Insulin resistance is a characteristic feature of type 2 diabetes that can cause many complications if left untreated. Although no consensus for a unifying mechanism has been reached( Reference Ye 1 ), several lines of evidence suggest that oxidative stress plays an important role( Reference Evans, Goldfine and Maddux 2 ). Therefore, there is an urgent need to find effective strategies against oxidative stress to prevent or treat insulin resistance( Reference Jimenez-Osorio, Gonzalez-Reyes and Pedraza-Chaverri 3 ).
Nuclear factor E2-related factor 2 (Nrf2) is a basic leucine zipper transcription factor that regulates the expression of many antioxidant and phase II detoxifying enzymes, including haeme oxygenase 1 (HO-1), glutamate-cysteine ligase C (GCLC), glutamate-cysteine ligase M (GCLM), NAD(P)H:quinone oxidoreductase 1 (NQO-1) and others( Reference Motohashi and Yamamoto 4 , Reference Kensler, Wakabayashi and Biswal 5 ), thus acting as a central regulator for oxidative stress. Accumulating evidences indicate that Nrf2 is a therapeutic target for a variety of metabolic disorders, particularly insulin resistance. It has been shown that rimonabant, a cannabinoid 1 receptor antagonist, ameliorates hepatic fat infiltration and inflammation by activating the hepatic Nrf2-5’ AMP-activated protein kinase pathway in a rat model( Reference Chang, Kim and Yang 6 ). Also, oltipraz, an Nrf2 activator, prevents high-fat diet-induced insulin resistance and obesity in C57BL/6J mice( Reference Yu, Shao and Chiang 7 ). In addition, increased Nrf2 signalling suppresses hypothalamic oxidative stress and improves insulin and leptin resistance in rats( Reference Yagishita, Uruno and Fukutomi 8 ).
Butyrate is a SCFA that naturally exists in butter and cheese( Reference Hamer, Jonkers and Venema 9 ) and can also be produced endogenously by colonic bacterial anaerobic fermentation of fibre polysaccharides( Reference Canani, Costanzo and Leone 10 ). SCFA not only play an important role maintaining gut health but also enter the systemic circulation and directly affect metabolism and the function of peripheral tissues( Reference Canfora, Jocken and Blaak 11 ). Many studies have indicated that supplementing with butyrate through various means may help prevent obesity and whole-body insulin resistance( Reference Chang, Kim and Yang 6 , Reference Khan and Jena 12 , Reference Huang, Gao and Chen 13 ). Gao et al. reported that dietary supplementation of sodium butyrate (NaB) results in reduced fasting glucose and insulin levels, as well as improved insulin sensitivity in C57BL/6J mice fed a high-fat diet( Reference Gao, Yin and Zhang 14 ). Similarly, butyrate supplementation in drinking water also protects against high-fat diet-induced glucose intolerance and metabolic disorders in type 2 diabetic mice( Reference Zhang, Du and Yano 15 ). Additionally, butyrate ameliorates insulin resistance and improves renal function in a rat model of chronic kidney disease by promoting the intestinal barrier function( Reference Gonzalez, Krieg and Massey 16 ). NaB modulates high-fat diet-induced skeletal muscle mitochondrial adaptation in mice with increased percentage of type 1 fibres showing higher β-oxidation activity and insulin sensitivity( Reference Henagan, Stefanska and Fang 17 ). Oral butyrate prevents diet–induced insulin resistance in mice via reducing energy intake and enhancing fat oxidation by activating brown adipose tissue( Reference Li, Yi and Katiraei 18 ). NaB and its synthetic amide improve glucose tolerance in the rat liver by inhibiting inflammation and reducing steatosis( Reference Mattace Raso, Simeoli and Russo 19 , Reference Jin, Sellmann and Engstler 20 ), as well as affecting the activation of the insulin-like growth factor type 1 receptor signalling pathway( Reference Karna, Miltyk and Palka 21 ). Moreover, NaB is reported to protect against oxidative stress by modulating the Nrf2 pathway and mitochondrial function in HepG2 cells( Reference Xing, Jiang and Tang 22 ). However, it remains elusive whether and how Nrf2 is involved in the action of butyrate in alleviating high-fat diet-induced oxidative stress.
Butyrate exerts its biological function by binding to its G protein coupled receptors( Reference Miyamoto, Hasegawa and Kasubuchi 23 , Reference Brown, Goldsworthy and Barnes 24 ) or acting as a histone deacetylase (HDAC) inhibitor( Reference Davie 25 ). It has been reported that NaB inhibits the diabetes-associated increase in HDAC activity and restores the acetylation of histone H3 in liver, thereby reducing insulin-resistance in type 2 diabetic rats( Reference Khan and Jena 12 ). Nevertheless, it remains elusive whether butyrate regulates hepatic Nrf2 expression by modulating histone acetylation status on its promoter.
