Introduction
Obstructive sleep apnea (OSA) is characterized by episodic upper airway collapse, which is sleep state dependent, resulting in periodic reductions or cessations in ventilation, hypoxia, hypercapnia, or arousals from sleep.Reference Dempsey, Veasey, Morgan and O'Donnell1 OSA affects about 25% of adults in the USA and is a leading cause of excessive sleepiness, resulting in a lower quality of life, impaired work performance, and an increased risk of a car accident.Reference Punjabi2
OSA is also linked to various long-term health problems, including cardiovascular disease, metabolic disorders, and psychiatric problems.Reference Drager, Togeiro, Polotsky and Lorenzi-Filho3–Reference Wheaton, Perry, Chapman and Croft5 Furthermore, numerous reports have associated cognitive difficulties in memory and new learning, attention, and executive function.Reference Bédard, Montplaisir, Richer, Rouleau and Malo6–Reference Beebe and Gozal9 A meta-analysis of six prospective studies have found that 26% of patients with OSA experience significant cognitive decline or dementia.Reference Leng, McEvoy, Allen and Yaffe10 Another study has found that the combined prevalence of depressive symptom in patients with OSA is about 35%.Reference Garbarino, Bardwell, Guglielmi, Chiorri, Bonanni and Magnavita11 Sleep fragmentation, often seen in patients with OSA, may play a role in the cognitive impairments associated with OSA by disrupting neural networks, particularly in the frontal lobes.Reference Beebe and Gozal9 It contributes to cognitive impairments, particularly attention and memory problems.Reference Verstraeten12,Reference Daurat, Foret, Bret-Dibat, Fureix and Tiberge13 Decreased sleep efficiency also reduces the efficacy of restorative processes, resulting in cellular and biochemical stress.Reference Madsen14,Reference Maquet15 Another cause of cognitive impairments is the intermittent hypoxia associated with OSA.Reference Bartlett, Rae and Thompson16 These changes affect cell neurogenesis and the density in the hippocamus.Reference Bartlett, Rae and Thompson16,Reference Hopkins, Kesner and Goldstein17 These factors contribute to OSA, increasing the risk of mild cognitive impairment, Alzheimer’s disease (AD), and other types of dementia.Reference Mubashir, Abrahamyan and Niazi18–Reference Lutsey, Misialek and Mosley20
Brain magnetic resonance imaging (MRI) studies have found that atrophy of the hippocampus and amygdala in older adults with normal cognitive function is a risk factor for developing dementia.Reference den Heijer, Geerlings, Hoebeek, Hofman, Koudstaal and Breteler21 The amygdala’s and hippocampus’s asymmetric atrophy could also be a particularly sensitive indicator for detecting early cognitive impairments.Reference Yue, Wang and Wang22 Previous research has found that patients with OSA have impaired attention, memory, emotion, and executive functions linked to multiple brain regions, especially in the amygdala and hippocampus.Reference Torelli, Moscufo and Garreffa23 The basolateral amygdala/hippocampus are the regions of structural atrophy and functional disturbances in OSA, and these changes are linked to emotional, sensory, and limbic dysfunction.Reference Tahmasian, Rosenzweig and Eickhoff24 The limbic system is a network of interconnected cortical and subcortical structures that is responsible for connecting visceral states, emotion, and cognition to behavior.Reference Mesulam25 It is well known that the limbic system’s activation during sleep plays a crucial role in memory consolidation.Reference Luppi, Billwiller and Fort26 Thus, we could assume that the patients with OSA have abnormalities in the limbic system, which could be detected by brain MRI.
In the past, it was challenging to obtain volume automatically. Recently, machine learning techniques have become available to segment and determine the volumes of the limbic structures, including the hippocampus, amygdala, thalamus, mammary body, hypothalamus, basal forebrain, septal nuclei, fornix, and nucleus accumbens.Reference Greve, Billot and Cordero27 Furthermore, graph theory, which uses natural frameworks to handle large networks analytically, may quantify the topological configuration of brain connections and evaluate brain efficiency for information processing and network features.Reference Bullmore and Sporns28,Reference Pasemann29 Graph theory based on the limbic structure volumes can provide the state of the intrinsic limbic network. However, no studies have focused on limbic structure volumes and investigated the intrinsic limbic network in patients with OSA compared to healthy controls. Abnormalities in limbic structures in patients with OSA may suggest that OSA is associated with cognitive impairments.
We investigated the change in limbic structure volumes and intrinsic limbic network in patients with OSA in this study compared to healthy controls. We hypothesized that there were significant alterations of limbic structure volumes and intrinsic limbic networks in patients with OSA.
