Fe deficiency (ID) is the most common aetiological factor for anaemia globally, and between 35 and 65 % of the anaemia burden in low-income regions in Africa, Asia and Latin America is attributable to ID( Reference Kassebaum, Jasrasaria and Naghavi 1 ). Food fortification is regarded as a safe and cost-effective approach to counteract and prevent ID as long as the targeted populations consume significant quantities of industrially manufactured fortified foods( Reference Hurrell, Ranum and de Pee 2 ). Condiments are promising vehicles for fortification as they are among the very few regularly purchased food items in resource-poor areas( Reference Zimmermann, Wegmueller and Zeder 3 ), and Fe-fortified condiments have been shown to be efficacious in improving Fe status( Reference Ballot, Macphail and Bothwell 4 – Reference Andersson, Thankachan and Muthayya 7 ).
Bouillon cubes are a promising vehicle for Fe fortification, particularly in sub-Saharan Africa where previous national surveys have reported a high proportion of Burkinabe, Cameroonian, Nigerian, Senegalese and Ivorian women (79–96 %) who consumed bouillon cubes regularly( Reference Engle-Stone, Ndjebayi and Nankap 8 – Reference Staubli-Asobayire 10 ). However, ensuring the bioavailability of Fe and the stability of the cubes in these climates is challenging. Water-soluble Fe compounds such as ferrous sulphate (FeSO4) provide the most bioavailable Fe but induce oxidative rancidity noticeable to consumers at very low levels( Reference Bovell-Benjamin, Viteri and Allen 11 ) as well as other unwanted sensorial defects of the product in terms of colour, taste or flavour. In addition, ionic Fe may cause protein precipitation or interact with compounds in the food matrix causing colour changes when the cube is used as a condiment( Reference Hurrell 12 ). Ferric pyrophosphate (FePP) is a poorly water-soluble Fe compound that is white and minimally affects sensory properties of fortified foods; its addition to food vehicles produces negligible colour change( Reference Moretti, Lee and Zimmermann 13 ). However, FePP in general is less than half as well absorbed as FeSO4 in humans( Reference Hurrell, Furniss and Burri 14 , Reference Hurrell, Reddy and Dassenko 15 ). Nevertheless, the efficacy of FePP to reduce anaemia or ID has been shown in several randomised controlled trials( Reference Zimmermann, Wegmueller and Zeder 3 , Reference Zimmermann, Wegmueller and Zeder 6 , Reference Moretti, Zimmermann and Muthayya 16 – Reference Wegmuller, Camara and Zimmermann 18 ). Limited evidence exists on potential enhancers of Fe bioavailability from FePP. Addition of ascorbic acid has been shown to increase Fe bioavailability from FePP( Reference Fidler, Davidsson and Zeder 19 ), but its use in FePP-fortified foods, which are usually cooked, is limited because of the susceptibility to oxidation of vitamin C into inactive compounds when exposed to air or during heat treatment( Reference Hurrell, Lynch and Bothwell 20 ).
Tetra sodium pyrophosphate (NaPP) is a food additive of the complexing agents family that binds minerals and acts as a water ligand( Reference Belitz, Grosch and Schieberle 21 ). This binding effect has a positive effect on the sensorial product quality over time. In addition, pyrophosphates such as in NaPP may interact at specific ratios with Fe, as in FePP, forming soluble complexes that remain in solution at neutral pH( Reference Tian, Blanco and Smoukov 22 ). This suggests that presence of such soluble Fe complexes could increase in vivo Fe bioavailability. Therefore, to investigate whether NaPP has the potential to enhance Fe bioavailability, we conducted in vitro bioavailability studies followed by an in vivo absorption study with bouillon cubes fortified with either FePP only, FePP+NaPP, FeSO4 only or FeSO4+NaPP. The in vitro studies followed a protocol of simulated digestion investigating dialysable and ionic Fe release, and included the cellular ferritin response of the simulated digestion in a Caco-2 cell model. In vivo Fe absorption was assessed in Fe-deficient women using bouillon cubes that were intrinsically labelled with stable Fe isotopes and by assessing Fe incorporation of stable isotopic labels at least 14 d after administration.
