Introduction
Waterhemp [Amaranthus tuberculatus (Moq.) Sauer] is a pernicious weed species limiting corn (Zea mays L.) (Steckel and Sprague Reference Steckel and Sprague2004), soybean [Glycine max (L.) Merr.] (Hager et al. Reference Hager, Wax, Stoller and Bollero2002b), and grain sorghum [Sorghum bicolor (L.) Moench.] (Feltner et al. Reference Feltner, Hurst and Anderson1969) production in the United States. Amaranthus tuberculatus is a summer annual dicot with C4 physiology and is a dioecious, diploid (2n = 32) outcrossing weed species (Steckel Reference Steckel2007). It is characterized by high fecundity, with female plants producing up to a million seeds that persist in the soil seedbank for years combined with multiple emergence events per year (Hager et al. Reference Hager, Wax, Bollero and Simmons2002a; Steckel et al. Reference Steckel, Sprague, Stoller, Wax and Simmons2007). The dioecious biology of A. tuberculatus contributes to its rich genetic diversity, adaptability, and evolutionary success (Kreiner et al. Reference Kreiner, Latorre, Burbano, Stinchcombe, Otto, Weigel and Wright2022) despite application of various weed control strategies (Tranel Reference Tranel2021). To date, A. tuberculatus is resistant to herbicides from seven site-of-action groups (Heap Reference Heap2023). Most recently, it was postulated that dicamba resistance in a multiple herbicide–resistant (MHR) A. tuberculatus population from central Illinois displayed moderate heritability and is potentially controlled by multiple genes (Bobadilla et al. Reference Bobadilla, Giacomini, Hager and Tranel2022). The growing number of resistance cases to preemergence herbicides among A. tuberculatus populations (Evans et al. Reference Evans, Strom, Riechers, Davis, Tranel and Hager2019; Strom et al. Reference Strom, Gonzini, Mitsdarfer, Davis, Riechers and Hager2019) warrants innovative strategies that quickly detect resistance as well as improve herbicide efficacy (Tataridas et al. Reference Tataridas, Jabran, Kanatas, Oliveira, Freitas and Travlos2022).
Previous studies have characterized the genetics and inheritance of herbicide resistance in A. tuberculatus (Bobadilla et al. Reference Bobadilla, Giacomini, Hager and Tranel2022; Huffman et al. Reference Huffman, Hausman, Hager, Riechers and Tranel2015; Kohlhase et al. Reference Kohlhase, Edwards and Owen2018; Oliveira et al. Reference Oliveira, Gaines, Jhala and Knezevic2018). Importantly, however, these previous studies only investigated foliar-applied herbicides. Field populations of resistant A. tuberculatus are rarely purified via recurrent selection beyond a single generation before crossing with a sensitive population. Genetically purified populations should theoretically have an increased frequency of resistance alleles if subjected to herbicide selection during inbreeding (Falconer Reference Falconer1989; Kohlhase et al. Reference Kohlhase, Edwards and Owen2018). Three generations of recurrent selection designed to increase homozygosity of an Iowa A. tuberculatus population were utilized for creation of an F1 in previous genetic research, which led to reproducible segregation of mesotrione resistance in F2 lines (Kohlhase et al. Reference Kohlhase, Edwards and Owen2018). However, starting without a homogenous, homozygous parent population of mesotrione-resistant A. tuberculatus may have contributed to unclear segregation results in another genetic study (Huffman et al. Reference Huffman, Hausman, Hager, Riechers and Tranel2015).
Resistance to preemergence herbicides is a trait of interest warranting further research to investigate the mechanisms involved. Resistance and inheritance studies of the very-long-chain fatty-acid elongase (VLCFAE)-inhibiting preemergence herbicide pyroxasulfone (Busi et al. Reference Busi, Gaines, Vila-Aiub and Powles2014, Reference Busi, Porri, Gaines and Powles2018; Busi and Powles Reference Busi and Powles2016) and preplant-incorporated herbicide triallate (Brunton et al. Reference Brunton, Boutsalis, Gill and Preston2021) have been conducted in rigid ryegrass (Lolium rigidum Gaudin.). Resistance to pyroxasulfone in L. rigidum is metabolism based and is characterized by upregulation of glutathione S-transferase (GST) and cytochrome P450 (P450) genes (Busi et al. Reference Busi, Gaines, Vila-Aiub and Powles2014, Reference Busi, Porri, Gaines and Powles2018; Busi and Powles Reference Busi and Powles2016). Resistance to S-metolachlor in an A. tuberculatus population from Stanford, Illinois (SIR) occurs via P450- and GST-catalyzed detoxification mechanisms acting in concert (Strom et al. Reference Strom, Hager, Seiter, Davis and Riechers2020, Reference Strom, Hager, Concepcion, Seiter, Davis, Morris, Kaundun and Riechers2021). However, research has not been reported that clearly establishes the genetic basis and inheritance of S-metolachlor resistance in A. tuberculatus.
