Introduction
Tendons serve to attach soft tissue to bone and are specialised to resist and transmit the forces generated by muscle fibres to the skeletal system (Koob and Summers, Reference Koob and Summers2002). Collagen is the major structural protein of the connective tissue and the most abundant protein of the tendon in which it is found in fibrous state (Birk et al., Reference Birk, Southern, Zycband, Fallon and Trelstad1989). The collagen content is about 70% of the dry matter in the tendon (Nimni and Harkness, Reference Nimni and Harkness1988). There are also certain non-collagenous proteins in tendon, such as osteopontin, osteocalcin and proteoglycans (PGs) (Grover and Shoshan, Reference Grover and Shoshan1980). PGs consist of a core protein with one or more covalently attached glycosaminoglycans (GAGs). Theses GAGs chains are long-linear carbohydrate polymers that are negatively charged under physiological conditions due to the occurrence of sulphate and uronic acid group. Despite their small amount (less than 5% of total dry matter), GAGs could play an important role in the biomechanical properties of tendon. A previous study has shown that GAGs bind the collagen fibril to another, like a molecular bridge, providing interfibrillar connections (Derwin et al., Reference Derwin, Soslowsky, Kimura and Plaas2001). This architecture would suggest their possible role in influencing the mechanical integrity of the tendon structure. It has also been suggested that GAGs and associated PG molecules would be able to transmit forces from one collagen fibril to another (Redaelli et al., Reference Redaelli, Vesentini, Soncini, Vena, Mantero and Montevecchi2003).
Out of a pathological situation, factors such as age of the animal or physical activity can modify the biochemical and biomechanical properties of tendon (Hae Yoon et al., Reference Hae Yoon, Brooks, Kim, Terada and Halper2003). In connective tissues, the main change with ageing is the stiffening of collagen (Gal and Everitt, Reference Gal and Everitt1970). According to Voet and Voet (Reference Voet and Voet2005), ageing induces an increase in the degree of interaction between and within the elementary collagen fibrils. This makes the connective tissue become increasingly resistant to solubilisation (Butzow and Eichhorn, Reference Butzow and Eichhorn1968). These changes in collagen quality and/or quantity could influence the mechanical properties of tendon as expected by Buchanan and Marsh (Reference Buchanan and Marsh2002) who reported that changes in biomechanical properties of tendons are associated with changes in collagen concentration. The total content of GAGs mainly depends on the biomechanical role of tendons. Parry et al. (Reference Parry, Flint, Gillard and Craig1982) report that, in the flexor digitorum profundus tendon of rabbit, the GAGs content vary from 2.3–3.5% to 0.2% of the dry weight on the pressure side and in tensional parts, respectively. Ageing is also normally associated with an increase in total GAGs content in tendons (Derwin et al., Reference Derwin, Soslowsky, Kimura and Plaas2001).
In response to physical activities, tendons have the ability to modulate their structural, biochemical and biomechanical properties to meet specific requirements (Buchanan and Marsh, Reference Buchanan and Marsh2002). Under normal physiologic conditions, there is both static and dynamic tensile loading on collagen molecules and associated GAGs and PGs in tendons. An increase in tensile loading of the tendon will induce a mechanical–chemical transduction leading to modifications in these structural elements (Viidik et al., Reference Viidik, Nielsen and Skalicky1996). Woo et al. (Reference Woo, Ritter and Amiel1980) found an increase in total collagen content of digitorum extensor tendon in pigs with exercised tendons. On the other hand, Hae Yoon et al. (Reference Hae Yoon, Brooks, Kim, Terada and Halper2003) reported that a moderate exercise led to a 20% increase in the total GAGs content in the tendon of chicken.
For consumers and meat processors, the quality of the attachment of muscle to bone can have a particular interest when meat needs to be harvested for processing or eating. This is of particular interest in small animals, such as poultry or rabbits, because whole anatomical parts are generally eaten. In France, fast-growing standard (S) broilers have a poor image because they are often reported to present, mainly in the thigh, a very poor cohesion between meat and bone especially after a safe (or long) time of cooking. On the other hand, more extensive poultry production systems, such as Label Rouge (LR) chickens are considered to have a higher quality of meat associated with a better cohesiveness. In the US, Alvarado et al. (2005) also reported that free-range chicken drumsticks had a stronger attachment to the bone than did the commercial chickens drumsticks.