Methods
Participants: Patients with OSA and Healthy Controls
This study took place in a tertiary care hospital. The hospital’s institutional review approved this study board, which was conducted in accordance with the Declaration of Helsinki. We retrospectively identified patients who met the following criteria for OSA: Reference Kapur, Auckley and Chowdhuri30 1) a diagnosis of OSA based on laboratory polysomnography demonstrating an apnea-hypopnea index (AHI) >5 in addition to symptoms such as sleepiness or chronic snoring, 2) OSA was the only medical or neurological disorder, 3) no structural lesions on brain MRI on visual inspection, 4) no complaints of cognitive impairment, and 5) with three-dimensional T1-weighted MRI data, which were suitable for volumetric analysis. Patients with OSA did not complain of memory loss or problems in daily living. We collected clinical and polysomnographic data from patients with OSA, including their age, sex, Epworth sleepiness scale score, total sleep time, sleep efficiency, the ratio of sleep stages N1, N2, N3, and R during sleep, total AHI during sleep, AHI during stage N, AHI during stage R, and total respiratory disturbance index during sleep.
We calculated a value of %AHI and defined the patients with non-rapid eye movement (NREM)-predominant OSA (more than 66.7% of %AHI) and rapid eye movement (REM)-predominant OSA (less than 33.3% of %AHI).Reference Yamauchi, Fujita and Kumamoto31
Our control group was age and sex matched with study cases. They had been previously recruited from our study,Reference Jang, Lee, Lee and Park32 who did not have a history of medical or neurological disorders. They had a normal brain MRI on visual inspection. None complained of snoring or other OSA symptoms and, therefore, did not have polysomnography testing.
MRI Acquisition
All patients with OSA and controls underwent three-dimensional T1-weighted MRI on a 3 T MRI scanner with the following acquisition parameters: TI = 1300 ms, TR/TE = 8.6/3.96 ms, flip angle = 8°, and isotropic voxel size = 1 mm3. To rule out structural lesions, they were scanned using standard brain MRI protocols, including FLAIR and T2-weighted imaging.
Calculation of Limbic Structure Volumes
The limbic structures were analyzed volumetrically using the development version of FreeSurfer program with the following steps. First, we used the FreeSurfer “recon-all” commandReference Dale, Fischl and Sereno33 to process our three-dimensional T1-weighted MRI data. Using this command, we could obtain the volumes of the hippocampus, amygdala, and thalamus. Second, we used “mri_sclimbic_seg”Reference Greve, Billot and Cordero27 scripts to segment limbic structures and obtain their absolute volumes, including the mammary body, hypothalamus, basal forebrain, septal nuclei, fornix, and nucleus accumbens. This method used a U-net-based deep learning algorithm. All segmentations were visually inspected for accuracy prior to inclusion in the group analysis to correct for a potential error in the automated procedure. Figure 1 illustrates an example of segmentation in limbic structures in a patient. Third, we corrected the limbic structure volumes for their estimated intracranial volumes.