The current research was designed to investigate inheritance of S-metolachlor resistance in an MHR A. tuberculatus population (SIR). Our working hypothesis is that the S-metolachlor resistance trait in SIR is controlled by a single, dominant, nuclear-encoded gene. Specific aims of our study were to: (1) genetically advance (i.e., increase homozygosity of) the SIR field population via a breeding scheme designed for a dioecious species combined with recurrent selection; (2) optimize a phenotyping assay for VLCFAE-inhibiting, preemergence herbicides; (3) develop F2 populations segregating for resistance to the VLCFAE-inhibiting herbicide, S-metolachlor; and (4) evaluate the inheritance patterns of S-metolachlor resistance in these segregating populations.
Materials and Methods
Generation of Full-Sib Near-Inbred Resistant Lines and Parent Selection
Two A. tuberculatus populations (SIR and SEN) previously investigated for responses to the VLCFAE-inhibiting herbicide S-metolachlor (Kerr Reference Kerr2021) were used as parental populations in this study. SIR is a subpopulation derived from the original MCR (McLean County, Illinois–resistant) A. tuberculatus population (Hausman et al. Reference Hausman, Singh, Tranel, Riechers, Kaundun, Polge, Thomas and Hager2011; Jacobs et al. Reference Jacobs, Butts-Wilmsmeyer, Ma, O’Brien and Riechers2020; O’Brien et al. Reference O’Brien, Davis and Riechers2018). The SIR/MCR population is resistant to herbicides from five site-of-action groups (Concepcion et al. Reference Concepcion, Kaundun, Morris, Hutchings, Strom, Lygin and Riechers2021; Heap Reference Heap2023). The second A. tuberculatus population (SEN) is a standard sensitive population used in previous research (Kaundun et al. Reference Kaundun, Hutchings, Dale, Howell, Morris, Kramer, Shivrain and Mcindoe2017; O’Brien et al. Reference O’Brien, Davis and Riechers2018) and was previously confirmed sensitive to S-metolachlor (Kerr Reference Kerr2021).
To create a uniform, highly resistant population, male and female SIR plants were intermated (Figure 1A) before creation of F1 and segregating F2 A. tuberculatus populations (Figure 2). Three generations of single-plant, full-sib mating and recurrent selection were conducted with male and female SIR plants. Four full-sib near-inbred lines were ultimately obtained, and each line was screened for survival to 2,140 g ai ha−1 S-metolachlor at each cycle via a “toothpick tracker” method in the greenhouse (Figure 1B). Before planting, seeds were surface sterilized and cold stratified in 0.15% agarose (w/w) for at least 30 d, as previously described (Bell et al. Reference Bell, Hager and Tranel2013). Large cell pack inserts (801 Daisy Trays, Greenhouse Megastore, 70 Eastgate Drive, Danville, IL 61834) were filled halfway with soil mix (1:1:1 soil:peat:torpedo sand) with a pH of 6.4 and 3.5% organic matter. A 15-g portion of slow-release fertilizer (Osmocote 13-13-13, Scotts Miracle-Gro Company, 14111 Scottslawn Road, Marysville, OH 43041) was added, then the remainder of the insert was filled with soil mix, patted firmly, and allowed to saturate with water overnight, as previously described (Strom et al. Reference Strom, Gonzini, Mitsdarfer, Davis, Riechers and Hager2019). Stratified A. tuberculatus seeds from SIR were planted onto the moistened soil surface into seven of the eight inserts, while SEN was planted in the eighth insert to ensure herbicide efficacy before selection and transplanting (Figure 1B). S-metolachlor was applied at a rate of 2,140 g ha−1 using a research-grade cabinet sprayer (Generation III Research Sprayer, DeVries Manufacturing, 86956 State Highway 251, Hollandale, MN 56045). Immediately following herbicide treatment, each insert was lightly covered with nontreated soil mix and gently pressed. S-metolachlor–treated flats were placed under an overhead misting system fitted with 0.4 L min−1 nozzles and set on a timer to deliver water three times per day to ensure herbicide incorporation, as previously described (Strom et al. Reference Strom, Gonzini, Mitsdarfer, Davis, Riechers and Hager2019).

Figure 1. Recurrent selection of SIR Amaranthus tuberculatus. (A) Breeding scheme for selection of uniform, highly S-metolachlor–resistant progeny derived from the original SIR field population. Four crosses per generation utilized single male and female resistant plants to select uniformly resistant lines. Numbers in parentheses below DK lines in Generations 1, 2, and 3 represent percent survivorship following recurrent selection with 2,140 g ai ha−1 S-metolachlor (four lines per generation). Survivorship of lines 3-1 and 3-4 was not determined, because lines 3-2 and 3-3 were the most uniform, displayed the highest percent survivorship in Generation 1, and yielded ample viable seeds for analysis. Line DK3-2 (green rectangle) was ultimately chosen for subsequent crosses with sensitive plants. (B) The “toothpick tracker” method of screening for survival at each step following 2,140 g ha−1 S-metolachlor preemergence treatment at 14 d after treatment. Cereal rye (Secale cereale L.) seeds were planted along the edges of all trays to reduce possible border effects.

