Obesity is a major public health concern across the world(Reference Bagheri, Rashidlamir and Ashtary-Larky1). Based on the WHO reports, 39 % of adults aged 18 years and older (38 % of men and 40 % of women) were overweight(2). The worldwide prevalence of obesity nearly doubled between 1980 and 2014, with 11 % of men and 15 % of women – equivalent to more than half a billion adults – classified as obese(2).
The definition of obesity is dependent upon the assessment method used (i.e. BMI, waist circumference (WC) or body fat percentage (BFP)). BMI is the most common metric used for population and clinical screening for obesity(Reference Cabler, Agarwal and Flint3,Reference Adab, Pallan and Whincup4) . However, it has been clearly demonstrated that obesity is characterised by an excess accumulation of body fat rather than excess body weight alone(Reference Deurenberg, Deurenberg-Yap and Guricci5,Reference Deurenberg-Yap, Schmidt and van Staveren6) . WHO defines obesity as an excessive accumulation of fat to the extent that health may be impaired(7). Therefore, in addition to weight loss (WL) in general, preferential reduction of fat mass (FM) may be the best way to manage obesity and its complications(Reference Ashtary-Larky, Daneghian and Alipour8). As such, the relationships between FM and fat-free mass (FFM) during WL are worthy of consideration. Mathematical modelling by Hall expanded upon early attempts to quantify the proportion of body weight changes attributable to FFM and indicated a small effect of the magnitude of body weight change when initial FM is taken into account and changes in body weight are not large, such as is often observed in lifestyle interventions(Reference Hall9). However, the rate of body weight change was not considered in these models, and the importance of further longitudinal investigations was emphasised.
Dietary interventions for WL have always been considered the first step in obesity management(Reference Jakicic, Clark and Coleman10). Accordingly, a variety of dietary interventions for WL have been suggested(Reference Freire11). Numerous strategies are based on the distribution of macronutrients such as low-carbohydrate/high-fat, high-carbohydrate/low-fat(Reference Yancy, Olsen and Guyton12) or low-carbohydrate/high-protein diet(Reference Floegel and Pischon13), as well as manipulation of energy balance to promote either gradual or rapid WL(Reference Ashtary-Larky, Daneghian and Alipour8). Although rapid WL strategies remain appealing to individuals with obesity, it has been hypothesised that gradual WL may produce superior changes in body composition and anthropometric indices(Reference Ashtary-Larky, Daneghian and Alipour8). In the scientific literature, some researchers and professional organisations(Reference Hill14–Reference Sbrocco, Nedegaard and Stone16) have recommended a gradual WL approach, contending that gradual WL may produce better long-term weight management as compared with rapid WL, which is unlikely to be sustained. However, other researchers(Reference Astrup and Rössner17–Reference Nackers, Ross and Perri20) have posited that larger energy deficits and subsequent rapid WL are more likely to reinforce the weight-change process and produce superior long-term WL outcomes. As a result, uncertainty remains regarding the ideal energy intake and rate of WL needed for optimal obesity management. Additionally, other physiological variables could reasonably be influenced by the rate of WL and its potential effects on body composition. RMR, as the largest component of total energy expenditure, is a proxy indicator of FFM(Reference Carpenter, Poehlman and O’Connell21). Some studies showed that obese subjects decreased their RMR following WL, which is influenced by a decrease in FFM(Reference Ballor and Poehlman22,Reference Kiortsis, Durack and Turpin23) . Moreover, the existence of a low RMR is likely to contribute to reaching to a WL plateau and/ or the high rate of weight regain(Reference Astrup, Gøtzsche and van de Werken24,Reference Trexler, Smith-Ryan and Norton25) . Changes in RMR with WL could potentially be influenced by the rate of WL per se or through effects on FFM.
It is hypothesised that slower WL leads to greater FM loss, and therefore gradual WL diets may better preserve FFM and RMR(Reference Ashtary-Larky, Ghanavati and Lamuchi-Deli26). Yet, the general impact of the WL rate on body composition changes is unsettled, demonstrating the need for comprehensive systematic reviews and meta-examinations of clinical trials on this topic. Therefore, the aim of this study was to conduct a systematic review and meta-analysis of the pooled data from controlled adult human trials to compare the efficacy of dietary WL with different rates in individuals with overweight and obesity for body composition variables including FM, FFM, BFP, WC, hip circumference (HC) and waist:hip ratio (WHR), as well as RMR.