Methods
Subjects
The present study was carried out among the student and staff population of ETH Zurich and University Zurich, Switzerland. In all, twenty-four, non-pregnant, non-lactating women with ID (plasma ferritin (PF)<15 µg/l), aged between 18 and 27 years with body weight <66 kg, were selected from an initial screening of 178 women. Women with known metabolic, chronic and gastro-intestinal diseases, as well as women on long-term medication (except oral contraceptives), were excluded from participating. In addition, smokers, women who did more than 10 h of intensive sport per week and women who consumed more than fourteen units of alcohol per week were not enrolled. Intake of vitamin and/or mineral supplements was not allowed during and 2 weeks before the study. No women were recruited who had donated blood or experienced substantial blood loss within 6 months of the beginning of the study. The study was conducted according to the guidelines laid down in the Declaration of Helsinki, and all procedures involving human subjects were approved by the cantonal ethics committee in Zurich, Switzerland (approval no. 2014-0279). Written informed consent was obtained from all participants. The study was registered at clinicaltrials.gov (NCT02327299; https://clinicaltrials.gov/ct2/show/NCT02327299).
Absorption study design
A double-blind, randomised, partial William’s cross-over design was used with each woman serving as her own control. Participants received one of four different types of bouillon containing either 54FeSO4, 54FeSO4+NaPP, 57FePP or 57FePP+NaPP on study days 1, 4, 18 and 21, respectively (Fig. 1). The William’s cross-over design was partial because the same Fe compounds were labelled with the same isotopes, and could therefore not be administered consecutively on days 1 and 4 or on days 18 and 21. Nevertheless, each of the four bouillon types could have been administered on any study day if some sequences were avoided (e.g. 54FeSO4-fortified bouillon was administered on days 1, 4, 18 or 21 but then 54FeSO4-fortified bouillon with NaPP was not given on days 4, 1, 21 or 18, respectively). The labelled Fe-fortified bouillons made from two cubes according to a standard protocol were administered between 06.30 and 09.30 hours after an overnight fast. The participants consumed the complete bouillon (400 ml) and a glass of 160 ml nanopure water in the presence of the investigators and were not allowed to eat for 3 h after consuming the bouillon. Within 1 h after meal completion, participants were allowed to drink 330 litres of mineral water (Evian Junior; Evian) provided by the investigators. No other fluids were allowed for 3 h after completion of the bouillon.

Fig. 1 Schematic diagram of the study design. Four different bouillons containing either ferric pyrophosphate (FePP) only, FePP+tetra sodium pyrophosphate (NaPP), ferrous sulphate (FeSO4) only or FeSO4+NaPP were randomly administered on days 1, 4, 18 or 21, respectively. FePP was labelled with 57Fe isotopes and FeSO4 with 54Fe isotopes; therefore, a partial William’s cross-over design was used, meaning that the same isotopes were not administered consecutively on days 1 and 4 or on days 18 and 21, respectively. In all, twenty-four women from an initial screening of 178 women were selected; there were no dropouts. Redundant participants were participants who were eligible but were not included in the study because the targeted sample size was already reached. The participants were included according to their screening number, meaning that the first twenty-four participants with iron deficiency were included, whereas the additional iron-deficient participants were redundant and not included.
During screening (baseline measurements), 19–11 d before the first bouillon administration, body weight and height of the participants were measured, and a first blood sample was drawn for Fe status determination (Hb, PF, soluble transferrin receptor (sTfR), C-reactive protein (CRP), α-1-glycoprotein (AGP)). On day 18, a second blood sample was collected for determining Fe status and Fe absorption from the administration of the first and second bouillons. On day 35 (final study day), a third blood sample was drawn for Fe isotopic analysis and determination of Fe status. Fe absorption was determined using stable isotope technique, in which the incorporation into erythrocytes of isotopic Fe labels was measured at least 14 d after the administration of the last bouillon( Reference Walczyk, Davidsson and Zavaleta 23 ). Adverse events and concomitant medication were inquired and documented during the entire study after subject enrolment.
