1. Introduction
Impact delivery of organic compounds and shock synthesis on planetary bodies, such as the Earth, is considered as one of the possible routes for the supply of prebiotic organics on the early Earth and thus might have played significant in the origin of life (Chyba & Sagan, Reference Chyba and Sagan1992). Impacts release huge amounts of energy, generating shock waves that provide a sudden increase in pressure and temperature due to shock compression and subsequent cooling due to expansion within a very short time scale. Such extreme conditions have the potential to drive complex chemistry. Gilvarry and Hochstim (Reference Gilvarry and Hochstim1963) first proposed the idea of shock synthesis of organic compounds from meteoroids. Hochstim (Reference Hochstim1963) further developed this theory based on physical and chemical parameters associated with shock around a meteorite and suggested that complex chemical compounds could be synthesized due to meteorite impact. Thus shock generated from meteors, airbursts and giant impact plumes could have produced large quantities of organics (Chyba & Hand, Reference Chyba, Hand and Thomas2006). Bar-Nun et al. (Reference Bar-Nun, Bar-Nun, Bauer and Sagan1970) demonstrated the shock synthesis of amino acids in simulated Earth’s primitive atmosphere using the experimental setup of a shock tube. Since then, many experiments have been performed to understand impact-shock chemistry using various experimental designs to simulate various impact environments. As a result of these investigations, many biomolecules, such as amino acids and nucleobases were known to be synthesized by impact-generated shock and related processes (Bar-Nun et al., Reference Bar-Nun, Bar-Nun, Bauer and Sagan1970; Ferus et al., Reference Ferus, Pietrucci, Saitta, Ivanek, Knizek, Kubelík, Krus, Juha, Dudzak and Dostál2019; Ferus et al., Reference Ferus, Rimmer, Cassone, Knížek, Civiš, Šponer, Ivanek, Šponer, Saeidfirozeh and Kubelík2020; Martins et al., Reference Martins, Price, Goldman, Sephton and Burchell2013). Molecular dynamics simulations have also shown that complex chemistry can occur as a result of impact induced shock processes (Goldman et al., Reference Goldman, Reed, Fried, Kuo and Maiti2010; Goldman & Tamblyn, Reference Goldman and Tamblyn2013). However, limited studies are performed to understand their further fate in impact-induced shock condition.
Natural impact processes are very complicated in terms of their physical and chemical characteristics. It is impossible to conduct a comprehensive investigation of the full-scaled effect of the impact processes. So the laboratory investigations are focused only on a limited aspect of the impact process at a time. Most common impact experiments are performed using accelerating devices capable of firing millimetre-sized projectiles with a velocity of a few km s−1. A wide variety of accelerating devices are available in the laboratory, such as single- and two-stage light gas guns, Titov guns, and Van de Graaf accelerator, and so forth (Burchell et al., Reference Burchell, Cole, McDonnell and Zarnecki1999; Gerasimov et al., Reference Gerasimov, Ivanov, Yakovlev and Dikov1998). Such small-scale impact experiments are beneficial for the investigation of physical aspect of impact events, such as the formation of craters, dynamics of ejecta, and others. High-temperature heating effect of impact process can be simulated in the laboratory to investigate the chemical modification induced by impact. Such methods do not reproduce the actual impact process, rather, they simulate characteristic temperatures (thousands of Kelvin) to understand impact-induced melting, vaporization, and chemical reactions at high temperatures.
Among various sources, a powerful pulsed laser is a valuable tool for laboratory simulation of such a process (Berthe et al., Reference Berthe, Bezaeva, Gattaceca, Boustie, de Rességuier and Rochette2011; Křivková et al., Reference Křivková, Petera, Laitl, Kubelík, Chatzitheodoridis, Lenža, Koukal, Knížek, Dudžák and Páclík2021). Although laser-induced irradiation experiments do not reproduce the actual high-pressure shock effect of impact, they are capable of simulationing the high-temperature thermal effect of impacts, when decompression occurs after impact and pressure value returns to normal (Ebert et al., Reference Ebert, Hecht, Hamann and Luther2017). Thus they are a useful tool to investigate chemical processes that are induced as a result of the impact. Many laser experiments have been performed to investigate the melting and mixing process of projectile and target during meteorite impact and shown results as observed in natural impact events (Ebert et al., Reference Ebert, Hecht, Hamann and Luther2017; Gerasimov et al., Reference Gerasimov, Yakovlev, Dikov and Wlotzka2005). The shock tube also offers exciting characteristics, such as a sharp increase in temperature (thousands of kelvin) within a very short time scale and subsequent cooling, with the rate of millions of degrees per second. Such transient events are expected as a result of impact events. Previously, shock tubes have been used for the shock synthesis of amino acids in the laboratory (Bar-Nun et al., Reference Bar-Nun, Bar-Nun, Bauer and Sagan1970). Our previous investigations have reported complex macroscale structure formation due to the shock processing of amino acids and nucleobases, simulating the high-temperature heating effect of shock using a shock tube (Singh et al., Reference Singh, Vishakantaiah, Meka, Sivaprahasam, Chandrasekaran, Thombre, Thiruvenkatam, Mallya, Rajasekhar and Muruganantham2020; Surendra et al., Reference Surendra, Jayaram, Muruganantham, Vijay, Vijayan, Samarth, Hill, Bhardwaj, Mason and Sivaraman2021). In the present investigation, we extend the previous results to by performing the shock studies on other combinations of amino acids at a temperature of ~5,500 K for an approximate time scale of 2 ms.