Experimental methods
The present systematic review and meta-analysis adhered to the Preferred Reporting Items of Systematic Reviews and Meta-Analysis statement guidelines(Reference Moher, Liberati and Tetzlaff27).
Data sources and search strategies
A comprehensive literature search of six databases, including PubMed, the Cochrane Library, Web of Science, Embase, Scopus and Ovid, was performed using the keywords ‘weight loss,’ ‘rapid’, ‘quick,’ ‘slow,’ ‘gradual,’ ‘rate of weight loss,’ ‘weight reduction’, ‘diet’ and ‘caloric restriction’ in combination with the keywords ‘body composition’, ‘fat mass’, ‘fat free mass’ and ‘resting metabolic rate’ to identify studies in English languages published up to 10 October 2019. The process of study selection is shown in the flow diagram (Fig. 1).
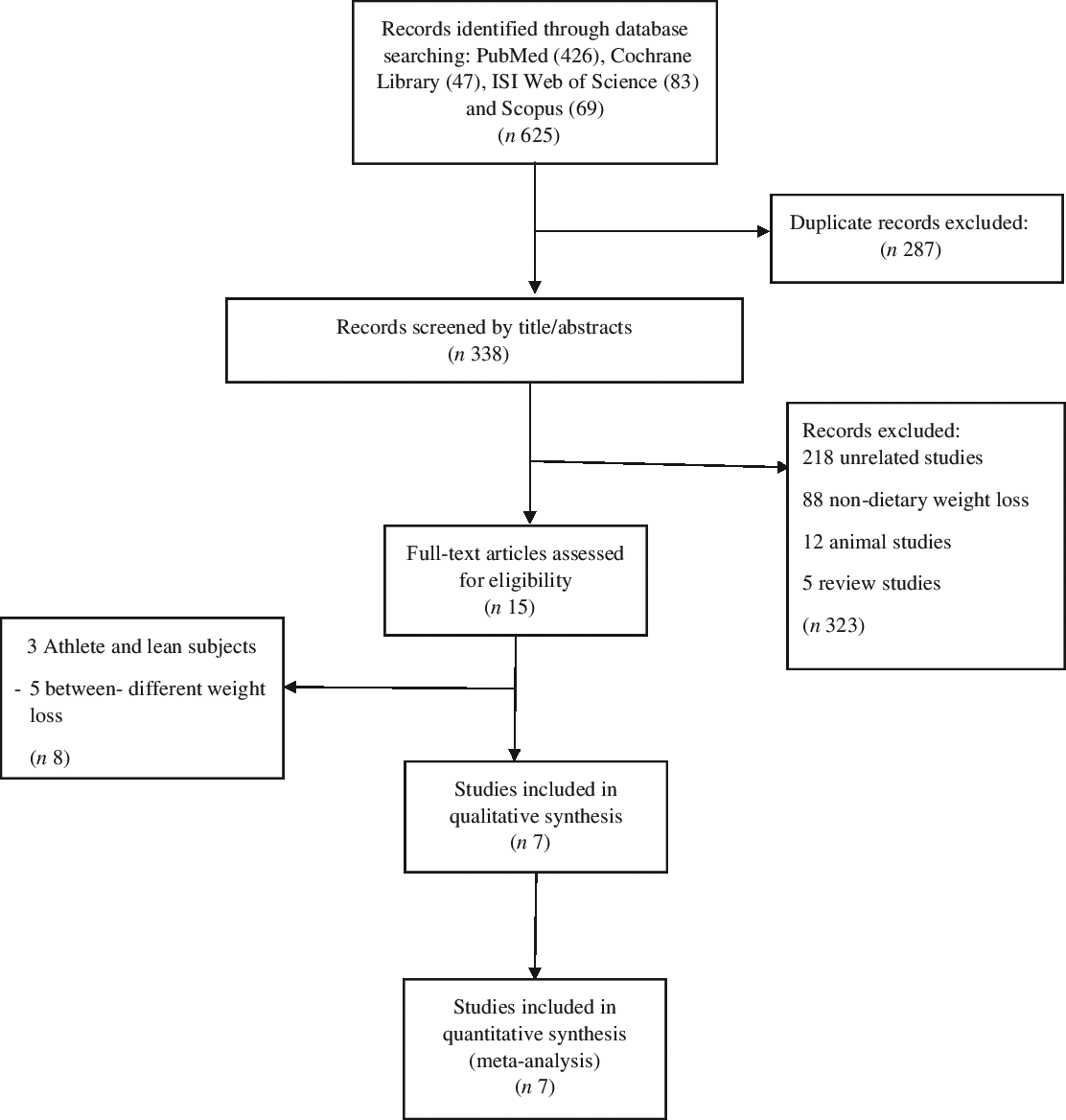
Fig. 1. Flow diagram of the literature search.