Standard broiler (S) or Label Rouge (LR) chicken production systems differ in many ways such as genetic origin, feed composition, rearing density, etc. (for a review see Rémignon and Culioli, Reference Rémignon and Culioli1995). Among all these factors, the age at slaughtering (35 to 40 days v. 81 days old for S and LR chickens, respectively) and the access to free-range only for LR chickens may have a direct influence on the structure and development of various tendons. The aim of this study was to verify if those largely different conditions of rearing are sufficient to modify the biomechanical and biochemical properties of two different tendons harvested from S and LR chickens.
Material and methods
Animals
Fifty slow-growing chickens (LR type) and 50 broilers (S type) aged 82 and 41 days, respectively, were conventionally slaughtered in a poultry slaughterhouse and immediately stored at +4°C for 24 h. Within a day, all the carcasses were dissected and the two thigh–leg and breast–wing parts of the carcasses were weighed, vacuum packaged, frozen and then kept at −20°C until analysis.
Measurements of biomechanical properties
After an overnight thawing at 4°C, the gastrocnemius (Gst) and pectoralis minor (Pm) tendons were precisely dissected and immediately immersed in physiological serum to avoid severe dehydration. The Gst tendon was identified as the one that links the m. gastrocnemius to the tarso-metatarsus while the Pm tendon relies the m. pectoralis minor to the humerus bone (Nickel et al., Reference Nickel, Schummer and Seiferle1977). For each animal, one tendon was first used for performing biomechanical tests (tensile tests see above) and then frozen until biochemical analysis. Same measurements were also performed after cooking of the second tendon (previously vacuum packaged) in a water-bath at 80°C for 10 min.
Passive stretch (or tensile) tests were performed with a universal testing machine (MTS System Corporation, Eden Prairie, MN, USA) driven by Testworks 4.0 software. Briefly, the two extremities of the tendons were first deeply frozen in liquid nitrogen to ensure a good adhesion and then inserted in two jaws. One jaw was fixed on the cross-head of the universal testing machine and could move vertically while the second jaw remained fixed on the basis of the machine. The speed of the cross-head was set to 50 mm/min and the length and force values applied to the tendon were recorded (frequency = 100 Hz) until the rupture of the tendon. From the data collected, the software allowed us to measure the following parameters: maximum load (in N), energy at maximum load (in N·mm), stiffness value during the elastic elongation (in N/mm) and elongation (or ΔL in mm) of the tendon at the maximum load. The initial length (L o in mm) of the tendon was recorded before the beginning of the test and the longitudinal strain was calculated as ΔL/L o.
Immediately after the tensile test, parts of the tendon were removed from the jaws, weighed (fresh weight in g), and then frozen and stored at −20°C until biochemical analysis.
Measurements of biochemical properties
All the measurements were done in duplicate. Frozen samples were ground in a mortar. The dry matter content was determined at 103°C for 24 h according to Journal Officiel des Communautés Européennes (1971).
Determination of the total collagen content
The total collagen content was determined according to Woessner (Reference Woessner1961). Briefly, total hydroxyproline (OH-Pro) content of the tissue was determined after total acidic hydrolysis and the OH-Pro content spectrometrically determined at 557 nm. The mean rate of OH-Pro in collagen was estimated to be 14.6%.
Determination of collagen solubility
The soluble fraction of the collagen was determined according to Snowden and Weidemann (Reference Snowden and Weidemann1978). Samples were first heated in a buffer (0.14 mol/l NaCl, 0.01 mol/l PO42−, pH 7) for 10 min at 85 °C. Then the fraction of collagen solubilised by heating was removed after centrifugation at 4000 × g for 30 min. Pellets were then submitted to enzyme (Pronase E from Sigma-Aldrich, St Louis, MD, USA; 1 mg/ml) digestion for 16 h at 20 °C. The solubilised products were collected in supernatant after centrifugation at 80 000 × g for 30 min. OH-Pro content in the different collected fractions was determined according to Woessner (Reference Woessner1961). Solubility of collagen was expressed as the percentage of soluble collagen extracted from total collagen.