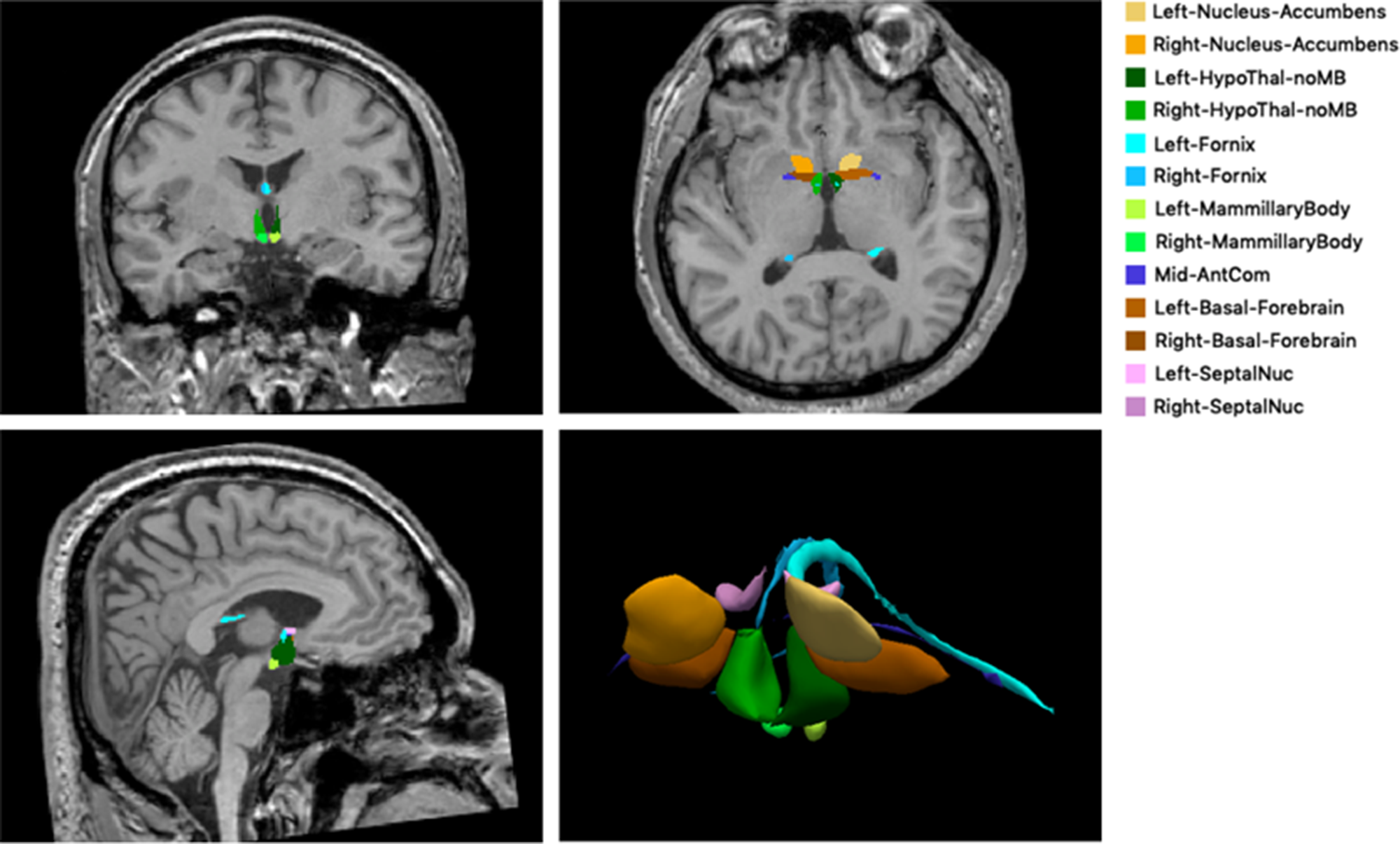
Figure 1: The example of segmentation of subcortical limbic structures. The segmentations are overlaid onto a T1-weighted image in coronal, axial, and sagittal orientation and shown in volume rendering. HypoThal-noMB: hypothalamus, AntCom: anterior commissure, SeptalNuc: septal nucleus.
Calculation of Intrinsic Limbic Network
We examined the intrinsic limbic network in patients with OSA and healthy controls using the Brain Analysis with Graph Theory (BRAPH) program.Reference Mijalkov, Kakaei, Pereira, Westman and Volpe34 This software develops a collection of nodes representing brain regions (individual volumes within limbic structures) and edges representing their connections (calculated as partial correlation coefficients between each pair of brain regions while controlling for age and sex effects) for each group. Each group was assigned a weighted, undirected connection matrix. We applied graph theory to determine the differences in the intrinsic limbic network between the groups using network measures such as average degree, average strength, radius, diameter, eccentricity, characteristic path length, global efficiency, local efficiency, mean clustering coefficient, transitivity, modularity, assortativity, and small-worldness index.Reference Farahani, Karwowski and Lighthall35–Reference Park, Lee and Shin37 These network parameters were compared between patients with OSA and healthy controls.
Statistical Analysis
The age and sex were compared using the chi-squared test and the Student’s t-test, respectively, between the patients with OSA and healthy controls. We used Student’s t-test to compare limbic structure volumes between the groups. We used nonparametric permutation tests with 1000 permutations to determine the statistical significance of the differences between the groups in the intrinsic limbic network, because we could obtain network measures at the group level through the BRAPH program data. We defined statistical significance as a p-value less than 0.05 for comparing baseline characteristics and correlations between the groups. All statistical analyses were carried out using MedCalc® Statistical Software, version 20.022 (MedCalc Software Ltd, Ostend, Belgium; https://www.medcalc.org; 2021).
Results
Clinical and Polysomnographic Characteristics
We enrolled 26 patients with OSA and 30 healthy controls. The groups did not differ by age and sex. Table 1 shows the clinical and polysomnographic characteristics of patients with OSA and healthy controls.