Study selection and quality assessment
Two authors independently assessed trial eligibility and quality. To be eligible for inclusion, dietary trials had to evaluate gradual v. rapid WL in patients with overweight/obesity (BMI > 25 kg/m2) and report at least one additional body composition parameter or RMR. Trials where both rapid and gradual WL groups lost similar weight (≤2 kg between-group differences(Reference Brochu, Mathieu and Karelis28,Reference Brochu, Tchernof and Turner29) and/or no significant between-group differences) were included. The Cochrane Collaboration’s tool for assessing the risk of bias was used to assess the risk of bias as previously described(Reference Higgins, Altman and Gøtzsche30). Briefly, nine items were scored, and these items were divided into six domains of bias with three rating categories available for each item: (1) low risk of bias; (2) unclear risk of bias and (3) high risk of bias. All selected articles were scored by two authors (D. A.-L. and R. B.). The disagreement between the assessors was resolved by discussion, and in case of remaining discussion, a third assessor (A. A.) was consulted in order to make a final decision (Table 1).
Table 1. Quality assessment (method: Cochrane Collaboration’s Tool for assessing risk of bias)

Inclusion and exclusion criteria
Articles were included if they satisfied the following criteria: (1) original research article; (2) designed as a parallel or crossover trial; (3) conducted on humans 18 years of age or older; (4) used dietary-induced WL for interventions; (5) conducted on participants with overweight and/or obesity; (6) assessed body composition parameters, anthropometric indices or RMR; (7) intervention groups did not receive any food or supplement and (8) similar total WL in both gradual and rapid WL group (<2 kg between-group differences). Articles were excluded for: (1) unclear data; (2) lack of clear inclusion and exclusion criteria; (3) different amounts of WL and/or ≥2 kg differences between gradual and rapid WL groups; (4) inclusion of normal-weight and/or athletic participants and (5) short study durations (<2 weeks). For articles that contained unclear data, email communications were sent to the corresponding authors to obtain additional information.
Outcomes and data extraction
Data from eligible studies were extracted by two investigators (D. A.-L. and M. A.) using an Excel form. The following data were extracted from each eligible study: first author, publication year, study location, study population, study design, sample size, type of body composition analysis, method of RMR measurement, age, sex ratio, measures of association and brief results together with the adjusted covariates.
Data synthesis and analysis
Meta-analysis was carried out using Stata version 12.0 (Stata Corp.). The fixed effects model was used for the assessment of the pooled effect size. When heterogeneity was present, the random effects model was used. Heterogeneity was tested using the I 2 statistic, and an I 2 value ≥50 % with a level of significance of P < 0·05 by the Cochran Q-test was interpreted as evidence of substantial heterogeneity. Publication bias was assessed by a funnel plot analysis, the Begg adjusted rank correlation test and the Egger regression asymmetry test. Additionally, the meaning test was used to assess the effect of individual studies by estimating the r values obtained when each study was omitted.
Results
Study selection
The first step of searching yielded 462, 47, 83 and 69 citations in PubMed, Cochrane Library, Web of Science and Scopus, respectively. Of these, 287 articles were excluded due to the duplication. The titles and abstracts of 338 articles were reviewed. Of these, 323 studies were excluded due to the following reasons: animal model, reviews, non-dietary WL and unrelated studies. Therefore, the full text of fifteen studies assessed for eligibility. Eventually, seven articles were included in this meta-analysis(Reference Ashtary-Larky, Daneghian and Alipour8,Reference Ashtary-Larky, Ghanavati and Lamuchi-Deli26,Reference Hintze, Goldfield and Seguin31–Reference Sénéchal, Arguin and Bouchard35) (Fig. 1). It should be noted that not all outcomes were reported in the seven studies included.
