The large intestine of humans hosts an important microbial population that can reach 1010–1011 bacteria cells/g of intestinal content, with nearly 400–1000 species(Reference Quigley1–Reference Egert, de Graaf and Smidt3). This gut microbiota plays a key role in homeostasis and health status by interfering with digestion processes, preventing colonisation by pathogens and modulating the development of the immune system(Reference Maukonen and Saarela4). It also regulates a set of metabolic pathways of the host(Reference Erejuwa, Sulaiman and Ab Wahab5, Reference Sekirov, Russell and Antunes6). Many metabolic diseases, such as obesity, the metabolic syndrome, diabetes and gastrointestinal diseases such as cancer and inflammatory bowel disease, are related to alterations of gut microbiota composition and function, called dysbiosis(Reference Ley, Turnbaugh and Klein7, Reference Peterson, Frank and Pace8).
The gut microbiota is highly influenced by the diet. In particular, the undigested carbohydrates and proteins that reach the large intestine are subjected to fermentation. Hence, intestinal microbes produce gases, such as H2, carbon dioxide, methane, ammonia and hydrogen sulphide (H2S)(Reference Poelaert, Despret and Sindic9), as well as various metabolites, such as SCFA and branched-chain fatty acids (BCFA), phenolic and indole compounds, biogenic amines and sulphur metabolites(Reference Gilbert, Ijssennagger and Kies10).
All these compounds influence the physiological and metabolic functions in humans(Reference Koecher, Noack and Timm11, Reference Min, Hu and Nie12). SCFA are linear carboxylic acids, including acetate, propionate and butyrate, and are produced from fermentation of carbohydrates and fibres. The main BCFA are iso-valerate, iso-butyrate and valerate(Reference Russell, Gratz and Duncan13). BCFA originate from the fermentation of protein and the degradation of their constitutive amino acids(Reference Binder14).
Several studies have demonstrated the impact of dietary fibres (DF) on gut microbial communities, using not only purified DF, such as resistant starch, β-glucan, fructo-oligosaccharides, sugar beet peptic oligosaccharides, guar gum, pea fibre and pectin, but also DF isolated from whole grains(Reference Koecher, Noack and Timm11, Reference Yang, Martínez and Walter15–Reference Yang, Keshavarzian and Rose18). Among the DF, purified inulin and fructo-oligosaccharides were the first non-digestible carbohydrates evaluated for their prebiotic properties, since they can modulate the gut microbiota composition, with consequences on host physiology(Reference Bindels, Delzenne and Cani19).
Fruits, cereals and vegetables are the major sources of DF in the human diet. Strong variation exists among food products regarding the amount and the type of DF they provide(Reference Stone, Chapman and Lovell20, Reference Kalala, Kambashi and Everaert21). Soluble DF is potentially most effective in modulating intestinal microbiota. Carbohydrates embedded in a vegetable food matrix are likely to present a different functionality compared with purified fractions(Reference Williams, Grant and Gidley22).
Purified inulin and fructo-oligosaccharides, given as supplement, on obesity have been highlighted in both preclinical(Reference Catry, Bindels and Tailleux23, Reference Hiel, Neyrinck and Rodriguez24) and clinical studies(Reference Salazar, Dewulf and Neyrinck25, Reference Dewulf, Cani and Claus26). We have shown that only few vegetables provide significant amounts of inulin and fructo-oligosaccharides(Reference Kalala, Kambashi and Everaert21). It could be beneficial to encourage the consumption of inulin-rich vegetables and fruits, in the context of obesity, if their properties of interaction with the gut microbiota are maintained in a food matrix, and if they exert health effect in this particular context.
The present study addressed the question of the relevance of inulin-rich food in the modulation of the functionality of the gut microbiota, by performing an in vitro fermentation, using the faecal material obtained from two groups of obese individuals, before and after a dietary treatment differing by inulin intake. Obese patients received either a diet based on inulin-rich vegetables coupled with inulin supplementation or an inulin-poor diet coupled with maltodextrin supplementation as control for 3 months. The study assessed the ability of these treatments to modify the faecal microbiota composition and fermentation capacity in a humanised in vitro model of the gastrointestinal tract.