Determination of the sulphated glycosaminoglycans (sGAGs) content
Samples were first denatured by heating for 10 min at 85°C in a buffer (0.1 mol/l Tris-acetate, 10 mmol/l calcium acetate, pH 7.8). After centrifugation (4000 × g for 10 min) supernatants were discarded while pellets were submitted to enzymatic digestion (Pronase E, Sigma, 8.33 mg/l) at 37°C for 72 h and under agitation. Supernatants were finally collected after centrifugation at 2000 × g for 10 min and stored at −20°C until analysis. sGAGs content (i.e. chondroitin sulphates+dermatan sulphates+keratan sulphates+heparin sulphates) was determined according to the recommendations of the manufacturer (Biocolor Ltd, UK) of the Blyscan™ sGAGs assay. Percentage of sGAGs sulphated on an N or an O were also determined according to the manufacturer’ procedures.
Statistical analysis
All the data compared values between the two groups of animals by using the general linear method (GLM) procedure of Statistical Analysis Systems Institute (2004). When appropriate, means were compared according to Duncan’s test.
Results
As expected, animals from the two groups exhibited similar carcass (around 1700 g) and thigh (approx. 325 g) weights.
Biomechanical results
Biomechanical results recorded in Gst and Pm tendons are reported in Tables 1 and 2, respectively. Before cooking, neither maximum load nor stiffness nor energy at the maximum load values were found to be significantly different between LR and S chickens. On the contrary, longitudinal strain of Gst and Pm raw tendons were found to be higher in S than in LR birds. After cooking, only the longitudinal stress values were found to be similar in LR and S chickens while other biomechanical parameters were significantly higher in LR than in S chickens.
Table 1 Biomechanical values in raw or cooked Gst tendons from S and LR chickens (values are means ± s.d. (n = 50))

Abbreviations are: Gst = gastrocnemius; LR = Label Rouge; NS = non-significant; S = standard; s.d. = standard deviation.
†Significance levels: *P < 0.05, **P < 0.01, ***P < 0.001.
Table 2 Biomechanical values in raw or cooked Pm tendons from S and LR chickens (values are means±s.d. (n = 50))

Abbreviations are: Gst = gastrocnemius; LR = Label Rouge; NS = non-significant; Pm = pectoralis minor; S = standard; s.d. = standard deviation.
†See Table 1 for significance levels.
From these first observations, we can conclude that the two studied raw tendons have similar biomechanical behaviours in the two groups of animals. In consequence, this behaviour seems to be independent of the physiological function and particularly independent of the level of activity of the tendon in the live bird because it can be hypothesised that the Gst tendon is much more used than the Pm in actions like standing up or walking to reach feeders and drinkers. Moreover, S broilers have a natural tendency to stay still for a long time (Bizeray et al., Reference Bizeray, Leterrier, Constantin, Picard and Faure2000), while LR chickens are usually described as more active.
For both types of birds, the two tendons were found to be less extensible at the raw state than after cooking. After the thermal denaturation induced by cooking, the absolute mechanical values were also less important than before. This thermal denaturation affected more the S than the LR tendons as illustrated by maximum energy value reductions observed, for each tendon, between the raw and cooked state.
Biochemical results
Data reported in Table 3 showed that Gst tendons from the two types of birds weighed approximately the same, while Pm tendons were significantly heavier in LR than in S chickens. Dry matter content was more important in tendons from LR than from S birds, indicating more important biochemical content in older and more active birds. For all samples, total collagen content represented more than two-thirds of the total dry matter content and was consequently the first biochemical component of the tendon in the chicken. The total collagen content was always higher in samples from LR than from S chickens whatever the tendon we considered. On the contrary, the solubility of collagen was less important in LR than in S samples either for Gst or Pm tendons. According to Redaelli et al. (Reference Redaelli, Vesentini, Soncini, Vena, Mantero and Montevecchi2003), the older age of LR birds could explain the observed differences in total collagen and collagen solubility. Nevertheless, increased physical activity of LR birds could also influence those parameters and then explain the lower differences observed in Pm than in Gst tendons which are much more involved in standing up, laying or walking.