Power analysis
A fixed-effect meta-analysis necessarily results in an increase in power(Reference Jackson and Turner36). Therefore, we have performed a power analysis for random-effect meta-analysis(Reference Valentine, Pigott and Rothstein37). According to the results, we used a random effects model only for FFM. The power calculated for FFM was 0·95.
Characteristics of included studies
All studies except for one were randomised controlled studies. The intervention duration ranged from 9 to 36 weeks and 5 to 12 weeks in gradual and rapid groups, respectively. Pooled data included 167 participants from gradual WL intervention arms and 194 participants from rapid WL arms. The age range of the participants was 18–70 years. All the included studies were parallel in design. Five studies were conducted on both sexes, and two other studies were performed on only females. Of seven studies included in the systematic review and meta-analysis, all seven studies reported weight, FM and FFM(Reference Ashtary-Larky, Daneghian and Alipour8,Reference Ashtary-Larky, Ghanavati and Lamuchi-Deli26,Reference Hintze, Goldfield and Seguin31–Reference Sénéchal, Arguin and Bouchard35) , five studies reported WC(Reference Ashtary-Larky, Daneghian and Alipour8,Reference Ashtary-Larky, Ghanavati and Lamuchi-Deli26,Reference Purcell, Sumithran and Prendergast32,Reference Vink, Roumans and Arkenbosch33,Reference Sénéchal, Arguin and Bouchard35) , four studies reported BMI and HC(Reference Ashtary-Larky, Daneghian and Alipour8,Reference Ashtary-Larky, Ghanavati and Lamuchi-Deli26,Reference Purcell, Sumithran and Prendergast32,Reference Vink, Roumans and Arkenbosch33) , RMR(Reference Ashtary-Larky, Daneghian and Alipour8,Reference Ashtary-Larky, Ghanavati and Lamuchi-Deli26,Reference Hintze, Goldfield and Seguin31,Reference Coutinho, With and Rehfeld34) , and BFP(Reference Ashtary-Larky, Daneghian and Alipour8,Reference Ashtary-Larky, Ghanavati and Lamuchi-Deli26,Reference Vink, Roumans and Arkenbosch33,Reference Coutinho, With and Rehfeld34) and three studies reported WHR(Reference Ashtary-Larky, Daneghian and Alipour8,Reference Ashtary-Larky, Ghanavati and Lamuchi-Deli26,Reference Vink, Roumans and Arkenbosch33) . The basis on the Cochrane Collaboration’s tool, four studies had a low risk of bias (≥4) and a quality score of three studies were lower than 4 (Table 1). The energy intake also varied between studies. The characteristics of the included studies are summarised in Table 2.
Table 2. Characteristics of included studies
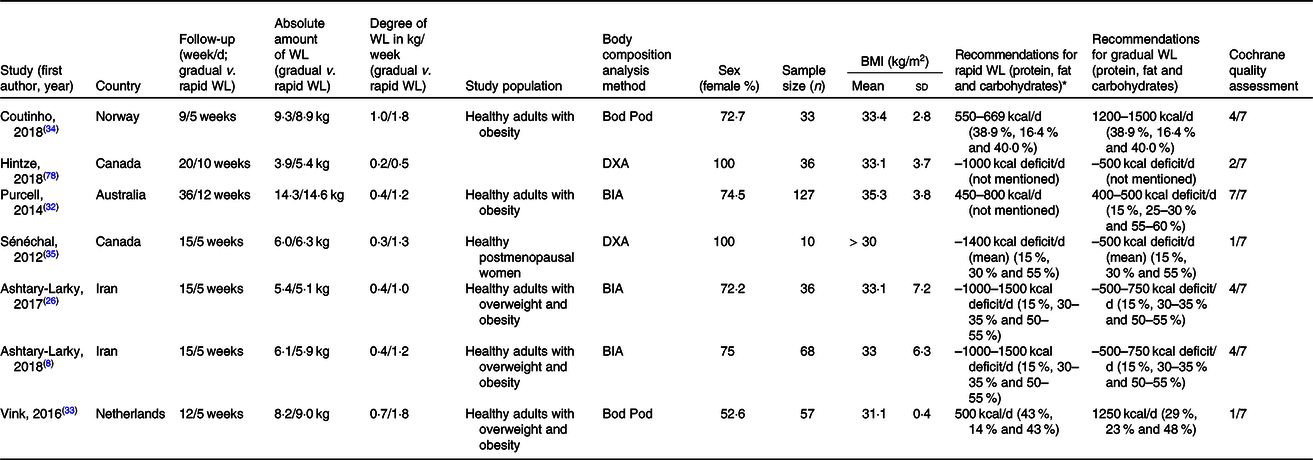
WL, weight loss; DXA, dual-energy X-ray absorptiometry; BIA, bioelectrical impedance analysis.