2. Materials and methods
Shock experiments were performed at the Material Shock Tube (MST1) facility at IISc Bangalore and the High-Intensity Shock Tube for Astrochemistry (HISTA) facility at PRL Ahmedabad for laboratory simulation of impact-induced shock. The detailed experimental method for shock processing of amino acids, measurement of shock speed, reflected shock temperature, and pressure can be found in Surendra et al. (Reference Surendra, Jayaram, Muruganantham, Vijay, Vijayan, Samarth, Hill, Bhardwaj, Mason and Sivaraman2021). The mixture of amino acids (amino acids were procured from Sigma Aldrich, purity >99%) studied in these experiments are shown in Table 1 as are the shock parameters for each of the experiment.
Table 1. Experimental shock parameters for different experiments
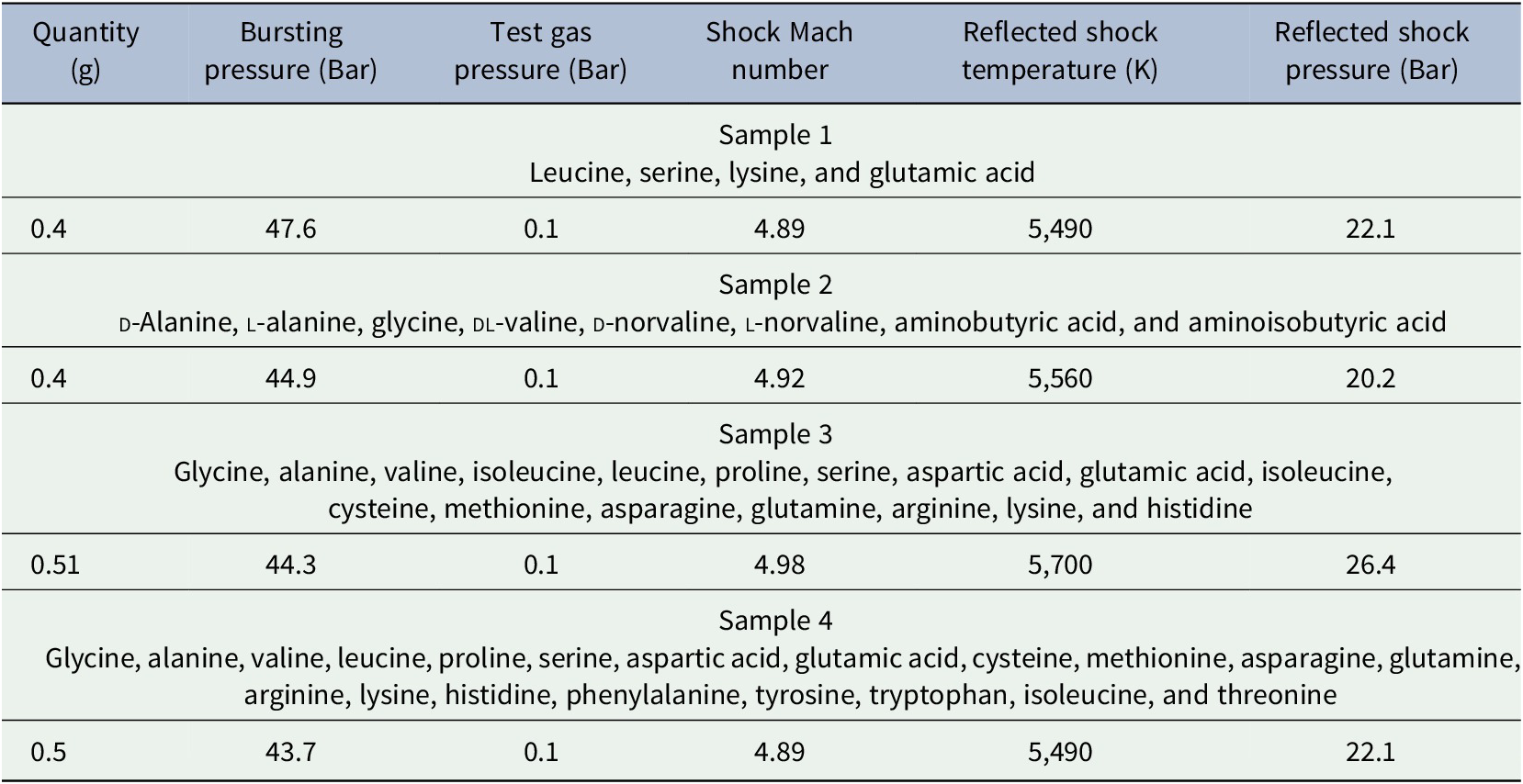
3. Results and discussion
Sample 1, a mixture of four amino acids, containing leucine, serine, lysine, and glutamic acid, was chosen based on a combination of positively charged, negatively charged, and neutral amino acids, as suggested by Miller and Orgel, such combinations of partially complementary components might have stereochemical basis and potentially worth for investigating experimentally (Miller & Orgel, Reference Miller and Orgel1974). The amino acids were mixed in equal weight ratios and shock processed at an approximate temperature of 5,490 K, resulting in large aggregates with a size of hundreds of microns. Imaging the postshock sample, using SEM, showed a solid sphere joint to a cone-like structure with large clumps embedded on the surface (Figure 1a). The magnified images show an irregular texture on the surface with a few holes of diameters of ~10 μm (Figure 1b). The clump architectures seem to be bunched together (Figure 1c), with some of them having blunt corners and others having sharp edges (Figure 1d). These smaller clumped structures were observed to be arranged together in various shapes and many having sharp flakes (Figure 1e–h).
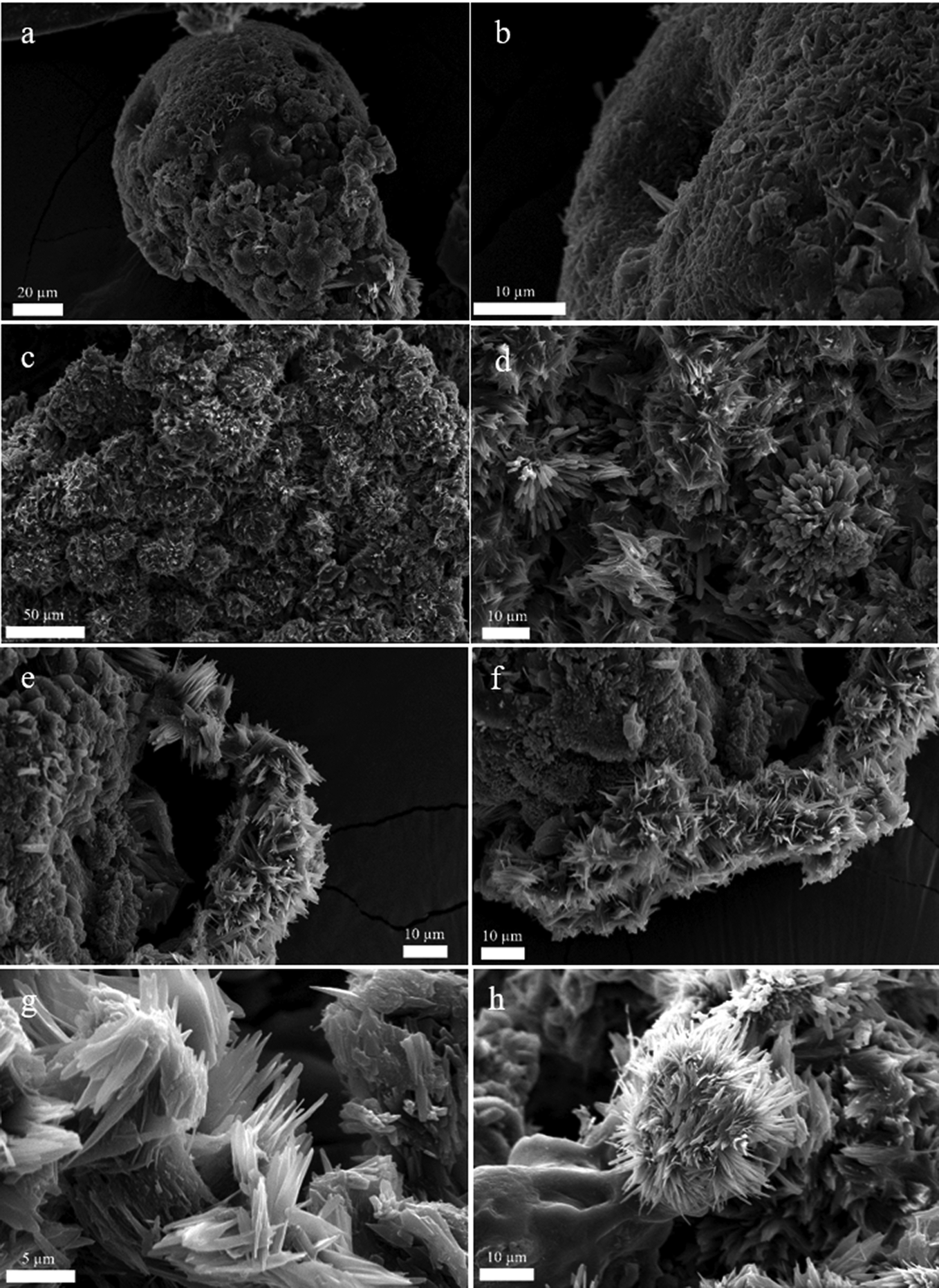
Figure 1. SEM micrograph of shocked sample 1 (containing four amino acids) showing various structures with complex morphology.
In Sample 2, a mixture of eight amino acids was used, mixed in equal weight ratios and shock processed at an approximate temperature of 5,560 K. These amino acids have all been shown to be synthesized together in impact processing of a simple ice mixture (Martins et al., Reference Martins, Price, Goldman, Sephton and Burchell2013). Given