Preparation of bouillon
Each bouillon cube (4 g) was intrinsically labelled with 2·5 mg Fe isotopes as the only source of Fe in the cube. Preparation of bouillon started with boiling nanopur water in a water boiler. Next, 400 g boiling nanopur water was weighed into a heat-resistant glass beaker, and two bouillon cubes (two cube weighing total 8 g, containing 5 mg Fe isotopes) were dissolved in the water. The vial, used for storage and transport of the bouillon cubes, was rinsed with 1 ml boiling nanopur water to dissolve any remaining particles and the rinsing was added to the beaker. Bouillon cubes were dissolved by stirring using a stainless steel spoon until no visible cube particles were left. The bouillon was served to the participants when it had cooled down to a temperature of 58±2°C, measured using an infrared thermometer. The empty beaker was rinsed two times with 20 ml boiled nanopur water, and the rinsing liquid was consumed by the participants. To ensure complete intake of bouillon, participants were instructed to clean the stainless steel spoon by licking it and to use a plastic scraper to remove and consume the remaining particles at the bottom of the beaker. After complete consumption of the bouillon, the participants immediately consumed 160 g nanopur water.
Production of isotopically labelled bouillon cubes
Isotopically labelled 54FeSO4 (monohydrate crystals) and 57FePP with a volume-weighed mean diameter D[4,3] of 18·1 µm and a surface-weighed mean diameter D[3,2] of 14·6 µm were prepared in powder form by Paul Lohmann GmbH from isotopically enriched elemental Fe (54Fe-metal: 99·7 % enriched; 57Fe-metal: 96·7 % enriched; all Chemgas).
Bouillon cubes were prepared from the ingredients as in the equivalent commercial product (salt, sugars, starch, vegetable fats, herbs and spices) using a dry-blending protocol at 300 g scale. Ingredients were added in the order of quantity required, starting with the largest amount by weight (salt), to a small stainless steel bowl blender with K-mixer (Kenwood). The isotopically labelled material (2·5 mg Fe/cube) and the NaPP (Na4P2O7; 12 mg/cube) were added in the final blending step, resulting in approximately 1:1 molar ratio for equivalents (eq.). NaPP:Fe. Blend homogeneity was monitored by visual inspection. From the blend, 4000 mg was accurately weighed (±10 mg at maximum) and transferred to a stainless steel die for immediate pressing with a fixed end-pressure to obtain bouillon cubes (14×14×14 mm). Dose and uniformity of dose were checked via the total cube weight variation and by multiple, randomly selected cube Fe analysis.
Measurement of iron in the bouillon cubes
The Fe concentration and isotopic composition of each type of labelled bouillon cubes was determined in two bouillon cubes, corresponding to the administered dose. Doses of two cubes were mineralised in triplicate by boiling in 50 ml nitric acid 65 % for 12 h, followed by the addition of 3 ml hydrogen peroxide 30 % and further boiling for 2 h. The solutions were then diluted to 300 g with water. Fe concentration in aliquots of the mineralised samples was determined by inverse isotope dilution mass spectrometry using an Fe standard solution prepared gravimetrically from an Fe isotopic reference material (IRMM-014; EU Institute of Reference Materials). Fe and isotopic label concentrations of two bouillon cubes were expressed as means and standard deviations and used for the calculation of Fe bioavailability.
Blood sample analysis
Hb was measured in whole blood on the day of collection using a Hemocue during Screening and an automated hematology analyzer (Sysmex Corporation) for follow-up blood samples; anaemia was defined as Hb<120 g/l( 24 ). PF was measured using an IMMULITE automatic system (Siemens); ID was defined as PF<15 μg/l and ID anaemia as Hb<120 g/l plus PF<15 μg/l( 24 ). STfR, AGP and CRP were measured using a combined Sandwich ELISA technique( Reference Erhardt, Estes and Pfeiffer 25 ); sTfR concentrations >8·3 mg/l were considered as second-stage ID, indicating Fe-deficient erythropoiesis. Expected AGP and CRP concentrations for healthy individuals were <1 g/l and <5 mg/l, respectively( Reference Dati, Schumann and Thomas 26 ).