* To convert energy values from kcal to kJ, multiply by 4·184.
Gradual weight loss v. rapid weight loss
Based on the analysis of seven RCT, gradual WL produced greater reductions in FM (weighted mean difference (WMD) kg: –1·00, 95 % CI –1·70, –0·29) and BFP (WMD –0·83 %, 95 % CI –1·49, –0·17). Moreover, gradual WL significantly attenuated the reduction of RMR (WMD 407·48 kJ, 95 % CI 78·78, 118·01) compared with rapid WL. However, there was no significant difference in body weight (WMD 0·03 kg, 95 % CI –0·65, 0·71), BMI (WMD 0·14 kg/m2, 95 % CI –0·25, 0·52), HC (WMD 0·21 cm, 95 % CI –1·20, 1·63), WC (WMD –0·32 cm, 95 % CI –1·80, 1·16), FFM (WMD 0·74 kg, 95 % CI –0·15, 1·64) and WHR (WMD –0·00, 95 % CI –0·02, 0·01) between gradual and rapid WL (Figs. 2–10). Mean changes in body composition and RMR in rapid and gradual WL, respectively, are as follow: weight: –7·7 ± 3·5 and –7·5 ± 3·5; BMI: –3 ± 1·6 and –3 ± 1·5; FM: –5·6 ± 3·6 and –6·7 ± 3·7; PBF: –2·5 ± 1·8 and –3·8 ± 1·2; WC: –7·8 ± 4·5 and –8·7 ± 4·2; HC: –6·3 ± 4·7 and –7·4 ± 5; WHR: –0·04 ± 0·05 and –0·05 ± 0·5; FFM: –1·6 ± 1·3 and –0·6 ± 0·6; RMR: –136·9 ± 58·6 and –87·5 ± 74·3.
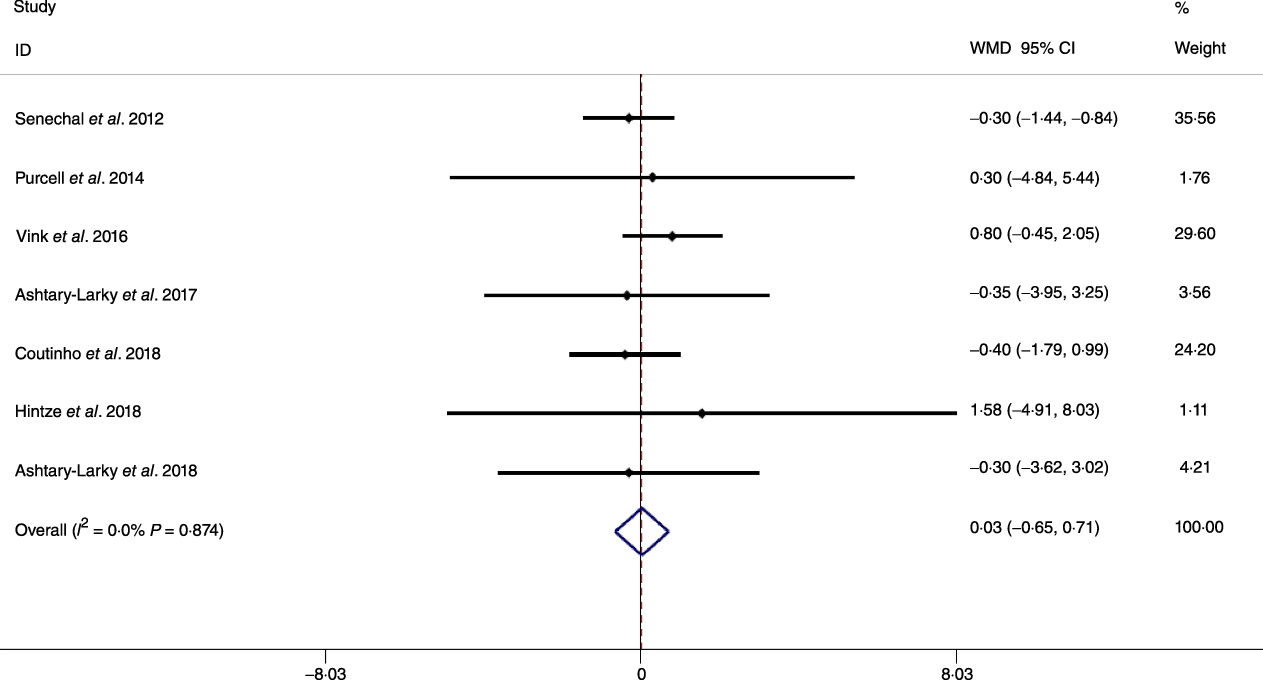
Fig. 2. Forest plot of the fixed effects meta-analysis of the effect of gradual v. rapid weight loss on body weight. WMD, weighted mean difference.