Materials and methods
The study was approved by the Faculty Hospital Ethics Committee of the Faculty of Medicine of the Catholic University of Louvain (Woluwe, Belgium) and was registered at clinicaltrial.gov as NCT03852069. The present study was conducted according to guidelines laid down in the declaration of Helsinki, and all procedures involving human patients were approved. Written informed consent was obtained from all patients.
The faeces of twelve obese patients (six females, six males) ranging in age from 21 to 62 years and with a BMI > 30 kg/m2 were used. These patients were able to provide sufficient amounts of fresh faeces before (initial phase) and after a 3-month treatment, consisting of one of the two possible dietary instructions: a diet based on fructan-rich vegetables (treated group) and a diet based on vegetables poor in fructans (control group). Moreover, the treated group was supplemented with a daily dose (16 g) of inulin-type fructans (Fibrulin, Cosucra), and the control group was given 16 g of maltodextrin (Cargill).
Fructan intake (g/d) was evaluated by submitting the patients to a FFQ, and the calculation of fructans’ intake was based on the analysis of fructan content in foodstuffs (vegetables, fruits and cereals) as reported(Reference Kalala, Kambashi and Everaert21, Reference Muir, Shepherd and Rosella27–Reference van Loo, Coussement and de Leenheer29).
The true estimation of inulin and maltodextrin intake (compliance) was evaluated upon bring-back of empty and unused packets. To verify compliance related to the selected vegetable consumption, a phone call with all the patients was organised three times during the study (after 2 weeks, 1 and 2 months of treatment). A meeting with dietitians was also scheduled every month to ensure that patients followed the dietary advice and prepared their meal following the recipe book received at the start of the intervention. This recipe book describes several recipes based on vegetables enriched in fructans for the treated group and recipes with vegetables poor in fructans for the control group. The patients had to report weekly the number of meals corresponding to the selected recipes, or using the vegetables included in the recipes prepared in another way.
Fructans intake (g/d) was evaluated by submitting the patients to a FFQ created in order to include foodstuffs rich in fructans. Indeed, we have previously published the quantitative analysis of fructans in vegetables. The amount of fructans in cereals and fruits has been calculated taking into account published data.
The questionnaire has been validated by the dietitians implicated in the clinical follow-up. We propose to add the questionnaire (in French) as online Supplementary data. Using this questionnaire, fructan intake was calculated on the basis of fructans content analysis in foodstuffs (vegetables, fruits and cereals) as reported.
Faeces were used for in vitro fermentation of the indigestible fibre residue of vegetables rich (salsify and Jerusalem artichoke) and poor (asparagus, fennel, pumpkin and swede) in inulin, as well as purified fibres (inulin and cellulose), to compare the shifts in fermentation patterns resulting from the expected diet-induced changes in microbiota.
For this purpose, a two-step in vitro model of the pig gastrointestinal tract was adapted to human samples. It consists of the enzymatic hydrolysis of food material to simulate digestive processes occurring in the stomach and the small intestine, followed by an in vitro batch fermentation of the indigested residues using faeces as bacterial inoculum.
Enzymatic hydrolysis
Six vegetables were sampled in triplicates (n 3) and steamed for 20–30 min: Jerusalem artichoke (Helianthus tuberous), salsify (Scorzonera hispanica), asparagus (Asparagus officinalis), pumpkin (Cucurbita maxima Duchesne ssp.), fennel (Foeniculum vulgare) and swede (Brassica napobrassica). These vegetables were chosen because they display a variable content of fructans, soluble DF and insoluble DF(Reference Kalala, Kambashi and Everaert21).
Steamed vegetables were hydrolysed in vitro with porcine pepsin and pancreatin, to mimic digestion in the upper gut(Reference Bindelle, Buldgen and Boudry30), and undigested fibre residues were recovered using a 6 kDa dialysis membrane placed in beakers containing distilled water that were under continuous agitation. After 24 h, the undigested fibre residues remaining in the dialysis membrane were freeze-dried.
The composition of vegetables before and after enzymatic hydrolysis is listed in Table 1.
Table 1. Chemical composition of the ingredients and their hydrolysates used for fermentation (n 3) (g/100 g DM) (Mean values with their standard errors)

OM, organic matter; CP, crude protein; IDF, insoluble dietary fibre; HMWSDF, high molecular weight soluble dietary fibre; LMWSDF, low molecular weight soluble dietary fibre; SDF, soluble dietary fibre; TDF, total dietary fibre.