Table 3 Biochemical parameters in Gst and Pm tendons from S and LR chickens (values are means ± s.d. (n = 50))

Abbreviations are: Gst = gastrocnemius; LR = Label Rouge; NS = non-significant; S = standard; s.d. = standard deviation; Pm = pectoralis minor.
†See Table 1 for significance levels.
Data reported in Figure 1 showed that Pm tendons contained two times less sGAG than Gst tendons. Moreover, only Gst tendons contained significantly more sGAG in LR than in S birds. This difference was not present in Pm tendons indicating that this parameter could be influenced by the type of tendon. In Figure 2 are reported the percentage of N-sulphated GAGs which are significantly higher in Gst tendons from LR than from S chickens. This difference in percentage of the types of the sulphatation of the GAGs was not observed in Pm tendons from the two types of birds.

Figure 1 Total sulphated glycosaminoglycans (sGAGs) content (% of dry matter) in the two studied tendons (gastrocnemius (Gst) and pectoralis minor (Pm)) from the two types of chickens (Label Rouge and standard). Values are means ± s.d., n = 50. Values with different letters are significantly different (P< 0.05).
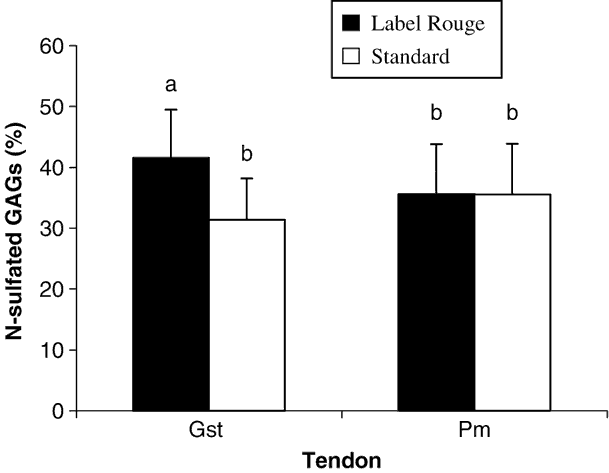
Figure 2 Percentages of N-sulphated glucosaminoglycans (% of total sGAGs content) in two studied tendons (gastrocnemius (Gst) and pectoralis minor (Pm)) from the two types of chickens (Label Rouge and standard). Values are means±s.d., n = 50. Values with different letters are significantly different (P < 0.05).
Discussion
According to Buchanan and Marsh (Reference Buchanan and Marsh2002), one might expect changes in biomechanical properties of tendons to be associated with changes in collagen concentration. This is not the case in our study since a significant difference in total collagen content exists between the two groups of birds but it is not associated with biomechanical modifications. Many other studies also do not support this hypothesis: Viidik (Reference Viidik1967) in rabbits, Vailas et al. (Reference Vailas, Pedrini, Pedrini-Mille and Holloszy1985) in rats or Curwin et al. (Reference Curwin, Vailas and Wood1988) in chickens and Woo et al. (Reference Woo, Gomez, Amiel, Ritter, Gelberman and Akeson1981) in pigs.
As reviewed by Buchanan and Marsh (Reference Buchanan and Marsh2002), it is generally admitted that ageing and training result in increased tensile strength and stiffness values in tendons. With increasing age, tendons are supposed to be more resistant to tensile strength because they have to support a heavier load due to an increase in body weight. In the present experiment, if the two groups of animals were killed at largely different ages (82 v. 40 days of age for LR and S groups, respectively), they exhibited same body and thigh weights. Previous studies also indicate that body compositions are approximately equivalent at these ages between the two types of birds. This indicates that the different tendons from the two groups of animals have to support the same loads. In consequence, it seems normal that most of the biomechanical parameters considered here were found to be in the same ranges for the two types of birds. Nevertheless, for raw tendons, the longitudinal strain value at maximum load was higher in S than in LR samples. This parameter indicates that the degree of stretching was higher in S than in LR tendons. This could have been due to a lower degree of cross-linking of the molecule of collagen generally observed in younger birds and illustrated here by the higher solubility of collagen determined in tendons from S animals.