Fig. 3. Forest plot of the fixed effects meta-analysis of the effect of gradual v. rapid weight loss on fat mass. WMD, weighted mean difference.

Fig. 4. Forest plot of the fixed effects meta-analysis of the effect of gradual v. rapid weight loss on body fat percentage. WMD, weighted mean difference.

Fig. 5. Forest plot of the random effects meta-analysis of the effect of gradual v. rapid weight loss on fat-free mass. WMD, weighted mean difference.

Fig. 6. Forest plot of the fixed effects meta-analysis of the effect of gradual v. rapid weight loss on RMR. WMD, weighted mean difference.

Fig. 7. Forest plot of the fixed effects meta-analysis of the effect of slow v. rapid weight loss on BMI. WMD, weighted mean difference.

Fig. 8. Forest plot of the fixed effects meta-analysis of the effect of slow v. rapid weight loss on waist circumference. WMD, weighted mean difference.

Fig. 9. Forest plot of the fixed effects meta-analysis of the effect of slow v. rapid weight loss on hip circumference. WMD, weighted mean difference.

Fig. 10. Forest plot of the fixed effects meta-analysis of the effect of slow v. rapid weight loss on waist:hip ratio. WMD, weighted mean difference.
Publication bias and sensitivity analysis
Publication bias as assessed by Egger’s regression asymmetry test was as follows: RMR (P = 0·005), FFM (P = 0·327), HC (P = 0·004), WC (P = 0·093), BFP (P = 0·012), FM (P = 0·405), BMI (P = 0·093) and body weight (P = 0·920). Results of the sensitivity analysis indicated that the elimination of each individual study did not change the pooled effect size (online Supplementary File 1).
Discussion
In this meta-analysis, we compared the effects of gradual v. rapid WL on body composition in individuals with overweight and obesity. The main result of our analysis was that when a similar magnitude of WL occurred, gradual WL was associated with greater declines in FM and BFP, as well as superior preservation of RMR. However, the rate of WL was not associated with differential changes in FFM, WC, HC and WHR.
Previous studies have shown that obesity is a risk factor for all-cause mortality and fatal cardiovascular events(Reference Lissner, Odell and D’Agostino38,Reference Blair, Shaten and Brownell39) . Moreover, increases in body fat may result in distinct disease risk as compared with increases in BMI alone(Reference Saito, Takahashi and Arioka40). It has been posited that gradual WL may increase the proportion of weight lost as FM. Although our results supported this contention by demonstrating that compared with rapid WL, gradual WL induced larger FM decrements, we did not detect significant differences in metrics of central obesity (i.e. WC and WHR). Several factors could be responsible for the disparity in whole-body FM and anthropometric measures related to central obesity. First, while WC and HC are well-known predictors of central obesity and decrease following dietary WL, they are not the best indices in terms of correlation with FM (as a criterion in obesity evaluation) during dietary WL(Reference Ashtary-Larky, Daneghian and Alipour8). Second, while the magnitude of WL observed in the present analysis (6·94 kg in gradual WL and 6·98 kg in rapid WL) elicited decreases in WC and HC, differences in these anthropometric indicators of central obesity based on the rate of WL may not have been large enough to become distinguishable. Therefore, further studies with long-term interventions, greater magnitudes of WL or more precise anthropometric measurement methods(Reference Tinsley, Moore and Dellinger41) may be needed to allow for additional evaluation of changes in surface anthropometric indices following gradual and rapid WL.