Table 1: The clinical and polysomnographic characteristics in the patients with obstructive sleep apnea

SD=standard deviation; BMI=body mass index; AHI=apnea-hypopnea index; RDI=respiratory disturbance index.
The Differences in Limbic Structure Volumes Between Patients with OSA and Healthy Controls
Table 2 reveals the differences in limbic structure volumes between patients with OSA and healthy controls. Significant differences existed between the groups' volumes of some limbic structures. The volumes in the right amygdala, right hippocampus, right hypothalamus, right nucleus accumbens, left amygdala, left basal forebrain, left hippocampus, left hypothalamus, and left nucleus accumbens in patients with OSA were lower than those in healthy controls.
Table 2: The differences in limbic structure volumes between patients with OSA and healthy controls

* p < 0.05.
OSA=obstructive sleep apnea.
The Differences in Limbic Structure Volumes Between Patients with NREM-Predominant OSA and REM-Predominant OSA
Eight patients were NREM-predominant OSA, whereas five patients were REM-predominant OSA. There were no significant differences in the limbic structures’ volumes, including right and left amygdala, basal forebrain, fornix, hippocampus, hypothalamus, mammary body, nucleus accumbens, septal nuclei, and thalamus between the groups (Suppl. 1).
The Differences in Intrinsic Limbic Network Between Patients with OSA and Healthy Controls
Table 3 shows the differences in the intrinsic limbic network between patients with OSA and healthy controls. There were no significant differences in network measures, including average degree, average strength, radius, diameter, eccentricity, characteristics path length, global efficiency, local efficiency, mean clustering coefficient, transitivity, modularity, assortativity, and small-worldness index, between the groups.
Table 3: The differences in the intrinsic limbic network between patients with OSA and healthy controls

OSA=obstructive sleep apnea; CI=95% confidence interval of difference.
Correlation Between Clinical and Polysomnographic Characteristics and Limbic Structure Volumes
We conducted a correlation analysis between the limbic structure volumes, including the right amygdala, right hippocampus, right hypothalamus, right nucleus accumbens, left amygdala, left basal forebrain, left hippocampus, left hypothalamus, and left nucleus accumbens, and clinical and polysomnographic characteristics in patients with OSA. There was a significant negative correlation between volumes in the right amygdala, right nucleus accumbens, left amygdala, and left nucleus accumbens and age. However, there were no significant correlations between the limbic structure volumes, including the right hippocampus, right hypothalamus, left basal forebrain, left hippocampus, and left hypothalamus and the other clinical and polysomnographic characteristics (Table 4). Furthermore, we conducted a correlation analysis between the limbic structure volumes and age in the healthy controls, which showed no significant correlations between them (Suppl. 2.).
Table 4: The results of correlation analysis between clinical and polysomnographic characteristics and limbic structures volumes in the patients with obstructive sleep apnea

* p < 0.05.
BMI=body mass index; AHI=apnea-hypopnea index; RDI=respiratory disturbance index.
Discussion
We found differences between cases and controls in the limbic structure volumes of the right amygdala, right hippocampus, right hypothalamus, right nucleus accumbens, left amygdala, left basal forebrain, left hippocampus, left hypothalamus, and left nucleus accumbens using a U-net-based deep learning algorithm.Reference Greve, Billot and Cordero27 We also found no alterations of the intrinsic limbic networks in patients with OSA compared to healthy controls, which was analyzed based on the graph theory.