In vitro fermentation using human faecal inoculums
Faecal material of the patients was collected in a plastic container with lid for a hermetic closure (Commode specimen collection system 02-544-208, Fisher Scientific), placed under anaerobiosis by inserting an Anaerocult C bag (Merck KG&A) and kept in a cooler (4°C) during transportation to the laboratory for further processing. Immediately upon arrival, faecal samples were conditioned as follows in a glove box (In vivo2 1000, Dual Chambers, Hypoxia Workstation, Ruskinn Technologies) fed with a stream of N2 and gas mixture (8 % H2, 8 % CO2, 84 % N2): 25 g of faecal material was introduced into sterile stomacher bags (384 ml, Nasco Whirl-Park) using sterile spoons (Spoons B01041, Nasco).
The bags were immediately quenched in liquid N2 to ensure ultra-fast freezing, preventing the destruction of the micro-organisms, and conditioned samples were kept in a freezer at –80°C(Reference Yang, Maldonado-Gomez and Hutkins31) until further use. Faeces samples were processed within a maximum of 2 h following their emission.
The fermentation was carried out according to the protocol of Bindelle et al.(Reference Bindelle, Buldgen and Boudry30) as modified by Tran et al.(Reference Tran, Boudry and Everaert32). For a given individual, both faecal inocula, that is, faeces collected before and after the dietary treatment, were processed on the same day. The day before fermentation, 0·1 g of substrate sample (residues of the hydrolysis of six steamed vegetables, and purified inulin and cellulose) was weighed in triplicate into 50 ml sterile glass vials. Three blanks served as a control. One litre of Menke and Steingass’ buffer (pH 6·8) mixture devoid of Na2S(Reference Poelaert, Nollevaux and Boudry33) was prepared and bubbled with N2. Mucus carriers were impregnated with mucin agar. The next day, all procedures were performed in the glove box at 37°C. The 25 g bag of faecal sample was thawed by immersion in Menke and Steingass’ buffer, to yield a concentration of 1:40 (w/v) and homogenised using a stomacher (Awel microbiology, Mixwel+) for 1 min. Three mucus carriers were placed in each glass vials, and 15 ml of inoculum (faeces and buffer) was poured into the vials containing the substrates. These were air-tight closed and incubated in a water bath at 37°C for 24 h. The pressure in the headspace of the bottles was read at 2, 5, 8, 12, 16, 20 and 24 h, using a Digital Pressure Gauge (Tracker 200)(Reference Theodorou, Williams and Dhanoa34) and converted into volume applying the law of Boyle and Marriott.
After fermentation for 24 h, the supernatants were emptied into 15 ml centrifuge conical tubes and stored at –20°C for analyses of the SCFA. The gas production curve was modelled according to the monophasic model of Groot et al.(Reference Groot, Cone and Williams35), which allows describing fermentation kinetics using four parameters: the total volume of gas produced at 24 h (A: ml/g DM); the time at which half of the total gas volume is obtained (B: h); the maximum rate of gas production (Rmax: ml/h per g DM) and the time at which the maximum rate of production is reached (Tmax: h).
Analysis of SCFA
Fermentation supernatants and inoculum of the twelve donors used in the fermentation study were analysed for SCFA and BCFA contents, by using a Waters 2690 HPLC system (Waters) fitted with an Aminex HPX-87 H column (300 mm × 78 mm; Bio-Rad Laboratories) combined with a Waters 486 tuneable absorbance detector set at 210 nm.
In vitro fermentation data were corrected for the content of the blanks, as well as the inocula. The sterile bottle containing 15 ml of fermentation supernatants was vortexed for 1 min, and 2 ml aliquot was centrifuged at 13 000 rpm for 15 min. Aliquot (1·5 ml) of the supernatants was transferred to a vial, and pH was adjusted to between one and three, using 0·1m HCl.
Statistical analysis
The analysis of the kinetic of fermentation and the SCFA of the fermented ingredients with the faeces of two groups of obese patients was made by comparing the two groups according to the period before and after intervention by ANOVA, using the MIXED procedure of SAS 9.4 software (SAS Inc.). Patients (n 6) were considered as the experimental unit, and the period was the effect that was tested.