the possibility that all these amino acids can be synthesized together from simple molecules in impact process, we intended to investigate their fate under further impact-shock conditions. SEM observations of the shocked material showed entirely different structures to those seen in sample 1. Long thick threads spanning hundreds of microns with fine textures on the threads were observed as shown in Figure 2a. These threads showed a twisted (Figure 2b) and folded (Figure 2c) appearance.
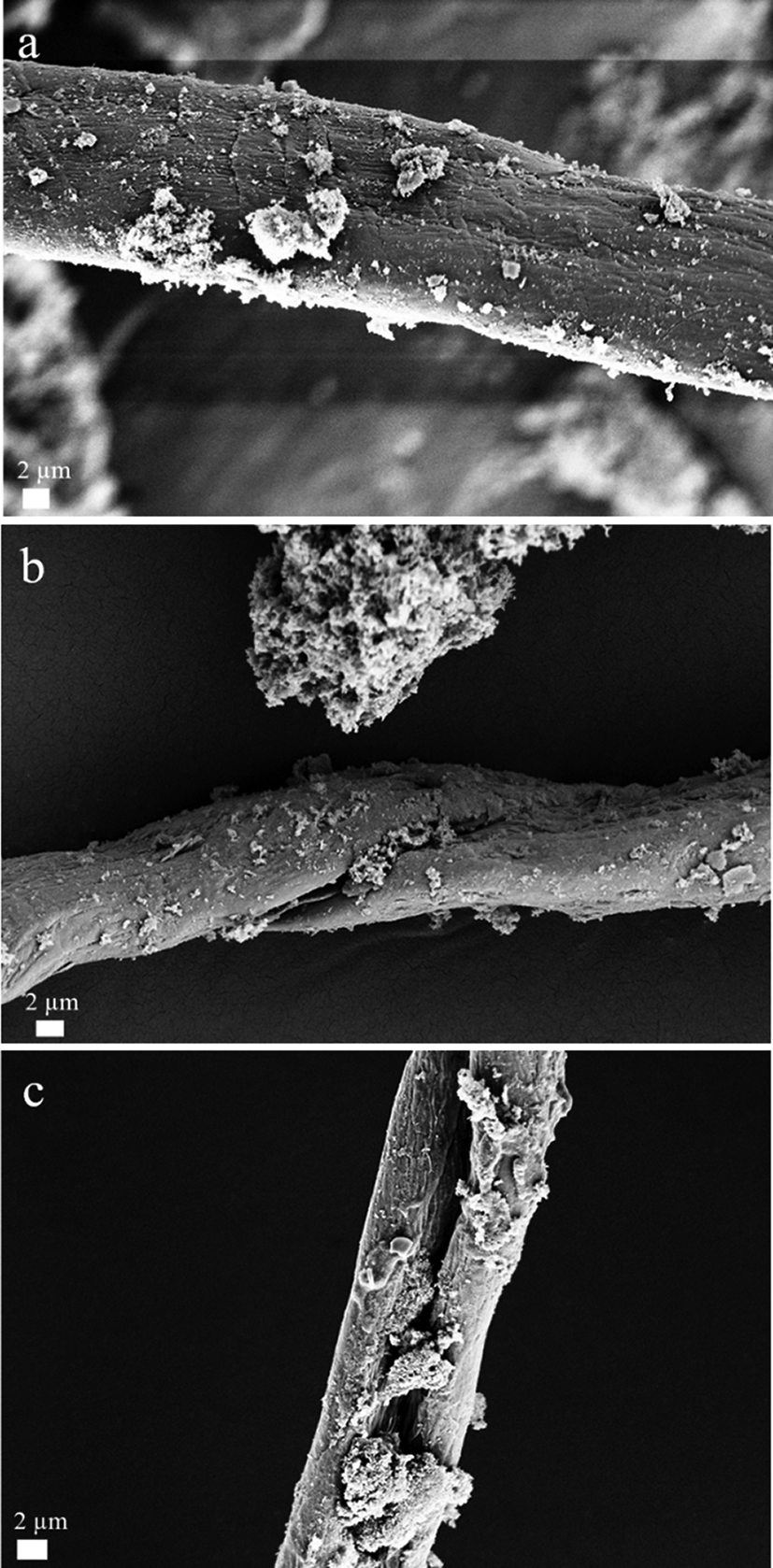
Figure 2. SEM micrograph of shocked sample 2 (containing eight amino acids) showing (a) long-thread morphology with fine surface texture, (b) twisted-thread morphology, and (c) folded threads.
Sample 3 is a mixture of seventeen amino acids mixed in equal weight ratios. Among the twenty proteinogenic amino acids, only seventeen amino acids were chosen, omitting the three amino acids containing a benzene ring. Thses amino acids with benzene ring (e.g. phenylalanine and tryptophan) were omitted because when they were subjected to shock, they do not show any such structure formation, only fine agglomerated particles were seen in these samples as shown in Supplementary Figure S2. When shock processed at a temperature