Each isotopically enriched blood sample was analysed in duplicate for its isotopic composition. Whole blood was mineralised by microwave digestion, and Fe was separated by anion-exchange chromatography and a subsequent precipitation step with ammonium hydroxide( Reference Hotz, Krayenbuehl and Walczyk 27 ). Fe isotope ratios were determined by an MC-ICP-MS instrument (Neptune; Thermo Finnigan).
Calculation of iron bioavailability
The amounts of 54Fe and 57Fe labels in the blood were calculated on the basis of the shift in Fe isotope ratios in the blood samples collected on days 18 and 35 and the estimated amount of Fe circulating in the body. For the calculation on day 35, the isotopic ratio of day 18 was considered as a new baseline. The changes in circulating isotopic tracer in blood between 14 and at least 35 d after administration are negligible (unpublished internal data partly based on the study by Petry et al.( Reference Petry, Rohner and Gahutu 28 )), particularly when taking into account the randomisation of the participants. Therefore, a first dose of isotopes does not affect the bioavailability calculation of a second dose of isotopes, administered at least 14 d after the first dose. Circulating Fe was calculated on the basis of the blood volume estimated from height and weight and measured Hb concentration( Reference Brown, Hopper and Hodges 29 ). The calculations were based on the principles of isotope dilution, taking into account that Fe isotopic labels were not monoisotopic, using the methods described by Kastenmayer et al.( Reference Kastenmayer, Davidsson and Galan 30 ). For calculating Fe bioavailability (fractional Fe absorption), 80 % incorporation of the absorbed Fe into erythrocytes was assumed( Reference Hosain, Marsaglia and Noyes 31 ).
In vitro studies
In vitro bioaccessible Fe was determined using previously described methods( Reference Miller, Schricker and Rasmussen 32 , Reference Luten, Crews and Flynn 33 ) and are described in detail in the online Supplementary Material. In brief, the experiments were carried out in triplicate and were based on simulating gastrointestinal digestion, by exposure of 80 ml bouillons (Fe concentration: 18·75 µg/ml) to gastric conditions (low pH and gastric enzymes) followed by intestinal conditions (neutralisation of pH and incubation with pancreatic enzymes and bile salts). Subsequently, the digested bouillon was dialysed (<8000 g/mol) and the dialysable Fe and ionic Fe, using inductively coupled plasma-atomic emission spectrometry and the Ferrozine method( Reference Viollier, Inglett and Hunter 34 ) with slight alterations to derive a linear response equation, respectively, were determined.
To simulate the cellular absorption of Fe after gastrointestinal digestion, uptake of Fe by Caco-2 cell monolayers was used. Exposed to ionic Fe, Caco-2 cells synthesise ferritin as response to Fe uptake and the amount is proportional to the Fe content in the culture medium. Cellular ferritin can be a good indicator of the Fe absorbed( Reference Glahn, Lee and Yeung 35 ). Ferritin was measured via a commercially available ELISA kit (H-ferritin (human), ELISA kit, Abnova KA0211; Abnova GmbH). A control without added Fe and a reference sample (5 µm-FeSO4 solution) were included to determine the cell blank and standard responses to confirm validity of the assay.
Statistical analysis
Analyses were conducted with SAS software version 9.4 and Excel (Windows 7; Microsoft). Results of Fe analysis, age, anthropometric features, Hb, PF, sTfR, AGP and CRP were presented as means and standard deviations if normally distributed. If not normally distributed, the results were presented as geometric mean values with their 95 % CI. The results of Fe absorption are presented as geometric mean values with their 95 % CI. The study was powered (90 %) to detect an intra-subject difference of 30 % in Fe bioavailability with a two-sided α set at 0·05, and sample size calculation was based on the primary hypothesis for the in vivo study, which was the comparison of FePP-fortified cubes with and without NaPP. Nevertheless, all the differently fortified bouillon cubes were compared using a mixed model ANOVA on the log-transformed Fe bioavailability with subject as a random effect and day of treatment and presence of stabiliser as fixed effects followed by Tukey’s adjustment to control the type 1 error to 0·05 over the set of the six potential comparisons; two-sided P values are reported. Fe concentrations in the differently fortified bouillon cubes, dialysable and ionic Fe and ferritin response for the differently fortified bouillon cubes were compared by one-factor ANOVA followed by Bonferroni-corrected multiple comparisons. Differences were considered significant at P<0·05. All data were converted to their logarithms for statistical analysis and reconverted for reporting.