After cooking, collagen content of the tendons is supposed to be the same as before because heating liberates less than 1% of the total collagen (data not shown). On the contrary, heating is able to modify proteins, such as collagen, by disrupting cross-links within the molecule. This may explain the dramatic decrease of biomechanical values after cooking for both types of birds. In cooked tendons, higher biomechanical values observed in LR birds indicate that they are better able to resist heat denaturation probably because of a higher level of collagen inter-molecular cross-linking normally associated with older age and higher level of physical activity (Sinex, Reference Sinex1964; Davison and Patel, Reference Davison and Patel1975; Mitchell and Rigby, Reference Mitchell and Rigby1975).
If different degrees of cross-linking of the molecule of collagen can explain different longitudinal strain values and different resistance of the tendon to heat denaturation, other biochemical components may also be involved in the determination of mechanical values of the tendon. Cooking implies biochemical modifications, within the tissue, that helps explain the observed dramatic drop of biomechanical properties probably due to a severe dehydration of the tissue. In connective tissue, GAGs produce a genuine hydrophilic gel. This ability to bind water is directly due to the structure of the GAGs in which long filaments are made of repetitive disaccharide monomers particularly prone to form hydrogen bonds able to hold back surrounding water molecules (Neuzil and Valdiguié, Reference Neuzil and Valdiguié2004). In consequence, GAGs are considered as important regulators of tissue visco-elasticity. GAGs polysaccharide chains also tie together collagen fibrils in animal extracellular matrices and their increased number could be associated with increased resistance to stress load (Redaelli et al., Reference Redaelli, Vesentini, Soncini, Vena, Mantero and Montevecchi2003). Nevertheless, in the present study, an increase in the amount of sGAGs in the Gst tendon from the L birds is not associated with an increase in corresponding biomechanical values in raw tendons. On the contrary, during cooking, the presence of higher sGAGs content in Gst tendons from the LR birds could have protected them from a severe dehydration and, consequently, given them a better resistance during the following biomechanical tests. Then, it can be hypothesised that the increased rate in sGAGs content was not sufficient to modify native biomechanical properties of the tendon but give to it a better resistance to cooking dehydration, leading to a better final conservation of native visco-elastic properties and biomechanical properties. This also explains why decreases of biomechanical values are less important in LR than in S animals after cooking. These differences in biochemical organisation of tendons could be due to the age of the animals, but also due to their physical activity because large differences in total sGAGs content are found between Gst and Pm tendons.
From a chemical point of view, GAGs can be sulphated at an O or an N atom. Among the seven existing sGAGs, heparan sulphate is the only one which is sulphated both on O and N atoms while others are only sulphated on O atoms. From the present results that show differences in the type of sulphatation of GAGs in Gst tendons, we must expect that different ways of rearing chickens are able to generate fine regulation of the metabolism of sGAGs. This could be one of the adaptative answers to increased solicitations of tendons. Because GAGs are only a small part of PGs, a precise study of these supramolecules could also give interesting information on possible modifications of the organisation of tendons in birds. Because of the close relationships between PGs (mainly decorin) and the collagen molecule in tendons, other biochemical implications might also be considered.
Conclusions
In this study we demonstrated that biomechanical properties of tendons from LR and S chickens are different mainly after cooking. The low values recorded in S chickens might explain the poor adherence of meat to bone generally reported in modern broilers. From a biochemical point of view, it seems that the most important differences concern the solubility of the collagen as well as the sGAGs and collagen contents. These modifications, mainly the sGAGs content variations, can explain the different water-holding capacity of tendons and therefore different resistance to heat dehydration leading to different mechanical resistances after cooking. Nevertheless, this experimental design failed to distinguish the relative effects of age and physical activity on the determination of biochemical and biomechanical properties of tendons in chickens. Further experiments are being conducted to determine if one of these two parameters has a particular impact on the organisation of the tendons in chickens.
Acknowledgement
The authors would like to thank Dr P. Swider (Purpan Hospital in Toulouse, France) for his help in biomechanical measurements.