Preservation of muscle mass accompanied by FM loss is the ideal outcome following dietary WL. In practice, WL achieved through a energy-reduced diet decreases both FM and FFM, the latter of which contains the majority of skeletal muscle(Reference Cava, Yeat and Mittendorfer42). In individuals with overweight or obesity, FFM contributes approximately 20–30 % to total WL(Reference Gormsen, Svart and Thomsen43–Reference Tobias, Chen and Manson48). It is well established that increasing dietary protein attenuates the WL-induced reduction in muscle mass(Reference Verreijen, Verlaan and Engberink49–Reference Layman, Evans and Baum51). However, the potential health benefits of WL could be attenuated by the WL-associated reduction of FFM(Reference Layman, Boileau and Erickson52–Reference Miller and Wolfe54), which when present along with other co-factors such as smoking and lack of exercise could increase the risk of additional disease states such as sarcopenia(Reference Miller and Wolfe54–Reference Fielding, Vellas and Evans56). Traditionally, a gradual WL has been suggested to be better preserve FFM. Some studies reported that rapid WL diets are suboptimal for FFM preservation(Reference Ashtary-Larky, Ghanavati and Lamuchi-Deli26,Reference Vink, Roumans and Arkenbosch33,Reference Peos, Norton and Helms57) . However, the present meta-analysis demonstrated that although rapid WL appeared to result in approximately 1 kg greater mean FFM loss than gradual WL, the difference between WL rates was not statistically significant. Several factors could be responsible for these results. First, it is well established that increasing dietary protein may attenuate the WL-induced reduction in muscle mass(Reference Pasiakos, Cao and Margolis58,Reference Church, Gwin and Wolfe59) . Some (two of seven) studies(Reference Hintze, Goldfield and Seguin31,Reference Purcell, Sumithran and Prendergast32) included in the meta-analysis did not report sufficient dietary information for these variables to be considered in the present analysis, meaning it is possible that the percentage of energy derived from different macronutrients, notably protein, could have differed between experimental arms or studies. Second, it has been suggested that the size of the energy deficit determines the extent of FFM loss(Reference Garthe, Raastad and Refsnes60), and the energy deficit varied widely in the included studies, even though all investigations implemented some form of a very low-energy diet. Third, although most of the included studies in our analysis directionally favoured gradual WL for FFM preservation, the lack of a significant difference could be due to a relatively small magnitude of the effect and the limited duration of these studies. Fourth, studies measured body composition using different methods including bioelectrical impedance analysis, dual-energy X-ray absorptiometry and air displacement plethysmography using Bod Pod®. Previous reports have demonstrated that there are significant differences in FFM estimates obtained by these methods(Reference Ritz, Salle and Audran61). Thus, the difference in techniques might explain some variability in the analysed data. Conversely, only one of seven studies included in our analysis showed apparently superior FFM changes after rapid WL as compared with gradual WL(Reference Purcell, Sumithran and Prendergast32). Since this study had a higher number of participants than any other investigation (n 127), the results had a large impact on the overall effect size. In contrast to our analysis, an earlier study that compared gradual and rapid WL, with body weight reductions of 1·9 v. 1·1 kg/week over 8 weeks, demonstrated that the rapid WL group experienced a larger reduction in FFM(Reference Coxon, Kreitzman and Brodie62). However, after adjusting for the magnitude of WL, differences between groups no longer remained. In addition, Vink et al.(Reference Vink, Roumans and Arkenbosch33) showed during similar WL, rapid WL induced greater loss of FFM compared with gradual WL (1·8 v. 0·6 kg/week). These contradictory findings indicate the need for more high-quality and long-term research to determine if gradual WL is better suited for the preservation of FFM during WL in individuals with overweight and/or obesity. Additionally, despite the lack of statistical significance, the practical significance of the approximately 1 kg mean difference in FFM between gradual and rapid WL in the present analysis should be considered.