Figure 2. Generation of F1 and F2 families of SIR Amaranthus tuberculatus derived from reciprocal crosses between full-sib near-inbred line, DK3-2, and a very-long-chain fatty-acid elongase (VLCFAE) inhibitor–sensitive population, SEN. Each cross per generation used single male and female plants. F1 seedlings were screened for survival in soil mix from 2,140 g ai ha−1 S-metolachlor at 14 d after treatment, while F2 seedlings were screened and phenotyped with three concentrations of S-metolachlor (0.5, 1.5, and 3.0 µM) using the preemergence resistance identification method (PRIM) soilless assay (Kerr Reference Kerr2021).
Amaranthus tuberculatus seedlings emerging within the first 7 to 10 d were identified and tracked using toothpicks placed at their bases (Figure 1B). After 14 d, living seedlings marked with a toothpick were recorded (compared with the untreated flat) and were subsequently transplanted for crossing experiments. Seedlings identified as resistant (no visual injury symptoms or growth reduction relative to control plants; Krähmer et al. Reference Krähmer, Dücker, Beffa, Babczinski, Jeschke, Witschel, Krämer and Schirmer2019; Pillai et al. Reference Pillai, Davis and Truelove1979) were transplanted into 720-cm3 pots using BM6 potting soil (Berger BM6 General Purpose Mix, Value-Added Mixing Plant, Watsonville, CA 95076) with slow-release fertilizer (Osmocote®) added, then allowed to grow under mercury-halide lamps delivering 800 µmol m−2 s−1 at 28/22 C day/night with a 16/8-h photoperiod. As A. tuberculatus plants developed into the desired size for producing large amounts of seed, they were placed in framed pollination chambers using 2.5-cm polyvinyl chloride and pollination bags capable of housing one male and one female (full sibs). Each pollination chamber was then placed in a separate greenhouse room using the same growing conditions previously mentioned, but with a 12-h photoperiod to stimulate flowering. Seeds from the full-sib crosses were stratified, planted, treated, and selected with S-metolachlor using the strategy described above for an additional two generations.
After three generations of recurrent selection for each resistant line (Figure 1A), survivorship was used as the primary method for identifying the most suitable parental population, henceforth called DK3-2 (Supplementary Table S1). Mean survivorship (per generation) calculated for the original SIR field population, DK1, DK2, and DK3 following treatment with 2,140 g ha−1 S-metolachlor in soil was 39%, 52%, 95%, and 93%, respectively. Full-sib near-inbred lines were not advanced to a fourth generation of recurrent selection due to the degree of genetic gain in percent survivorship in lines DK3-2 and DK3-3 (Figure 1A). Survival rates of line DK3-2 were 39% (original SIR field population), 71%, 114% and 95%, respectively, from Generation 0 to Generation 3. In Generation 2, the survival of line DK3-2 exceeded 100% due to the nontreated flat having lower than normal germination rates. Among the DK3 lines generated (Supplementary Table S1), DK3-2 consistently demonstrated the highest survival rates following three cycles of recurrent selection with S-metolachlor (Figures 1 and 3). Additionally, potential inbreeding depression was observed beyond two generations of full-sib crossing (data not shown). Inbreeding may have affected seed germination rates and aboveground physiology of mature A. tuberculatus plants, which ultimately reduced overall seed output.

Figure 3. Representative plants from five Amaranthus tuberculatus populations at 14 d after treatment with S-metolachlor: DK3-2, full-sib near-inbred line and R parent; F1♂- F1 paternal-derived R line; F1♀, F1 maternal-derived R line; SIR, original field population; SEN, sensitive parent. Treated pots are arranged from left to right with increasing S-metolachlor concentrations ranging from 0.015 to 15.0 µM. Nontreated controls are included for comparison on the left for each population. Herbicide treatments were applied using the preemergence resistance identification method (PRIM) soilless assay (Kerr Reference Kerr2021).
Generation of F1 and F2 Families
The full-sib near-inbred A. tuberculatus line, DK3-2, was used to choose R parents in subsequent reciprocal crosses with SEN. Stratified seeds of DK3-2 and the SEN population were planted, screened, and tracked for survival to 2,140 g ha−1 S-metolachlor using the method outlined in Figure 1B. Corresponding nontreated seedlings of each population were also prepared. Selected seedlings from DK3-2 and SEN at a similar growth stage were then transplanted after 14 d into larger pots, as previously described. At flowering, pollination chambers were constructed with each chamber containing one DK3-2 male parent and one SEN female parent. These crosses and the respective reciprocal cross (one DK3-2 female parent and one SEN male parent) were placed in separate greenhouse rooms and grown under the same conditions previously described. Mature female plants were harvested, and seed was threshed and stratified (F1 progeny) for full-sib crosses to produce F2 progeny (Figure 2). The two F1 A. tuberculatus lines derived from DK3-2 × SEN and SEN × DK3-2 cross are designated F1-♂ and F1-♀, respectively (Figure 1B; Supplementary Table S1). Maternal and paternal F1 progenies were grown and selected using the previously described method (Figure 1B). Before flowering, two pairs of F1-♂ siblings and two pairs of F1-♀ siblings were placed in pollination chambers, producing four F2 families designated as F2-♂-1, F2-♂-2, and F2-♀-1, F2-♀-2 (Supplementary Table S1).