of ~5,700 K, this sample with a mixture of seventeen amino acids produced needle-type nanorod structures (Figure 3a), many of them locked together or branched from a common point (Figure 3b). Similar structures are shown in Figure 3c. In addition, many zigzag structures of varying sizes seem to be hanging with nanorods (Figure 3c).
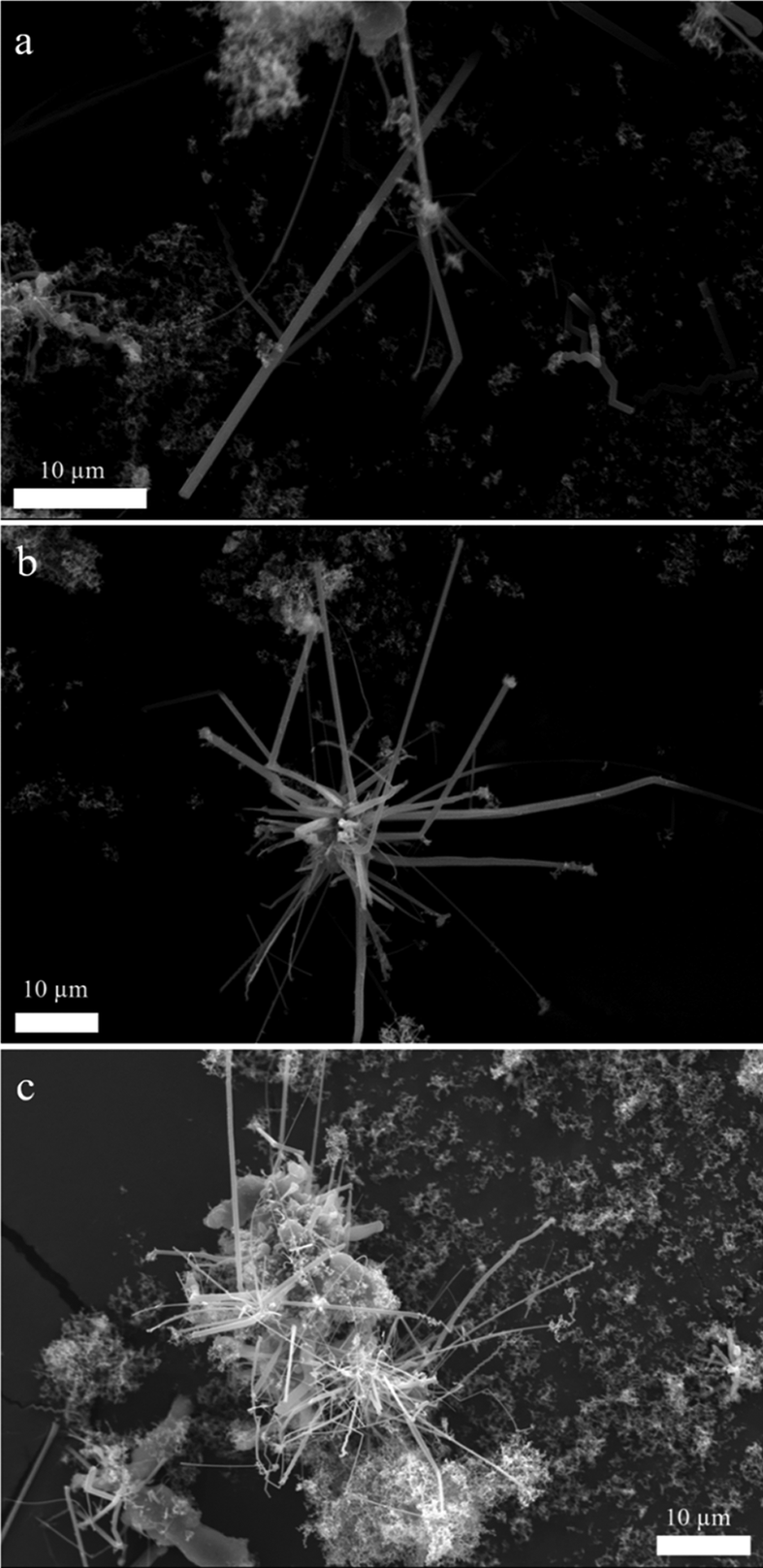
Figure 3. SEM micrograph of shocked sample 3 (containing 17 amino acids) showing (a) rod-like morphological structure, (b) branching structure, and (c) zigzag structures at various length scales.
Sample 4 was a mixture of all twenty amino acids being mixed in equal weight ratios and shock processed at a temperature of ~5,490 K. The shocked sample showed the formation of flat ribbon structures (Figure 4a), hollow tubular structures (Figure 4b), and folded sheet structures (Figure 4c).
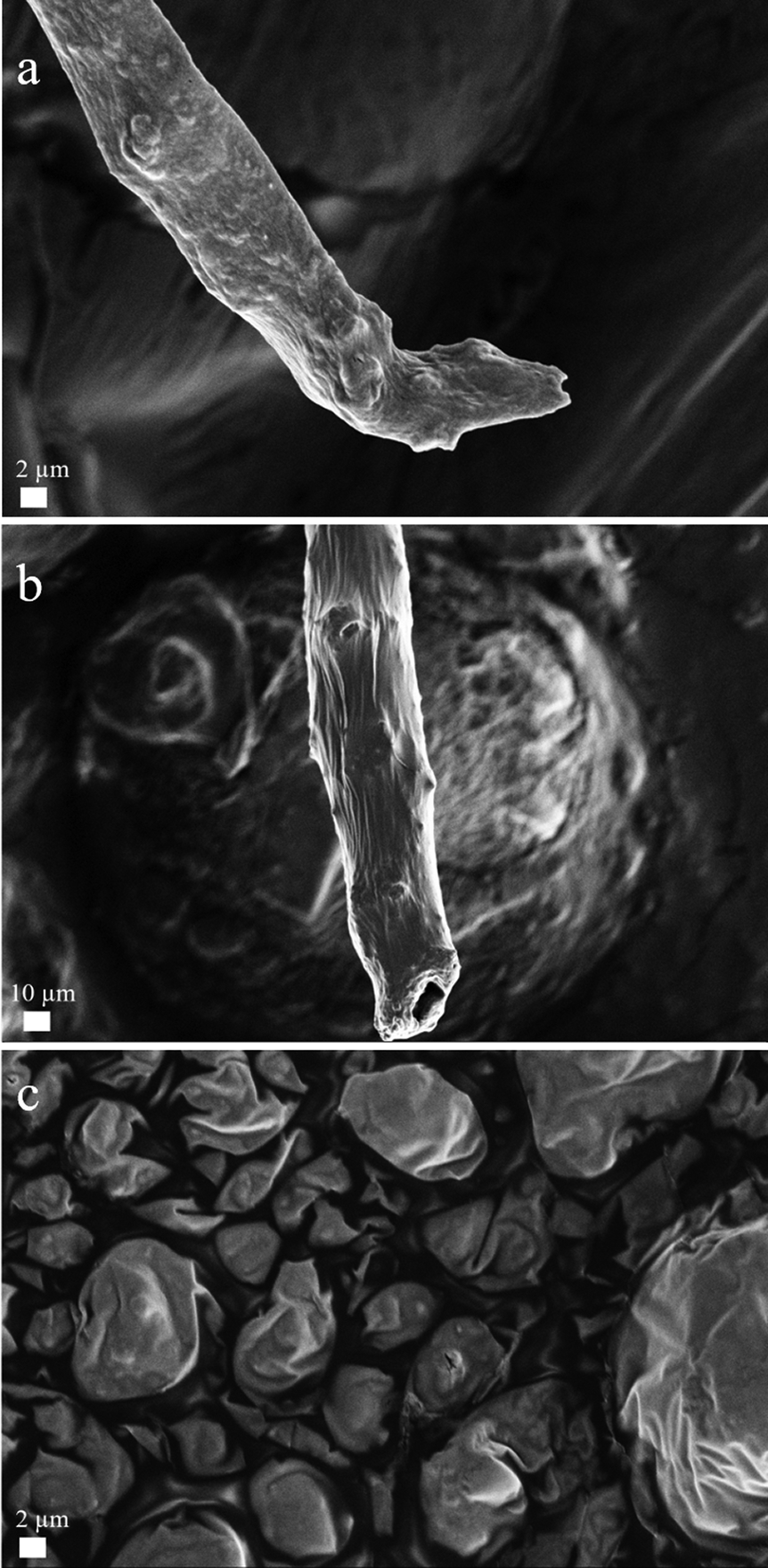
Figure 4. SEM micrograph of shocked sample 4 (containing 20 amino acids) showing (a) micro-ribbon structure, (b) tubular structure, and (c) folded sheet.
Experiments were repeated for all four samples and similar results were obtained in each case, demonstrating that a wide variety of complex structures are formed due to the impact of a strong shock