Decreases in RMR are a well-known consequence of WL(Reference Coxon, Kreitzman and Brodie62–Reference Browning, Franco and Cyrus66). A prior investigation demonstrated reductions in RMR following WL occur as early as the first week, with a continued decline until the end of the 10–20-week intervention(Reference Hintze, Goldfield and Seguin31). Similarly, other studies observed a decline in RMR from the early portion of energetic restriction interventions(Reference Müller, Enderle and Bosy-Westphal65,Reference Bray67) . It has been shown that both rapid and gradual WL may cause decreases in RMR(Reference Ashtary-Larky, Ghanavati and Lamuchi-Deli26). Our results showed that the rapid WL group presented a larger decrease in RMR. Since FFM contributes to metabolic rate, RMR decreases as FFM is decreased(Reference Byrne, Weinsier and Hunter68). However, given that changes in FFM do not fully explain the alteration in RMR after WL(Reference Siervo, Faber and Lara69), it has been suggested that the metabolic, neuroendocrine and autonomic systems regulating energy stores may be involved(Reference Rosenbaum, Hirsch and Murphy70). The adipocyte-secreted hormone leptin is one such factor, along with Peptide YY and thyroid hormones(Reference McNeil, Schwartz and Rabasa-Lhoret71), which may mediate these adaptive changes in energy expenditure(Reference Rosenbaum, Hirsch and Murphy70,Reference Park and Ahima72) . It has been shown that dietary WL may decrease the plasma levels of both, 3,5,3’ triiodo-l-thyronine (T3)(Reference Rosenbaum, Hirsch and Murphy70,Reference Gardner, Kaplan and Stanley73) and leptin. Müller & Bosy-Westphal reported a trend for a decline in serum triiodothyronine concentrations (0·307 ± 0·614 nmol/l) that correlated (r –0·56; P < 0·05) with the decrease in resting energy expenditure adjusted for FFM and FM following 3 weeks of energy restriction(Reference Müller and Bosy-Westphal74). However, there are limited data concerning the differences in hormonal and neurological responses following slow and rapid WL. Nonetheless, modulation of these physiological factors could be a possible reason for the attenuation in RMR reduction with gradual WL compared with rapid WL despite no statistically significant differences in FFM changes in our analysis. However, only four studies measured RMR, while all seven studies measured FFM. Because of limited data on the metabolic effect of the rate of WL, more studies are needed to determine the effects of gradual and rapid WL on RMR.
Our present analysis is not without its limitations. The meta-analysis was based on only seven trials, and some studies did not report dietary contents in each intervention. Thus, it is not possible to evaluate the effects of macronutrient composition or meal frequency on the observed results. Furthermore, since all but one trial lasted less than 4 months, our analysis is unable to show the long-term differences of gradual and rapid WL on anthropometric indices and RMR in individuals with overweight and/or obesity. Another limitation is the devices used for body composition analysis in the included studies. From seven studies, three studies measured body composition by bioelectrical impedance analysis, two studies used dual-energy X-ray absorptiometry and two other studies used Bod Pod. Although all three methods are established valid methods of assessing body composition in certain contexts, they do not always reflect changes in body composition associated with WL similarly(Reference Ritz, Salle and Audran61). Moreover, included studies provided different measures of non-fat tissue, that is, lean body mass and FFM. It has been mentioned that the differences between LBM and FFM are about 2–3 %(Reference Withers, Craig and Ball75). Therefore, the terms LBM and FFM are often used interchangeably(Reference Prado and Heymsfield76). Last, the lack of an energy deficit demarcation to define gradual and rapid WL is another limitation of our analysis. While future analyses could perform meta-regression to examine these effects, this is tenuous due to substantial concerns regarding self-reported dietary intake(Reference Dhurandhar, Schoeller and Brown77) and was beyond the scope of the present analysis.
In conclusion, gradual WL is associated with greater loss of FM and BFP, as well as enhanced maintenance of RMR, in participants with overweight and obesity. However, the rate of WL was not associated with different changes in FFM, WC, HC and WHR. Additional longer-term and high-quality clinical trials are needed to evaluate the differences of gradual and rapid WL, when similar WL is achieved, on body composition and physiological variables in individuals with overweight and/or obesity to further evaluate and confirm these findings.
Acknowledgements
No financial support was provided.
The contributions of the authors were as follows: D. A.-L. and R. B. designed the study; D. A.-L. and A. A. were responsible for review and study selection; D. A.-L. and M. A. extracted the data; D. A.-L. and A. A. were responsible for statistical analysis; D. A.-L., R. B., G. M. T. and A. W. drafted the manuscript.
The authors declare that there are no conflicts of interest.
Supplementary material
For supplementary material referred to in this article, please visit https://doi.org/10.1017/S000711452000224X