Results
Participant characteristics
Data of twenty-four women were included in the in vivo study. At baseline, all women had PF concentrations<15 µg/l and normal CRP concentrations (<5 mg/l) (Table 1); three of the women were anaemic at baseline and six women had Fe-deficient erythropoiesis as indicated by elevated sTfR concentrations. Among all, two women had slightly elevated AGP values at baseline (1·23 and 1·01 g/l), which then decreased <1·0 g/l during the study.
Table 1 Age, anthropometric features and Hb, plasma ferritin (PF), soluble transferrin receptor (sTfR), α-1-acid glycoprotein (AGP) and C-reactive protein (CRP) concentrations of the participating iron-deficient adult women at baseline (Mean values and standard deviations; geometric means and 95 % confidence intervals)

At the second blood sampling, PF concentrations of nine women increased to above 15 μg/l. The PF values for these nine women were between 15·1 and 26·8 μg/l. At the end point, fourteen women had PF concentrations <15 μg/l and twenty women <20 μg/l. One woman had slightly elevated CRP concentrations in her second blood sample (6·2 mg/l), and two women had CRP concentrations >5 mg/l at the end point (5·7 and 15·3 mg/l).
Iron concentration of bouillon cubes
The average Fe concentrations in the bouillon cubes fortified with FePP were close to the target of 5 mg Fe/2 cubes and showed good content uniformity, whereas the average Fe concentrations in the FeSO4-fortified bouillon cubes were slightly below and above the targeted value. In addition, the variability of Fe concentration measurements was higher in the bouillon cubes fortified with FeSO4 compared with the FePP-fortified bouillon cubes (Table 2).
Table 2 Total iron and isotopic label concentrations of the four different types of intrinsically labelled bouillon cubes (Mean values and standard deviations)
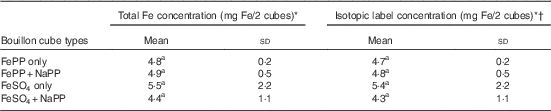
FePP, ferric pyrophosphate; NaPP, sodium pyrophosphate; FeSO4, ferrous sulphate.
a Mean values within a column with unlike superscript letter were significantly different (P<0·05).
* n 3 Independent analyses.
† The isotopic label concentration is part of the total Fe concentration. FePP was labelled with 57Fe isotopes and FeSO4 was labelled with 54Fe isotopes.
In vivo iron bioavailability measurements
Mean Fe bioavailability from FePP-fortified bouillon cubes plus NaPP was 46 % higher than that from FePP-fortified bouillon cubes without NaPP (P<0·005) (Table 3). In all, twenty-one of the twenty-four women showed higher Fe absorption from bouillon cubes fortified with FePP+NaPP than from cubes fortified with FePP only. Mean Fe bioavailability from bouillon cubes fortified with FeSO4+NaPP did not significantly differ compared with that from FeSO4-fortified bouillon cubes without NaPP (P=0·2). The relative bioavailability (RBV) of FePP-fortified bouillon cubes with or without NaPP was <20 %, and the mean Fe bioavailability was between 4·3 and 7·7 times lower than that from bouillon cubes fortified with FeSO4 with or without NaPP, respectively (all P<0·0001).
Table 3 Iron bioavailability and relative bioavailability per different type of bouillon cubes consumed by iron-deficient women (Geometric mean values and 95 % confidence intervals)

FePP, ferric pyrophosphate; NaPP, sodium pyrophosphate; FeSO4, ferrous sulphate.
a,b,c Mean values within a column with unlike superscript letters were significantly different (P<0·0001).