on the mixture of amino acids. The unshocked mixtures of amino acids were also analyzed using SEM and did not show any such complex structures as shown in Supplementary Figure S1. Further, infrared spectroscopy of shock processed residue shows that amino acid signatures are retained in postshock residues, as shown in Supplementary Figures S3–S6.
The formation of such complex structures under simulated conditions of impact bombardment has the potential to offer new pathways for the emergence of life on prebiotic Earth as well as other planetary bodies. The fundamental principle of organization in a cellular system requires organized self-assembly of building blocks of life such as amino acids, peptides, nucleobases, nucleic acids, and lipids in the prebiotic scenario. The demonstration of the formation of such architectures in simulated prebiotic conditions in the laboratory provides an essential step towards understanding prebiotic cellularity. However, it remains a great challenge to design and engineer such well-defined architectures analogous to a biological system, and advanced understanding in this field requires bridging the gap between molecular chemistry and life (Cardoso et al., Reference Cardoso, Cartwright, Čejková, Cronin, De Wit, Giannerini, Horváth, Rodrigues, Russell and Sainz-Díaz2020). Previous attempts have been made to synthesized such well-defined nanostructures such as nanotubes, nanorods, vesicles, and nanofibers by self-assembly process from molecular building blocks relevant to prebiotic chemistry (Folsome, Reference Folsome1976; Fox, Reference Fox1973; Lakshmanan & Hauser, Reference Lakshmanan and Hauser2011; Simionescu et al., Reference Simionescu, Denes and Totolin1985; Valladas-Dubois & Prudhomme, Reference Valladas-Dubois and Prudhomme1983; Yanagawa et al., Reference Yanagawa, Ogawa, Kojima and Ito1988; Zhang, Reference Zhang2012). However, the complex organized architectures achieved by shock processing of amino acids reported in this work have not been revealed before.
4. Conclusions
In this study, laboratory simulations of the high-temperature heating effect induced by shock generated in impact events have been performed. We have shown the spontaneous formation of complex structures organized at different scales in various architecture resulting from shock processing of various mixture of amino acids‥ These results provide elucidation of our understanding of the role played by complex molecules and impact events in the origins of life.
