chongmingensis (Du et al. Reference Du, Guo, Gao, Wang, Qing and Li2022). Using the identical nematode culture of Du et al. (Reference Du, Guo, Gao, Wang, Qing and Li2022), our detailed morphological study rejects previous identification as C. tripartitum and redescribes it as C. velatum. This misidentification emphasises the difficulty in distinguishing closely related nematode species, even among taxonomists. Indeed, misidentification can occur even with detailed morphology and molecular data, and thus numerous errors may exist in public barcoding databases due to incorrect species identification (Qing et al. Reference Qing, Wang, Karssen, Bucki, Bert and Braun-Miyara2020). Transcriptome analysis revealed that C. velatum shares a series of homolog proteins with parasitic species. The homologs of cuticle collagen domain protein in vertebrate parasitic nematodes were found in C. velatum (Supplementary Table S1). This collagen is known to provide greater resistance to environmental stresses for the free-living stage larvae (Zajac et al. 2020), and it is the main component of the basal layer related to organismal morphogenesis (Kramer et al. Reference Kramer, Johnson, Edgar, Basch and Roberts1988). Similarly, C. velatum has the SCP extracellular domain and SCP-like protein closest to the vertebrate parasitic Haemonchus contortus and Oesophagostomum dentatum, respectively (Supplementary Table S2). The SCP/TAPS proteins are known to be involved in the parasitism of Strongyloides (Hunt et al. Reference Hunt, Tsai, Coghlan, Reid, Holroyd, Foth, Tracey, Cotton, Stanley, Beasley, Bennett, Brooks, Harsha, Kajitani, Kulkarni, Harbecke, Nagayasu, Nichol, Ogura, Quail, Randle, Xia, Brattig, Soblik, Ribeiro, Sanchez-Flores, Hayashi, Itoh, Denver, Grant, Stoltzfus, Lok, Murayama, Wastling, Streit, Kikuchi, Viney and Berriman2016), while their function in C. velatum remains unclear.
Our study on the life cycle of C. velatum reflected its 7–9 day reproductive cycle, longer than that of C. elegans (2.5–4 days) (Hertweck et al. Reference Hertweck, Hoppe and Baumeister2003; Golden & Melov Reference Golden and Melov2007), while it was 4 days in Pristionchus pacificus (Sommer & McGaughran, Reference Sommer and McGaughran2013), but much shorter than among those with higher trophic level like Prionchulus and Mononchus (15–45 days) (Maertens Reference Maertens1975, Grootaert & Maertens Reference Grootaert and Maertens1976). Together with its high fecundity, this species may play an important role in nitrogen mineralization like other rhabditids opportunities.
Although integrated approaches have been implemented in various taxonomic works with detailed morphology, additional molecular barcodes, and biological observations (De Ley et al. Reference De Ley, De Ley, Morris, Abebe, Mundo-Ocampo, Yoder, Heras, Waumann, Rocha-Olivares, Burr, Baldwin and Thomas2005; Fonseca et al. Reference Fonseca, Derycke and Moens2008; Qing et al. Reference Qing, Decraemer, Claeys and Bert2017), “omics” data are generally rare. The presence of a specific protein family, e.g., glycoside hydrolases and vitamin synthesis, may provide new insight into their feeding habits and the mechanisms that drive this adaptation. Furthermore, the transcriptome contains a large number of informative gene sites; thus the transcriptome-based phylogenomic can be a powerful tool to resolve deep phylogeny (Smythe et al. Reference Smythe, Holovachov and Kocot2019). Given the single barcoding gene often lacks resolution in closely related species, while cryptic species are widely present in nematode communities (Palomares-Rius et al. Reference Palomares-Rius, Cantalapiedra-Navarrete and Castillo2014), phylogenomics is thus useful in intraspecific delimitation and cryptic species discovery. In addition, the type of expressed gene and its expression level reflect the nematodes’ biology and their responses to the environment. Consequently, the species description published along with the transcriptome will provide valuable information to further our understanding of biology, genetics, and evolution of the species.
Financial support
This research was supported by the National Natural Science Foundation of China (32001876), National Key R & D Program of China (2022YFD1401101), and the Postgraduate Research & Practice Innovation Program of Jiangsu Province (KYCX22_0767).
Competing interest
None.
Ethical standard
The conducted research is neither related to human nor animal use.
Supplementary material
The supplementary material for this article can be found at http://doi.org/10.1017/S0022149X23000342.