* Relative to the bioavailability from FeSO4 only (100 %).
In vitro iron measurements
The in vitro Fe accessibility by the digestion protocol showed a clear effect of the addition of the stabiliser for FePP, but not for FeSO4 (Fig. 2). The dialysable Fe (P=0·008) and ionic Fe (P=0·012) were significantly higher for cubes fortified with FePP+NaPP (1·36 (sd 0·23) mg/l and 34·7 (sd 3·2) %, respectively) than for FePP only (0·85 (sd 0·08) mg/l and 18·7 (sd 5·3) %, respectively). The dialysable Fe for FeSO4 only (2·33 (sd 0·25) mg/l) and FeSO4+NaPP (3·13 (sd 0·11) mg/l) was significantly higher than that from FePP+NaPP and from FePP (P<0·005). This was different for the ionic Fe where FePP+NaPP was not different from FeSO4 only (39·4 (sd 5·8) %) and FeSO4+NaPP (50·6 (sd 4·4) %).

Fig. 2 Results of the in vitro experiments for the four different bouillons containing either ferric pyrophosphate (FePP) only, FePP+tetra sodium pyrophosphate (NaPP), ferrous sulphate (FeSO4) only or FeSO4+NaPP. Bars represent dialysable (A) and ionic Fe (B) from simulated gastric and small intestinal digestion and ferritin response (C) from Caco-2-cell assay. Error bars with whiskers indicate standard deviations (n 3). a,b,c Mean values with unlike letters were significantly different in an one-way ANOVA followed by Bonferroni correction for multiple comparison (P<0·05).
The cellular ferritin responses generally showed higher variability (Fig. 2), and bouillons with FePP only (7·8 (sd 2·2) ng ferritin/mg protein) did not differ from FePP+NaPP (12·0 (sd 3·7) ng/mg). The only significant difference was found for FeSO4+NaPP (40·8 (sd 14·8) ng/mg) compared with FePP only (P=0·008) and FePP+NaPP (P=0·046). There was a tendency for an increased ferritin response for FeSO4+NaPP compared with FeSO4 only (14·4 (sd 7·0) ng/mg; P=0·076).
Discussion
The major finding of the current study is the enhancing effect of NaPP on Fe absorption from bouillon cubes fortified with FePP. To our knowledge, this is the first time that the effect of NaPP on Fe bioavailability has been investigated and NaPP has never been reported as an enhancer of Fe absorption. Previous studies have shown that Fe bioavailability from FePP-fortified infant cereals can be enhanced by the addition of ascorbic acid, but to a lesser extent than from FeSO4 ( Reference Fidler, Davidsson and Zeder 19 , Reference Moretti, Zimmermann and Wegmuller 36 ). Whereas the enhancing effect of ascorbic acid on human non-haem Fe absorption is related to the reducing and chelating properties of ascorbic acid during digestion of the food( Reference Hurrell, Lynch and Bothwell 20 ), our in vitro experiments suggest that NaPP may enhance bioavailability by forming soluble complexes of Fe and pyrophosphate anions, such as P2O7 4−and HP2O7 3−, hence increasing the amount of soluble ionic Fe, which is essential for intestinal Fe uptake. A previous study suggests that these complexes still exist at the neutral pH of the gastro-intestinal milieu. This is in contrast to most of the other ionic Fe compounds that form insoluble complexes at neutral pH( Reference Tian, Blanco and Smoukov 22 ).