Acknowledgments
S.V.S., J.K.M., M.M., V.S., A.B., and B.S. thank the support from Department of Space (Government of India). S.V.S. and B.S. acknowledge the support from Sir John and Lady Mason Academic Trust (UK). The authors are grateful to Mr. Aravind and Mr. Sarvesh for their help in carrying out the shock experiments; and IIT Gandhinagar, Ahmedabad University and CeNSE IISc for providing SEM facility.
Supplementary Materials
To view supplementary material for this article, please visit http://doi.org/10.1017/exp.2021.17.
Data availability Statement
Samples of the compounds that support these findings are available from the corresponding authors upon request.
Author Contributions
Conceptualization, S.V.S., J.K.M., J.V., and B.S; Methodology, S.V.S., J.K.M., J.V., M.M., V.S., and B.S.; Validation, S.V.S., V.T., V.S., A.B., N.J.M., and B.S.; Formal analysis, S.V.S., J.K.M., J.V., M.M., V.T., V.S., B.N.R., A.B., N.J.M., and B.S.; Investigation, S.V.S., J.V., V.S., B.N.R., A.B., N.J.M., and B.S.; Resources, S.V.S., J.V., V.T., A.B., N.J.M., and B.S.; Data curation, S.V.S., J.K.M, J.V., V.T., and B.S.; Writing—original draft preparation, S.V.S. and J.K.M.; Writing—review and editing, S.V.S., J.V., M.M., V.T., V.S., B.N.R., A.B., N.J.M., and B.S.; Visualization, S.V.S, N.J.M., and B.S.; Supervision, B.S.; Project administration, B.S.; Funding acquisition, B.S. All authors have read and agreed to the published version of the manuscript.
Funding Statement
This research received no external funding.
Conflicts of Interest
The authors declare no conflict of interest.
Comments
Comments to the Author: In this paper, the authors explored reprocessing and survival of aminoacids under extreme conditions of an impact event simulated by quite unique technique of shock tube with a reflected shock temperature of about 5500 K for 2 ms time scale. This paper is extremely interesting for scientific community and it is worth to be published. Except of great scientific value, I regret several language and formal issues in this paper. The body of the paper and the text should be definitely fixed with a native speaker and specialist in the field of prebiotic chemistry. I am not a native speaker, but the terminology used in this paper sounds to me strange: For example, statement that exogenous delivery by an impact event has been considered as one of the possible “methods” (I can imagine a method as a sophisticated, systematic and artificial process made by man, but not by nature). Also, next sentence: “amino acids can be manufactured.” Again, how can be aminoacid manufactured by nature? I can imagine manufacturing of a car in a factory... This is repeated also in the introduction. On the other hand, the paper is well structured and the scientific motivation is clearly presented. I agree that that further reprocessing of prebiotic molecules on the planetary surface must be definitely explored. The major issues are summarized below. The minor language and terminology issues can be fixed by the native speaker and they are not listed specifically.
Major comments:
Abstract: “impacts have been considered as one of the possible methods” replace “methods” by options, possibilities or anything appropriate. I cannot imagine that impact is a method...
“amino acids can be manufactured” replace by “originate” or “can be synthesized”
Introduction:
I recommend to cite classical work of Sagan and Chyba (https://www.nature.com/articles/355125a0), just for justification of the focus on impacts, reprocessing and delivery.
I recommend to add a few lines showing the history of impact shock simulations by shock tubes.
I recommend to compare expected conditions during the meteoroid or asteroid atmospheric entry and impact with the experimental parameters achieved in your set-up. I know that shock tubes are regularly used for this kind of simulations (such as lasers or high velocity guns), but I recommend to compare the conditions and mention also other methods. I recommend to use and cite following references and selected references therein:
a) https://www.sciencedirect.com/science/article/abs/pii/S027311771830406X
b) https://link.springer.com/article/10.1007/s10686-020-09688-3
c) https://lia.scitation.org/doi/10.2351/1.3556591 (provides a good comparison between laser and the real impact)
d) https://www.semanticscholar.org/paper/Meteor-Impact-on-Solid-Surface-Opik/4f41e32666afb893a1657e122be7664a6f0d04ed
Results and discussion:
“a size of 100s of microns, “use” hundreds” instead.
Conclusion:
What I miss here is a kind of chemical analysis. How was the mixture of aminoacids changed chemically? Can you confirm that the observed structures are not just aggregates and they are made of polymers? Consider it or justify, why you did not perform this analysis and if we can be sure that the observed structures are or are not made of aminoacid polymers.