Soilless Hydroponic Plant Culture
Different concentrations of formulated S-metolachlor (Dual Magnum®, Syngenta Crop Protection, Greensboro, NC 27419) were used for dose–response experiments. These herbicide concentrations were previously identified as effective for differentiating dose responses to S-metolachlor among A. tuberculatus populations using a soilless greenhouse assay described previously (Kerr Reference Kerr2021). Dry, medium-textured, exfoliated vermiculite (Vermiculite, Thermo-O-Rock East, New Eagle, PA 15067) was used to fill plastic cell pack inserts (31801 Deep Insert, BFG Supply, 3708 Enterprise Drive, Suite 180, Janesville, WI 53546), which were arranged in the greenhouse using a randomized complete block design with “replicate” as a blocking factor. Greenhouse conditions were set at 28/22°C day/night under a 16-h photoperiod and maintained throughout the two independent experimental runs (separated in time). Supplemental light was provided using mercury-halide lamps providing 800 µmol m−2 s−1 photon flux at the vermiculite surface. Pots were subirrigated every second day with 150 ml water-soluble fertilizer (Peters Hydroponic Special 5-11-26, ICL Specialty Fertilizers, Summerville, SC, USA) not containing S-metolachlor.
Herbicide Screening of Parent, F1, and F2 Populations
Amaranthus tuberculatus populations F1-♂, F1-♀, F2-♂-1, F2-♂-2, F2-♀-1, and F2-♀-2 were treated with three concentrations of S-metolachlor (0.5, 1.5, and 3.0 µM) under greenhouse conditions using a soilless assay (Kerr Reference Kerr2021). The two experimental runs evaluated 91 F1-♂, 94 F1-♀, 168 F2-♂-1, 161 F2-♂-2, 172 F2-♀-1, and 145 F2-♀-2 seedlings at 14 d after treatment. Segregation data for [F2-♂-1 and F2-♂-2] or [F2-♀-1 and F2-♀-2] were pooled, resulting in a total of 329 F2-♂ and 317 F2-♀ seedlings evaluated using two phenotypic classes: resistant (R) and sensitive (S). Due to the lack of genetic markers, R and S phenotypes were based solely on plant survival, which precluded the ability to identify possible heterozygotes. The full-sib near-inbred A. tuberculatus line (DK3-2), original SIR field population, SEN, F1-♀, and F1-♂ were chosen for dose–response experiments to compare resistance levels after treatment with various S-metolachlor concentrations (Kerr Reference Kerr2021). The DK3-2 line was treated with seven S-metolachlor concentrations ranging from 0.15 to 15.0 µM, and SEN was treated with six S-metolachlor concentrations ranging from 0.015 to 3.0 µM. The F1-♀, F1-♂, and SIR populations were treated with eight S-metolachlor concentrations ranging from 0.05 to 15.0 µM. Dose–response experiments using these concentrations were conducted with two experimental runs (separated in time) using the preemergence resistance identification method (PRIM) soilless assay described previously (Kerr Reference Kerr2021).
Statistical Analysis
Survival data were collected 14 d after treatment from two independent experimental runs consisting of surviving plant counts and respective aboveground biomass. Dose–response analysis was conducted with the drc package in R (v. 4.2.1) and RStudio (v. 2022.07.1) using a three-parameter logistic regression model (Knezevic et al. Reference Knezevic, Streibig and Ritz2007) with Equation 1:

where d is the upper limit, b is the slope of the curve, and e is the 50% reduction in seedling survival (LD50). A three-parameter model was used instead of other log-logistic models based on lack-of-fit tests conducted at α = 0.05, indicating all A. tuberculatus populations could be combined in the same model and accurately compared.
Chi-square (χ2) goodness-of-fit tests (Cochran Reference Cochran1952) were used to test whether the F2 segregation patterns of R and S A. tuberculatus seedlings after treatment with S-metolachlor (0.5, 1.5, and 3.0 µM) followed a single dominant gene or multiple gene model. The χ2 was calculated using Equation 2:

where O i is the observed frequency count for the ith level of the categorical variable, and E i is the expected frequency count for the ith level of the categorical variable. The null hypothesis (H0) in this study was: “survival ratio to 1.5-µM S-metolachlor in the F2 population is not significantly different from a 3:1 (R:S) single-gene model.” If the χ2 goodness-of-fit tests for each χ2 estimate resulted in P ≥ 0.05, H0 is accepted; otherwise, H0 is rejected, and the alternative hypothesis (Ha), which was “survival ratio to 1.5-µM S-metolachlor in the F2 population is significantly different from a 3:1 (R:S) single-gene model,” is accepted. The Ha would also lead to further hypotheses and experimental procedures to investigate the potential multigenic nature of S-metolachlor resistance in MHR A. tuberculatus, which was beyond the scope of the current study. The ratio of alive (R) to dead (S) plants relative to nontreated controls for each F2 family were fit to one-gene and two-gene phenotypic models at α = 0.05.