The volumes of the right amygdala, right hippocampus, right hypothalamus, right nucleus accumbens, left amygdala, left basal forebrain, left hippocampus, left hypothalamus, and left nucleus accumbens were significantly lower in patients with OSA than in the controls. This finding was consistent with a previous meta-analysis, which showed structural atrophy in the basolateral amygdala, hippocampus, and insular cortex in patients with OSA.Reference Tahmasian, Rosenzweig and Eickhoff24 These findings suggest the important role of the amygdala, hippocampus, and insula in abnormal emotional and sensory processing in patients with OSA. The right amygdala is thought to mediate aversive conditioning to errors, while the left amygdala is believed to underpin negative performance affect.Reference Polli, Wright and Milad38 Synaptic plasticity in the basolateral amygdala is shown to mediate the acquisition of associative memories of both ends of emotional valences, and different populations of neurons in that complex may encode fearful or rewarding associations.Reference Namburi, Beyeler and Yorozu39 In major depressive disorder, abnormal functional connectivity of the amygdala and hippocampus may interact with dysfunctional intrinsic network activity, which could underlie emotional memory disturbances in patients with OSA.Reference Tahmasian, Knight and Manoliu40 Thus, the findings with a decrease in amygdala volume in patients with OSA may suggest that this role of amygdala may have declined in patients with OSA.Reference Tahmasian, Shao and Meng41
Furthermore, the hippocampus is particularly vulnerable to intermittent hypoxia, which could explain the high frequency of neurobehavioral deficits in patients with OSA.Reference Gozal, Row, Schurr and Gozal42 A recent study discovered a link between OSA and AD. Cognitive impairments observed in patients with OSA could be partly explained by hippocampal dysfunction, as previously demonstrated in patients with AD.Reference Tahmasian, Pasquini and Scherr43 In addition, another study discovered that patients with AD were five times more likely than healthy controls to develop OSA symptoms.Reference Emamian, Khazaie and Tahmasian19 The right hippocampus is known to be involved in memory tasks that require concentric spatial location processing, which could impair driving ability in patients with OSA.Reference Iglói, Doeller, Berthoz, Rondi-Reig and Burgess44 This is in a line with the finding of the present study showing the significant difference in hippocampus volume between patients with OSA and healthy controls.
The nucleus accumbens is one forebrain nuclei that play a crucial role in pain modulation and sleep-wake cycle regulation.Reference Oishi and Lazarus45 Dopaminergic activity at the inhibitory D2 receptor reduces nucleus accumbens output, increases arousal, and disrupts sleep status.Reference Qiu, Liu, Qu, Urade, Lu and Huang46 The nucleus accumbens is more activated during forced wakening than during uninterrupted sleep, according to a study on forced wakening by time division.Reference Seminowicz, Remeniuk and Krimmel47 In this study, the nucleus accumbens volume in patients with OSA was lower than that in the healthy controls, which may be related to poor sleep quality in OSA. Changes in the nucleus accumbens caused by forced awakening may be linked to sleep fragmentation, affecting cognitive impairments in patients with OSA. These findings suggested that changes in the limbic structure volumes in patients with OSA are related to developing cognitive impairments.
However, our study revealed no differences in the intrinsic limbic network between patients with OSA and a healthy control group. The present results differed from previous studies that analyzed the entire brain network. In one study, researchers investigated structural brain connectivity using diffusion tensor imaging and discovered that white matter abnormalities in patients with OSA caused changes in structural connectivity.Reference Lee, Yun and Min48 Another study found that OSA caused changes in global topological characteristics in the brain network, demonstrated by statistical cortical volume associations.Reference Y-g, Wang and Liu49 There are several reasons for different results. A plausible explanation is that the intrinsic limbic network is likely to differ from the global brain network, which we did not analyze. Another possibility is that our small sample size had insufficient power to detect a difference. Further research with larger sample sizes is needed to confirm our findings.
There were some limitations in this study. First, this study was limited to a single center and relatively small sample size, limiting generalizability. Second, a temporal relationship could not be determined because this was a retrospective study comparing patients with OSA and healthy controls. As a result, it was unclear whether the change in limbic structure volumes was the result or cause of OSA. Third, we included the control group without polysomnographic examination and may have included individuals with undiagnosed sleep apnea. Lastly, since limbic structures were very small, it was difficult to completely rule out the possibility of errors in segmentation. However, we used the toolbox based on the U-Net for segmentation of limbic structures, which was one of the recent machine learning algorithms. It had been trained using 39 manually labeled MRI data sets for spatial, intensity, contrast, and noise augmentation. Test–retest reliability of the tool was already proven.Reference Greve, Billot and Cordero27 Nevertheless, this was the first study to focus on changes in limbic structural volumes and intrinsic limbic networks based on the graph theory in patients with OSA compared to healthy controls. Significant volume changes in the several limbic structures were successfully confirmed.
Conclusion
We demonstrate that the volumes of several limbic structures in patients with OSA are significantly lower than those in healthy controls. However, there are no alterations to the intrinsic limbic network. These findings suggest that OSA is one of the risk factors for cognitive impairments.
Supplementary material
For supplementary material accompanying this paper visit https://doi.org/10.1017/cjn.2022.303
Acknowledgement
This work was supported by the 2022 Inje University Busan Paik Hospital Research Grant.
Conflict of Interest
The authors declare no conflict of interest.
Statement of Authorship
Conception and design: Kang Min Park and Jinseung Kim. Acquisition of data, analysis, and interpretation of data: Kang Min Park and Jinseung Kim.