Therefore, the objectives of the present study were (1) to demonstrate the efficacy of NaB in ameliorating high-fat diet-induced oxidative stress and insulin resistance in rats; (2) to examine, both in vivo and in vitro, whether the actions of NaB are mediated by up-regulation of Nrf2 expression; and (3) to link the Nrf2 up-regulation with alterations in HDAC activity and histone acetylation status on Nrf2 gene promoter in the liver of NaB-treated rats.
Materials and methods
Animal model and experimental protocol
Male specific-pathogen-free (a term used for laboratory animals that are guaranteed free of particular pathogens) Sprague–Dawley rats (6 weeks of age) were purchased from Beijing Vital River Laboratory Animal Technology Co., Ltd. and were raised at the Animal Core Facility of Nanjing Medical University. They were kept in a controlled room with a temperature of 22 ± 0·5°C, humidity of 50 ± 5 % and a 12 h–12 h light–dark cycle. After 1 week of acclimatisation, the rats were randomly divided into two groups fed a control diet with 10 % of energy from fat (CON, n 8) and a high-fat diet with 45 % of energy from fat (HF, n 16), respectively, for 9 weeks. The diet composition of the experimental diets is shown in Table 1. Thereafter, rats in the CON group were fed the control diet throughout the experiment, whereas the HF rats remained on the HF diet and were subsequently assigned to two subgroups (n 8). One HF group received a vehicle for comparison with high-fat diet gavage with NaB (HFB), and the other received 300 mg/kg NaB per rat via gavage every other day for 7 weeks. At the end of week 7, the rats were fasted overnight and euthanised with an overdose of sodium pentobarbital (50 mg/kg). Blood and liver samples were harvested and stored at −80°C. The gavage dosage (300 mg/kg NaB) was determined based on a previous study indicating that NaB at both 200 and 400 mg/kg effectively alleviates insulin resistance, fat accumulation and dyslipidaemia in rats with type 2 diabetes( Reference Khan and Jena 12 ). The treatment procedure followed the method used in our previous studies on HF-induced obese mice( Reference Hong, Jia and Pan 26 , Reference Jia, Hong and Li 27 ). According to a power and sample size analysis performed with an online Power and Sample Size Calculator (https://www.statisticalsolutions.net/pssZtest_calc.php), as well as a previous study( Reference Sun, Jia and Hong 28 ), six rats per group are sufficient to obtain reliable molecular data for the statistical analysis. All procedures with animals were approved by the Animal Ethics Committee of Nanjing Agricultural University (project number 2012CB124703). The sampling procedures followed the ‘Guidelines on Ethical Treatment of Experimental Animals’ (2006) no. 398 set by the Ministry of Science and Technology, China.
Table 1. Composition and nutrient content of the experimental diets
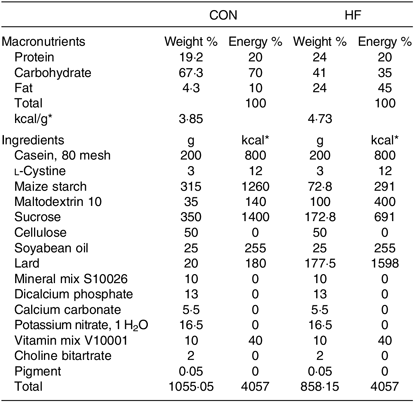
CON, control diet; HF, high-fat diet.
* To convert energy in kcal to kJ, multiply by 4·184.
Glucose tolerance test
Seven weeks after the NaB treatment (16 weeks after the beginning of the experiment), all rats were fasted for 12 h and then underwent a glucose tolerance test. Briefly, glucose was orally administered (1·5 g/kg body weight). Blood samples were collected sequentially from the tail vein, and glucose levels were tested at 15, 30, 45, 60, 90 and 120 min after gavage. Glucose levels were measured using a glucometer (AccuCheck II; Roche). To compare the time-course changes of the glucose concentration among groups, statistical analysis of AUC was performed using the GraphPad Prism software (GraphPad Software, Inc.).
Measurement of malondialdehyde, superoxide dismutase, and glutathione
Malondialdehyde, superoxide dismutase (SOD) and glutathione (GSH) were measured using respective commercial assay kits (no. A003-1, A001-3 and A006-1, Nanjing Jiancheng bioengineering Institute) according to the manufacturer’s instructions. Protein content was determined using a bicinchoninic acid protein assay kit (no. 23225, Thermo Scientific) according to the instructions provided by the manufacturer.
Cell culture and sodium butyrate treatment
Alpha mouse liver 12 (AML12) cells were routinely cultured in Dulbecco’s modified Eagle’s medium (Gibco, Life Technologies) supplemented with 10 % fetal bovine serum, 100 U/ml penicillin and 100 μg/ml streptomycin (Life Technologies/Gibco). The cells were grown at 37°C in a humidified incubator with 5 % CO2. At 24 h after seeding, cells were incubated with oleic acid (OA) (no. O1385-1, Sigma-Aldrich) with or without NaB (no. B5887-1, Sigma-Aldrich) for another 24 h.