The probability of resistance was calculated using an odds ratio described previously (Kohlhase et al. Reference Kohlhase, Edwards and Owen2018) by analyzing an equal number of progenies from each F1 and F2 line. The odds ratio is the ratio between the odds of resistance in F1 versus F2 families. If the ratio is >1, the probability is higher in the F1; if the ratio is <1, the probability is higher in the F2; and if the ratio = 1, the probability is equal among F1 and F2 lines. The normality of data was analyzed using a Kolmogorov-Smirnov test at α = 0.05.
Results and Discussion
Dose–Response Analysis of SIR, SEN, and F1 Lines
In the current study, we aimed to identify the genetic basis of S-metolachlor resistance in the A. tuberculatus population, SIR. Three cycles of recurrent selection were first carried out to increase homozygosity of the SIR population and produce the DK3-2 line (Figure 1A); for example, survival rates of DK3-2 were 39% (SIR population), 71%, 114%, and 95%, respectively, proceeding from Generation 0 to Generation 3. Recurrent selection also increased the level of S-metolachlor resistance compared with SIR, which is exemplified by a significantly higher LD50 value (3.7-fold) in DK3-2 compared with SIR (Table 1; Figure 4). If the flow of pollen from one male to one female A. tuberculatus plant is restricted, all loci conferring resistance can in theory be stacked into one population and compared with the original field population. Generation of reciprocal of DK3-2 and SEN (R × S) subsequently yielded F1 progenies (Figure 2).
Table 1. Lethal dose (LD) estimates of 50% (LD50) and 90% (LD90) Amaranthus tuberculatus control with S-metolachlor at 14 d after treatment in five populations using the preemergence resistance identification method (PRIM) soilless assay (Kerr Reference Kerr2021).
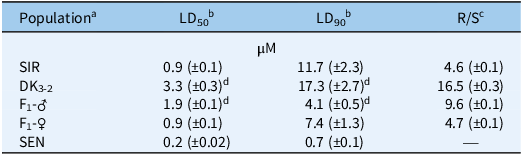
a SIR, Stanford, Illinois–resistant population from McLean County, IL. SEN, standard sensitive population used in previous research (Kaundun et al. Reference Kaundun, Hutchings, Dale, Howell, Morris, Kramer, Shivrain and Mcindoe2017; Kerr Reference Kerr2021; O’Brien et al. Reference O’Brien, Davis and Riechers2018).
b Estimated LD values are expressed as S-metolachlor concentrations (µM) followed by standard errors of the mean in parentheses.
c Resistant-to-sensitive (R/S) ratios derived from LD50 estimates of each A. tuberculatus population relative to SEN.
d LD estimate is significantly higher than the LD50 of the field population, SIR (P < 0.05).

Figure 4.
Amaranthus tuberculatus survival in response to increasing concentrations of S-metolachlor in a dose–response experiment of four A. tuberculatus populations using the preemergence resistance identification method (PRIM) soilless assay (Kerr Reference Kerr2021). Survival data were collected 14 d after treatment by recording the number of living plants as a percent of the untreated control. Lines in each graph were fit using Equation 1: where d is the upper limit, b is the slope of the curve, and e is the 50% reduction in seedling survival (LD50). Each error bar represents ± SE. SEN, S parent; SIR, original field population; F1-♀, F1 maternal-derived R line; F1-♂, F1 paternal-derived R line; DK3-2, purified R × R population and R parent.