Results
The compliance of powdered native inulin and maltodextrin packets intake reached 98·4 and 95·5 % for treated and control groups, respectively. Regarding the qualitative consumption of vegetables enriched in fructans (for the treated group) or vegetables poor in fructans (for the control group), data are available for five patients on six in each group. Based on the weekly follow-up of cooked recipes, patients consumed at least a mean of 5·6 and 4·4 of different recommended vegetables per week in the treated and control groups, respectively, based on the FFQ.
After 3 months of intervention, dietary fructan intake increased significantly in the treated group, whereas no significant effect occurred in the control group (before: 2·54 and 2·22 g/d v. after: 11·03 and 3·6 g/d for the treated group and control groups, respectively).
The results displayed in Table 1 demonstrate that the enzymatic hydrolysis allowed the collection of a significant amount of fibrous residues. These results show that the hydrolysis has led to a considerable decrease of the starch.
The faeces collected from patients used as inocula, displayed different SCFA and BCFA contents and production profiles according to the period (before v. after a 3-month treatment) and the treatment undergone by the patient (treated group v. control group) (Fig. 1).

Fig. 1. SCFA contents in the faeces of two groups of donors before and after the dietary treatment (molar ratio %) (n 6). Branched-chain fatty acids (BCFA) include iso-butyrate, valerate and iso-valerate. , BCFA %;
, butyrate %;
, propionate %;
, acetate %.
Both treatments provoked an increase in SCFA contents of faeces, with a significant difference for the treated group when comparing before and after treatment, but no significant difference before and after for the control group (before: 5·89 and 5·27 mg/g v. after: 10·12 and 8·19 mg/g for treated and control groups, respectively).
The molar ratios of butyrate (treated group) and acetate (treated and control groups) showed a significant difference between the two phases (butyrate before: 13·1 and 14·8 % v. after: 17·1 and 14·9 %, for treated and control groups, respectively; acetate before: 52·5 and 50·6 % v. after: 56·7 and 53·1 % for treated and control groups, respectively).
An increase in microbial fermentation activity (gas production) after treatment was also observed for the treated group, since patients after a 3-month treatment displayed a faster and more extended fermentation, as indicated by an increased Rmax and by higher final gas volumes (A) for most ingredients. The control group did not change gas production after treatment (Table 2).
Table 2. Effects of the ingredient and the period (before and after dietary treatment) on fermentation kinetics modelled according to Groot et al.(Reference Groot, Cone and Williams35) (Mean values with their standard errors)

A, total volume of gas produced (ml/g DM); B, time at which half of the total gas volume is obtained (h); Rmax, maximum rate of gas production (ml/h × g DM); Tmax, time at which the maximum rate of production is reached (h).
a,b,c For one ingredient, values followed by unlike letters in a column indicate that means differed at a significance level of 0·05.
The fermentation kinetics of fibres and vegetable extracts analysed in vitro also showed interesting results. The SCFA production upon 24 h was higher for the treated group after dietary intervention with significant differences compared with the phase before, while the control group did not significantly modify the fermentation profile of any of the two types of ingredients (vegetables and purified fibres) (Table 3).
Table 3. Impact of the ingredient and the period (before and after treatment) on total production of SCFA (mg/g DM) and molar ratio (%) (Mean values with their standard errors)

BCFA, branched-chain fatty acids regrouping iso-butyrate, valerate and iso-valerate.
a,b,c,d For one ingredient, values followed by unlike letters in a column indicate that the organic acids before and after treatments differ at a significance level of 0·05.
Indeed, we observe an increase of total SCFA in the treated group after the dietary intervention when compared with the initial phase, for both vegetables (225–298 v. 465–521 mg/g DM before and after treatment, respectively) and purified carbohydrate (137–315 v. 218–512 mg/g DM before and after treatment, respectively). In addition, the main organic acids, namely, acetate, propionate and butyrate, showed significant differences before and after intervention.
An increase in butyrate was observed after the intervention in the treated group for salsify, Jerusalem artichoke, asparagus and fennel (5–12 % v. 7–14 % before and after the treatment, respectively). Conversely, the control group showed a decrease in SCFA, with slight tendencies to increased butyrate in the post-phase (4–16 % v. 7–16 % before and after the treatment, respectively).
The principal component analysis for parameters related to fermentation kinetics and the SCFA production showed that in the post-treatment phase, the total SCFA, A, Rmax, butyrate and acetate were aligned on the same axis as the treated group. Conversely, Tmax, B, propionate and BCFA were aligned with the control group and inversely proportional to the treated group, with a negative correlation (Fig. 2).