The enhancing effect of NaPP on Fe absorption from FePP suggests that the addition of this salt could be used as an enhancer in other foods preferentially fortified with FePP, such as extruded rice, or infant cereals. In these foods, NaPP could enhance the low Fe bioavailability from FePP significantly. However, we tested the enhancing effect of NaPP on FePP using a comparably simple test (bouillon cubes dissolved in water) containing no or negligible amounts of Fe absorption inhibitors and only at one fixed molar ratio (approximately 1:1 for eq. NaPP:Fe). In vitro data investigating the dissolution behaviour of FePP in the presence of NaPP suggest that a higher ratio of NaPP:FePP (>1 eq.) could further improve the Fe bioavailability, because multiple pyrophosphate ligands per Fe cation would be available to form soluble complexes( Reference Tian, Blanco and Smoukov 22 ). Food matrix is known to affect bioavailability of FePP( Reference Moretti, Zimmermann and Wegmuller 36 ), and in complete meals, containing phytic acid, polyphenols and other common food ingredients known to inhibit Fe absorption( Reference Hurrell 37 – Reference Armah, Carriquiry and Sullivan 39 ), the enhancing effect of NaPP may be modulated by the food matrix. Therefore, further investigation is needed to understand NaPP effects. In the present study, NaPP at the tested ratio did not increase the Fe bioavailability of FeSO4; nevertheless, it would be of interest to investigate whether NaPP has an enhancing effect of Fe absorption from other Fe compounds, particularly the water-insoluble compounds.
The current study using FePP with a regular mean particle size, comparable with the commercially available FePP, confirms the rather low bioavailability of FePP found in previous human studies, where Fe bioavailability from FePP with regular particle size was significantly lower than that from other Fe compounds used to fortify infant cereals( Reference Davidsson, Kastenmayer and Szajewska 40 ) or full-cream milk powder( Reference Walczyk, Kastenmayer and Storcksdieck Genannt Bonsmann 41 ). On the basis of a human study using FePP with a markedly reduced average particle size (D[3,2]=0·30 µm), where Fe bioavailability from FePP-fortified infant cereals and yogurt drinks was not different compared with that from FeSO4 ( Reference Fidler, Walczyk and Davidsson 42 ), reducing the particle size of FePP has been suggested to potentially increase its bioavailability. However, in another study, micronised dispersible FePP with D[3,2] of 0·77 µm had a lower Fe bioavailability from infant cereals and rice than FeSO4 despite the small particle size( Reference Moretti, Zimmermann and Wegmuller 36 ). Owing to the difficulties in manufacturing labelled compounds with small particle size that closely mimic the commercially available compounds, it remains unclear whether the reduction of the particle size would further increase the bioavailability from FePP. The distinctly reduced RBV of FePP to FeSO4 in the present study is mainly due to the insolubility of FePP, but at some extent can also be explained by the low Fe status of the participating women as the RBV of FePP decreases with decreasing Fe status( Reference Moretti, Zimmermann and Wegmuller 36 ) as a result of the up-regulated bioavailability of FeSO4 in subjects with low or absent Fe stores( Reference Zimmermann, Biebinger and Egli 43 ). Whether the RBV of FePP from fortified bouillon cubes would be different in more complex meals due to the presence of enhancers and inhibitors, which would differently affect the bioavailability of FePP and FeSO4, needs further investigation.
In sub-Saharan Africa, bouillon cubes are reported to be consumed frequently, and the estimated median intakes in women in Burkina Faso, Cameroon, Niger and Senegal ranged between 2·1 and 4·3 and between 0·7 and 3·6 g/d in urban and non-urban areas, respectively( Reference Hess, Brown and Sablah 9 ). Our study suggests that in populations where the daily consumption of bouillon cubes is approximately 4 g/d per capita, cubes fortified with 2·5 mg Fe could provide between 0·7 and 0·9 mg Fe when fortified with FeSO4 and between 0·1 and 0·2 mg when fortified with FePP, which is between 48 and 58 % and between 7 and 11 % of the median daily Fe requirements for menstruating women older than 18 years( 44 ), respectively. These estimations indicate that higher fortification levels of FePP or higher intakes of FePP-fortified cubes than the current estimated median intake of bouillon cubes may be required to cover 20–30 % of the Fe requirements in women older than 18 years. In addition, it has to be stressed that extrapolation of our data is limited, as in sub-Saharan Africa bouillon cubes are used in complex meals that likely contain considerable amounts of Fe absorption inhibitors, such as phytic acid and polyphenols( Reference Cercamondi, Icard-Verniere and Egli 45 ), and therefore Fe bioavailability is likely to be lower than what has been measured in the present study. Therefore, our data suggest that FeSO4 would provide the highest amount of Fe and may be the preferred fortificant for bouillon cubes. However, product stability and sensorial characteristics are insufficient when using FeSO4, and therefore FePP likely remains the Fe compound of choice for bouillon cubes. With regard to Na intake, which because of the risk of hypertension is a point of concern in savoury consumer products, the addition of NaPP as absorption enhancer in the present study only minutely increased the Na intake by 12 mg/cube. Compared with the common Na concentration in bouillon cubes (approximately 0·8 g/cube) and the WHO recommendation for daily Na intake (<2 g/d)( 46 ), this would be a negligible increase.