Dose–response analysis of SIR, SEN, line DK3-2, paternal F1 (F1-♂), and maternal F1 (F1-♀) families resulted in different responses to S-metolachlor (Table 1; Figures 3 and 4). As expected, the LD50 value of SIR was significantly higher than that of SEN. However, DK3-2 exhibited a significantly higher LD50 than SIR, indicating a successful purification of the original SIR population after three cycles of full-sib mating (Figure 1). The F1-♂ and F1-♀ progeny displayed intermediate responses (measured by LD50 and LD90 values) to S-metolachlor relative to parental lines DK3-2 and SEN (Table 1; Figure 4). However, the LD50 value of F1-♂ was significantly higher than that of SIR, whereas F1-♀ had an LD50 value similar to SIR (Table 1), which may reflect heterosis in both F1 progeny (Figure 3). In addition, calculated R/S ratios based on LD50 values in DK3-2 and F1-♂ were 16.5- and 9.6-fold higher than for SEN, whereas SIR and F1-♀ were only 4.6- and 4.7-fold higher (Table 1). Interestingly, the maternal F1-♀ line demonstrated higher survival levels at S-metolachlor concentrations exceeding 1.5 µM compared with the paternal F1-♂ line (Figures 3 and 4). As a result, it is possible that: (1) the F1-♀ possesses additional resistance or sensitivity genes (see discussion below ‘Probabilities of S-Metolachlor Resistance and Segregation Analysis in F2 Families’ regarding F2 populations), (2) these resistance genes are inducible by S-metolachlor (compared with F1-♂), (3) unknown maternal effects are present, or (4) unequal inheritance was rendered by cellular concentration-dependent resistance mechanisms (Nobusawa et al. Reference Nobusawa, Okushima, Nagata, Kojima, Sakakibara and Umeda2013; Nobusawa and Umeda Reference Nobusawa and Umeda2012). Alternatively, variations in parentally biased gene expression between maternal and paternal lines could also be explained by an epigenetic phenomenon called genomic imprinting (Batista and Köhler Reference Batista and Köhler2020; Pignatta et al. Reference Pignatta, Erdmann, Scheer, Picard, Bell and Gehring2014). However, further research is needed to test these theories.
Probabilities of S-Metolachlor Resistance and Segregation Analysis in F2 Families
Pooled probabilities of resistance based on survival data from F1 and F2 families treated with three concentrations of S-metolachlor indicated equal probabilities at 0.5 µM but higher probabilities in the F2 at 1.5 and 3.0 µM, with odds ratios of 0.85 and 0.84, respectively (Table 2). A higher probability of resistance in the F2 generation at the higher herbicide concentrations could indicate a greater number of dominant alleles present in the F2 at each contributing locus (Kohlhase et al. Reference Kohlhase, Edwards and Owen2018). When considering reciprocal F2 families separately, however, a difference was noted in the calculated probabilities of resistance when comparing maternal- and paternal-derived F1 and F2 lines at 0.5-, 1.5-, and 3.0-µM S-metolachlor (Table 3). F2-♂ lines exhibited higher probabilities of resistance than their F1 parents at each concentration, whereas the F2-♀ lines exhibited similar (at 1.5 and 3.0 µM) or slightly lower (at 0.5 µM) probabilities of resistance as their F1 parents (Table 3). A potential explanation for these different trends in probabilities is that maternal-derived F1 and F2 populations may contain a putative recessive allele at a second, unlinked locus that lowers the magnitude of S-metolachlor resistance (or increases sensitivity) in the F2 population compared with the F1, which is not present in the paternal-derived F1 and F2 populations created in our study (further described below).
Table 2. Probabilities of resistance from pooled reciprocal crosses in F1 and F2 Amaranthus tuberculatus generations at three concentrations of S-metolachlor 14 d after treatment using the preemergence resistance identification method (PRIM) soilless assay (Kerr Reference Kerr2021).

a Estimated values are expressed as S-metolachlor concentrations (µM) followed by their respective standard errors of the mean.
b The odds ratio measures the resistance probability of the F1 generation compared with the resistance probability of the F2 generation (odds of resistance in F1/odds of resistance in F2). If ratio is >1, the probability of resistance is higher in the F1; if ratio is <1, the probability of resistance is higher in the F2; if ratio = 1, the probability of resistance is the same.
Table 3. Pooled probabilities of resistance of the F1 and F2 Amaranthus tuberculatus SIR generations within reciprocal crosses at three concentrations of S-metolachlor at 14 d after treatment using the preemergence resistance identification method (PRIM) soilless assay (Kerr Reference Kerr2021).

a Reciprocal crosses were generated from crossing a very-long-chain fatty-acid elongase (VLCFAE) inhibitor–sensitive population (SEN) with a purified parent derived from multiple herbicide–resistant A. tuberculatus, SIR (DK3-2). F2-♂ were derived from the parent cross (SEN-♀ × DK3-2-♂), whereas F2-♀ were derived from parent cross (DK3-2-♀ × SEN-♂).
b Estimated values are expressed as S-metolachlor concentrations (µM).
c The odds ratio measures the resistance probability of the F1 generation compared with the resistance probability of the F2 generation within each reciprocal cross (odds of resistance in F1/odds of resistance in F2). If ratio is >1, the probability of resistance is higher in the F1; if ratio is <1, the probability of resistance is higher in the F2; if ratio = 1, the probability of resistance is the same.
The χ2 tests for goodness of fit were performed with F2 segregation data generated from only 1.5-µM S-metolachlor (Table 4). A 3:1 (R:S) phenotypic ratio indicates a single dominant gene model. A phenotypic ratio of 9:7 (R:S) is consistent with a two-gene, complementary gene action model, indicative of an epistatic interaction between two unlinked loci (Falconer Reference Falconer1989). Chi-square tests for goodness of fit at 0.5- and 3.0-µM S-metolachlor concentrations were not pursued in this study. Segregation analysis at 0.5-µM S-metolachlor was deemed limited, because this concentration was likely not effective in killing 100% of sensitive plants, based on LD90 values in Table 1. Additionally, 3.0-µM S-metolachlor likely killed a significant portion of resistant plants by overwhelming metabolic resistance, leading to possibly inaccurate survival data compared with segregation results obtained with 1.5 µM (Table 4).