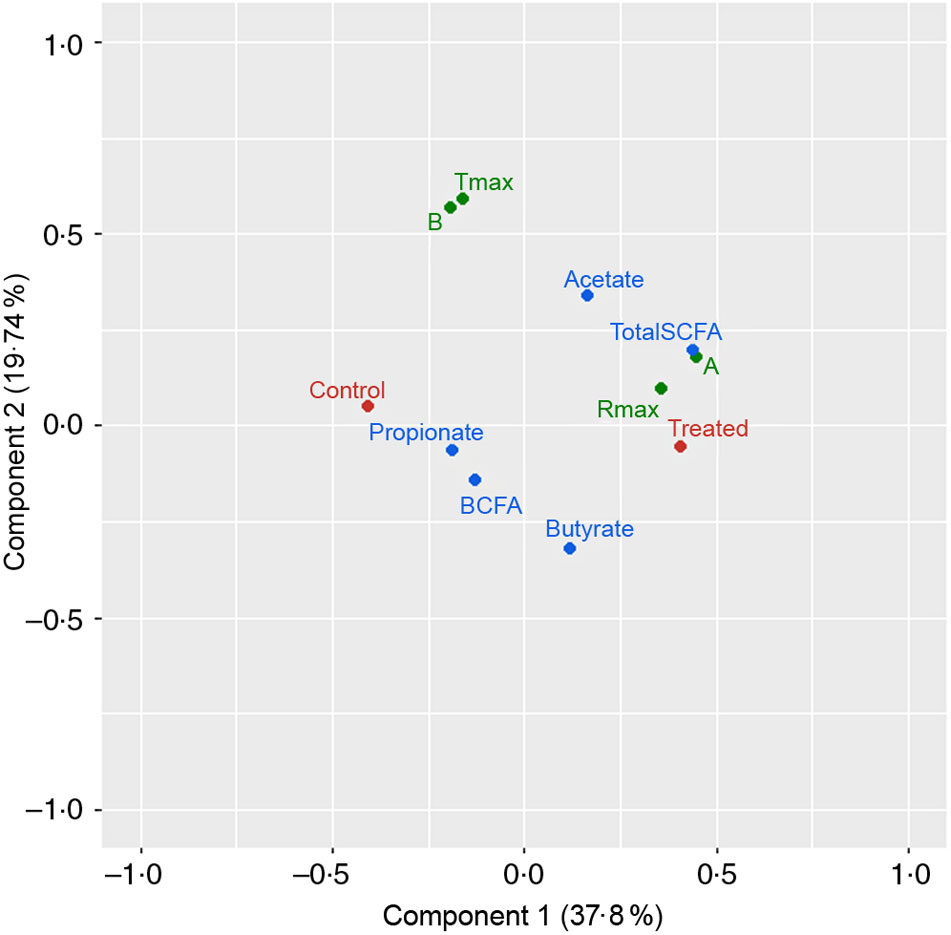
Fig. 2. Principal component analysis for kinetic parameters and SCFA of fermented ingredients after the treatment (n 6). BCFA, branched-chain fatty acids.
Finally, in addition to inulin, which displayed high fermentation, salsify and Jerusalem artichoke were the vegetables that approximated inulin the most in terms of fast fermentation patterns. Cellulose exhibited the slowest fermentation. Regarding the total volume of gas produced, salsify came first, followed by inulin, Jerusalem artichoke and swede. Again, cellulose was the least fermentable.
Discussion
In recent decades, the importance of gut microbiota on health has raised the attention of many, especially concerning the relationship between gut microbiota and obesity(Reference Schwiertz, Taras and Schafer36). Some studies have shown that the fructan content of vegetables positively impacts on the kinetic of fermentation associated with the gut microbial alteration(Reference Dhingra, Michael and Rajput37–Reference Hernot, Boileau and Bauer39). DF is a major factor driving the composition and metabolic activity of colonic microbiota(Reference Bindels, Delzenne and Cani19, Reference Scott, Gratz and Sheridan40). Data from epidemiological studies have shown that diets high in fibre are associated with reduced risk of metabolic diseases, such as diabetes and obesity, and SCFA are often presented as key metabolites involved in the improvement of host physiology(Reference Hur and Reicks41, Reference Weickert and Pfeiffer42). However, the studies relating the impact of inulin on SCFA in obese humans have been obtained through the analysis in the faecal samples, that lead to controversial data, and do not always reflect SCFA production(Reference Salazar, Dewulf and Neyrinck25). The faecal microbiota following a 3-month treatment with fructans has a direct impact on the fermentative capacity of the faeces since a faster fermentation (assessed by gas production) was observed combined with an increased butyrate and acetate production in the treated group. The higher butyrate production at the post-treatment period corroborated the results obtained by Koecher et al.(Reference Koecher, Noack and Timm11), who also analysed the inoculum of non-obese people subjected to a diet rich in purified inulin-type fructans. The post-intervention data obtained in the present trial also showed an increase in total SCFA produced from the inoculum of obese patients that were given the low-inulin diet.