Our combined approach of in vitro and in vivo studies confirms that dialysability and simulated gastrointestinal digestion in Caco-2 cell models can be a useful screening tool to understand factors that may affect Fe absorption( Reference Sandberg 47 ). Whereas the results of the dialysable Fe were in complete agreement with the in vivo results, measurement of in vitro ionic Fe only showed the enhancing effect of NaPP on Fe bioavailability from FePP. These results and the high variability in Caco-2 cell ferritin responses confirm the need to ultimately investigate Fe absorption in humans( Reference Fairweather-Tait, Phillips and Wortley 48 ). Discrepancies in the quantitative assessment between in vitro and in vivo methods assessing Fe bioavailability are well documented and are likely due to factors such as Fe status or dose of administered Fe, which can only be taken into account in in vivo studies( Reference Fairweather-Tait, Lynch and Hotz 49 ). A limitation of the present study was the relatively high variability of Fe concentration in the intrinsically labelled bouillon cubes fortified with FeSO4, and this may have increased measurement error in the bioavailability assessment of the FeSO4-fortified bouillon cubes.
Using intrinsically labelled bouillon cubes, our study shows for the first time that NaPP increases bioavailability of FePP. Although this enhancement does not match the high bioavailability obtained with FeSO4, the use of NaPP as an Fe absorption enhancer for FePP is a promising approach for bouillon cubes. Further research is now needed to investigate the effects of NaPP in composite meals prepared with Fe-fortified bouillon cubes and containing Fe absorption inhibitors and enhancers, in other fortified foods and in the long-term perspective.
Acknowledgements
The authors thank Carole Verhoeven, Unilever R&D, Vlaardingen, The Netherlands, for her contribution to the study, and Jeoffrey Jansen and Monique van der Burg-Koorevaar, both Unilever R&D, Vlaardingen, The Netherlands, for the instrumental help in the production and testing of the bouillon cubes. The authors also thank Laura Hackl, Tanja Barth-Jaeggi and Laura Cascales, all Human Nutrition Laboratory, ETH Zurich, Switzerland, for their assistance during screening.
The present study was financially supported by Unilever R&D, Vlaardingen, the Netherlands. G. S. M. J. E. D., W. P. K., R. v. d. B., R. K. H. and P. M. all employees of Unilever R&D, contributed to the study design, conduct of the study, analysis of data and the preparation of the manuscript. The intrinsically labelled bouillon cubes were prepared by Unilever R&D.
C. I. C., W. P. K., R. K. H., R. v. d. B., G. S. M. J. E. D., M. B. Z. and D. M. designed the study; C. I. C., D. M., C. Z. and G. S. M. J. E. D. conducted the study; C. I. C., C. Z., P. M., G. S. M. J. E. D. analysed the data; C. I. C., G. S. M. J. E. D., M. B. Z. and D. M. wrote the paper; C. I. C., M. B. Z. and D. M. had primary responsibility for final content. All the authors read and approved the final version of the manuscript.
C. I. C., D. M., C. Z. and M. B. Z. have no conflicts of interest. G. S. M. J. E. D., W. P. K., R. v. d. B., R. K. H. and P. M. are all employees of Unilever R&D, which markets bouillon cubes.
Supplementary Material
For supplementary material/s referred to in this article, please visit http://dx.doi.org/10.1017/S0007114516002191