Table 4. Chi-square (χ2) goodness-of-fit analysis of pooled paternal-derived (F2-♂) and maternal-derived (F2-♀) F2 Amaranthus tuberculatus, SIR populations. a

a Survival values were derived from the ratio of number of living plants to total seedlings at 14 d after treatment with 1.5-µM S-metolachlor using the preemergence resistance identification method (PRIM) (Kerr Reference Kerr2021). χ2 values were calculated using Equation 2: χ2 = ∑{[(O i − E i )2]/E i } (Cochran Reference Cochran1952).
b Expected values are calculated from a 0.75:0.25 resistant:sensitive (3:1) ratio.
c Expected values are calculated from a 0.5625:0.4375 resistant:sensitive (9:7) ratio.
d Calculated P-value is greater than 0.05; population fits one-gene model.
e Calculated P-value is greater than 0.05; population fits two-gene model.
Results of the χ2 goodness of fit test among segregating (F2-♂) F2 families based on survivorship of seedlings at 1.5-µM S-metolachlor indicate that inheritance of S-metolachlor resistance fits a one-locus model (α = 0.05; Table 4). Therefore, a single, dominant gene governs the 3:1 (R:S) phenotypic ratio and confers resistance to S-metolachlor at the 1.5-µM concentration in F2-♂ lines (Table 4). By contrast, segregation of F2-♀ lines best fits a two-locus model of 9:7 (R:S), consistent with two complementary genes wherein one homozygous recessive locus (rr) interacts with dominant allele(s) at a different locus (R-) controlling resistance (Bernardo Reference Bernardo2014; Falconer Reference Falconer1989). The most plausible biochemical explanation for the single, dominant gene model is that S-metolachlor resistance in SIR is conferred by a single P450 gene encoding a P450 enzyme that rapidly detoxifies the herbicide (Strom et al. Reference Strom, Hager, Seiter, Davis and Riechers2020, Reference Strom, Hager, Concepcion, Seiter, Davis, Morris, Kaundun and Riechers2021). However, the two-gene model described above is consistent with a single P450 gene that primarily confers resistance, but a second recessive gene (if homozygous) decreases the magnitude of S-metolachlor resistance by an unknown mechanism.
A genetic mechanism that could explain why paternally versus maternally derived F2 lines differed in their segregation ratios, and ultimately fit either one-gene or two-gene models (Table 4), is that the different SEN parent plants (SEN-♂ and SEN-♀) utilized in the reciprocal crosses (Figure 2) may not have been equally S-metolachlor sensitive. This scenario might have occurred, as the SEN population was not screened for sensitivity to S-metolachlor, but instead was presumed to be uniformly sensitive. For example, the SEN parent plant used to create the maternally derived lines (SEN-♂) may have been heterozygous or homozygous recessive for increased sensitivity to S-metolachlor at a second, different locus. A similar scenario was postulated to occur wherein two imidazolinone-resistant eastern black nightshade (Solanum ptycanthum Dunal) populations with identical target-site mutations and rates of imazamox metabolism exhibited different levels of whole-plant resistance (Volenberg et al. Reference Volenberg, Tranel, Holt, Simmons, Weller, Sharkhuu and Riechers2007).
S-Metolachlor Resistance: Mechanisms and Implications for Amaranthus tuberculatus Management
Our research posits a single, major gene confers S-metolachlor resistance in the SIR population and the full-sib near-inbred line, DK3-2. Similarly, resistance to another VLCFAE-inhibiting herbicide, pyroxasulfone, in L. rigidum is conferred by a single, semi-dominant allele (Busi et al. Reference Busi, Gaines, Vila-Aiub and Powles2014). The S-metolachlor resistance trait likely resulted from multiple years of preemergence and postemergence herbicide usage with the same sites-of-action (and/or similar biokinetic properties), which selected for gene(s) and enzyme(s) that metabolize multiple herbicides in SIR (Concepcion et al. Reference Concepcion, Kaundun, Morris, Hutchings, Strom, Lygin and Riechers2021; Hausman et al. Reference Hausman, Singh, Tranel, Riechers, Kaundun, Polge, Thomas and Hager2011, Reference Hausman, Tranel, Riechers, Maxwell, Gonzini and Hager2013; Jacobs et al. Reference Jacobs, Butts-Wilmsmeyer, Ma, O’Brien and Riechers2020; Obenland et al. Reference Obenland, Ma, O’Brien, Lygin and Riechers2019; Strom et al. Reference Strom, Gonzini, Mitsdarfer, Davis, Riechers and Hager2019). However, it has yet to be determined genetically if these resistance cases are examples of cross-resistance (i.e., pleiotropic) or multiple resistance.