The effect on total SCFA mirrored those obtained by other authors, who specified that total production of SCFA is correlated with the total volume of gas produced. Several criteria, such as the type and amount of substrate ingested, colon integrity and genetics of individual, can influence the production of SCFA(Reference Bouhnik, Vahedi and Achour43–Reference Rösch, Venema and Gruppen45).
The content of fructan in the food products appeared to be the major factor driving the production of SCFA, in the present investigation. Given these results and those obtained by some researchers in similar studies(Reference Rösch, Venema and Gruppen45, Reference Sawicki, Livingston and Obin46), it can be confirmed that vegetables with a high-fructan content increase the production of SCFA in a human in vitro model, but to a lesser extent than the purified inulin, although considerably more than the vegetables poor in inulin. Even though insoluble DF are less fermentable(Reference Uttara47), their importance in the approach of obesity is not negligible. Vegetables rich in insoluble fibres in the present study showed greater parameters of fermentation kinetics and higher SCFA production than those obtained for cellulose in an in vitro model. SCFA are recognised fermentation products of great importance for health, and which perform several functions, such as the regulation of absorption of water and minerals, the reduction of the pH of the colon thus inhibiting the development of pathogens present, while promoting the growth of beneficial bacteria(Reference Sasaki, Sasaki and Ikuta48). Thus, it should be understood that, the relationships between fermentable carbohydrates and metabolic yield depend on the composition of the microbial community and the metabolic characteristics of each anaerobic bacterium(Reference Duncan, Holtrop and Lobley49).
Given the results of the present study and in comparison with other investigations that analysed the SCFA and gut microbiota profile(Reference Duncan, Holtrop and Lobley49), we expect that the large production in the present study will correlate with the modification of the microbiota of the intestinal tract of patients in the treated group. This was observed by the very high rate of fermentation and the significant production of butyrate. Apart from the ability to produce a significant amount of SCFA during fermentation, another benefit of a high-fibre diet is to stimulate the growth of the gut bacteria essential for health.
Conclusion
Inulin-type fructans are involved in the modulation of the gut microbiota and alter the production of both gas and SCFA, during fermentation. An inulin-type fructan intervention in one group of obese patients produced a higher gas volume and higher SCFA amount in vitro, with a trend towards increasing amounts of butyrate. In contrast, the control group presented a lower gas volume and lower total SCFA, after the study trial compared with the initial (pre-trial) phase. We thus confirm that in obese individuals, the faecal microbiota exhibits a higher capacity to modulate SCFA production when they were pretreated with a diet rich and supplemented with inulin. The relevance of such effects on the metabolic response towards inulin-rich diet in a large cohort of obese patients is currently under investigation.
Acknowledgements
The authors thank the dietitians Coralie Franay, Marie Barea and Marjorie Fadeur.
This work was co-funded by the Walloon Government (Namur, Belgium) and Liège University in the framework of the FOOD4GUT excellence research project. G. K. was funded by a PhD grant of Wallonie-Bruxelles International (WBI, Brussels).
B. P., A. N., S. H., J. P. T., N. D. and J. B. designed the research; G. K., B. K., N. E., Y. B., A. R., P. N., B. P., S. H. and J. R. conducted the research; G. K., B. K., B. T., M. S., P. N. and P. F. analysed the data and performed statistical analysis; G. K. and J. B. wrote the paper; J. B. had primary responsibility for final content. All authors read and approved the final manuscript.
None of the authors has any conflicts of interest to declare.
Supplementary material
For supplementary material referred to in this article, please visit https://doi.org/10.1017/S0007114519002915