The crossing strategy used in our research (one R male × one R female) combined with recurrent selection produced a superior resistant line, DK3-2, from a heterogenous SIR field population (Figure 1). This strategy was necessary, because the original SIR population had displayed variable responses in dose–response experiments with S-metolachlor compared with a different MHR (including VLCFAE-inhibitor resistance; Strom et al. Reference Strom, Gonzini, Mitsdarfer, Davis, Riechers and Hager2019) A. tuberculatus population from central Illinois, CHR (Kerr Reference Kerr2021), indicating the possibility of segregation for resistance gene(s) in SIR.
It is possible a VLCFAE inhibitor–resistant population existed at the McLean County, Stanford, Illinois, site before field scouts noticed the lack of chemical control, particularly because S-metolachlor is typically not used alone for managing A. tuberculatus (Strom et al. Reference Strom, Jacobs, Seiter, Davis, Riechers and Hager2022). Furthermore, resistant waterhemp may have occurred before Group 5 (PSII inhibitors; e.g., atrazine and simazine) and Group 27 herbicides (HPPD inhibitors; e.g., mesotrione, topramezone, tembotrione) no longer controlled weed escapes (Hausman et al. Reference Hausman, Singh, Tranel, Riechers, Kaundun, Polge, Thomas and Hager2011; Strom et al. Reference Strom, Gonzini, Mitsdarfer, Davis, Riechers and Hager2019). Thus, resistance to VLCFAE-inhibiting herbicides in A. tuberculatus represents a relatively new trait of interest that warrants further research to investigate the underlying gene involved and to better understand how selection pressures shaped the evolutionary history and successful adaptation of A. tuberculatus (Kreiner et al. Reference Kreiner, Latorre, Burbano, Stinchcombe, Otto, Weigel and Wright2022).
The DK3-2 line as well as maternal- and paternal-derived F1 and F2 lines (Supplementary Table S1) provide unique genetic stocks for identifying the precise molecular mechanism conferring VLCFAE-inhibiting herbicide resistance. Although S-metolachlor resistance in A. tuberculatus is primarily conferred by enhanced metabolism—where P450 (primary) and GST (secondary) enzymes play important detoxification roles (Strom et al. Reference Strom, Hager, Seiter, Davis and Riechers2020, Reference Strom, Hager, Concepcion, Seiter, Davis, Morris, Kaundun and Riechers2021)—it is also possible transcription factors may affect expression of these metabolic genes or that altered target-site genes encoding the large family of plant VLCFAE elongases (Böger Reference Böger2003; Haslam and Kunst Reference Haslam and Kunst2013; Krähmer et al. Reference Krähmer, Dücker, Beffa, Babczinski, Jeschke, Witschel, Krämer and Schirmer2019; Trenkamp et al. Reference Trenkamp, Martin and Tietjen2004) may play a secondary role. Ongoing work in our lab utilizing proteomic, transcriptomic, and tissue-specific expression analysis in roots, etiolated shoots, and leaves is aimed at identifying which specific metabolic enzyme confers VLCFAE-inhibitor resistance in A. tuberculatus. Future gene mapping research, using the pseudo-F2 progenies generated by this work (Figure 2) combined with publicly available genomic resources (Montgomery et al. Reference Montgomery, Giacomini, Waithaka, Lanz, Murphy, Campe, Lerchl, Landes, Gatzmann, Janssen, Antonise, Patterson, Weigel and Tranel2020), will aim to identify resistance genes and alleles. Metabolism-based phenotyping could also be conducted on our pseudo-F2 lines to assist in comprehensively understanding the molecular-genetic basis of S-metolachlor resistance in SIR, which may ultimately lay the foundation for understanding VLCFAE-inhibiting herbicide resistance in additional MHR A. tuberculatus populations (Strom et al. Reference Strom, Gonzini, Mitsdarfer, Davis, Riechers and Hager2019) as well as in other dicots (Brabham et al. Reference Brabham, Norsworthy, Houston, Varanasi and Barber2019). A further understanding of the genetics and inheritance of VLCFAE inhibitor resistance in dioecious amaranths is crucial to assist with the identification of genes conferring resistance to preemergence herbicides, as well as to develop molecular markers for identification of resistant populations and streamline research efforts for improved management of A. tuberculatus.
Supplementary material
To view supplementary material for this article, please visit https://doi.org/10.1017/wsc.2023.63
Acknowledgments
We thank the University of Illinois at Urbana-Champaign (UIUC) Herbicide Evaluation program; Brian Diers and Eliana Monteverde (UIUC) for technical assistance; Syngenta Ltd. for providing A. tuberculatus seeds, herbicides, and partial funding for this study; and Deepmala Sehgal and Deepak Kaundun, Syngenta Ltd., for critically reviewing the article draft. No competing interests have been declared.