Cell viability assay
Cell Counting Kit-8 (CCK-8) (no. FC101-01, TransGen, Beijing TransGen Bioengineering Institute) was used to determine the effects of OA( Reference Bell, Wang and Chen 29 ) and NaB( Reference Xing, Jiang and Tang 22 , Reference Ji, Zhou and Zhang 30 ) on cell viability at different doses, according to the manufacturer’s instructions.
Transfection of nuclear factor E2-related factor 2 small interfering RNA plasmid
AML12 cells were cultured at a confluency of 60–70 % in six-well plates and transfected with Nrf2-small interfering RNA (siRNA) or control-siRNA plasmids in Opti-MEM (no. 11058021, Gibco, Life Technologies) using Lipofectamine 2000 (no. 11668, Invitrogen) transfection reagent according to the manufacturer’s protocol.
Intracellular reactive oxygen species immunofluorescence staining
Intracellular reactive oxygen species (ROS) level was determined using a Reactive Oxygen Species assay kit (YEASEN, Technologies, Co., Ltd.). Briefly, the cells were incubated with MitoSOX for 20 min at 37°C with slight shaking for 5 min. Cell nuclei was labelled with 4,6-diamidino-2-phenylindole. After washing with serum-free culture medium, the cells were examined under a fluorescence microscope.
Evaluation of reactive oxygen species content in alpha mouse liver 12 cells
MitoSOX Red Mitochondrial superoxide indicator (Invitrogen, catalogue no. M36008), which indicates the mitochondrial superoxide levels, were used in the ROS staining. Briefly, stock solutions of MitoSOX Red were diluted in PBS and added to AML12 cells and incubated for 30 min at 37°C under 5 % CO2, followed by washing in Tissue Culture Medium-199 twice. Samples were then centrifuged for 5 min at 600 g , and the pellets were resuspended in 0·5 ml PBS and subsequently transferred to 5 ml fluorescence-activated cell sorting tubes. The MitoSOX Red fluorescence was then measured on a flow cytometer, according to the method described in a previous study( Reference He, Yin and Gong 31 ).
RNA isolation and quantitative real-time PCR
Total RNA was isolated from 30 mg liver samples with TRIzol reagent (Invitrogen) and reverse-transcribed according to the manufacturer’s protocol (Vazyme Biotech). Diluted cDNA (2 μl, 1:25) was used for real-time PCR that was performed in a Mx3000P System (Stratagene). Moreover, 18S was chosen as a reference gene to normalise the mRNA abundance of target genes. All primers were made by Generay Biotech and listed in Table 2. The 2−ΔΔ Ct method was used to analyse the real-time PCR data.
Table 2. The primer of genes for real-time PCR and chromatin immunoprecipitation (ChIP) analysis

GCLC, glutamate-cysteine ligase C; F, forward; R, reverse; GCLM, glutamate-cysteine ligase M; NQO-1, NAD(P)H:quinone oxidoreductase 1; HO-1, haeme oxygenase 1; Nrf2, nuclear factor E2-related factor 2.
Protein extraction and Western blot analysis
Total protein was extracted from 50 mg frozen liver samples as previously described( Reference Lee, Kao and Tsai 32 ). Protein concentrations were measured using the bicinchoninic acid protein assay kit (no. 23225, Thermo Scientific) according to the instructions provided by the manufacturer. Western blot analysis of protein kinase B (AKT) (no. 4685, Cell Signaling, diluted 1:500), phospho-protein kinase B (p-AKT) (no. 4060, Cell Signaling, diluted 1:500), insulin receptor substrate 1 (IRS1) (P35568, Bio-tech, diluted 1:1000), phosphoinositide 3-kinase (PI3K) (P27986, Bio-tech, diluted 1:500), Nrf2 (BS1258, Bioworld, diluted 1:500) and HDAC1 (BS6485, Bioworld, diluted 1:500) were carried out. The tubulin (BS1699, Bioworld, diluted 1:5000) and glyceraldehyde-3-phosphate dehydrogenase (GAPDH) (AP0063, Bioworld, diluted 1:5000) were used as internal control.
Chromatin immunoprecipitation assay
Chromatin immunoprecipitation (ChIP) was analysed as described in a previous study, with several modifications( Reference Jia, Cong and Li 33 ). Frozen liver samples (300 mg) in liquid N2 were homogenised and then washed with PBS containing Protease Inhibitor Cocktail (no. 11697498001; Roche). Cross-linking of protein and DNA was performed by adding formaldehyde to a final concentration of 1 %. The reaction was stopped subsequently with 2·5 mol/l glycine at room temperature. The reaction mixture resulting from the aforementioned procedure was centrifuged (5000 rpm for 5 min at 4°C) and pellets were washed with PBS and lysed in an SDS lysis buffer. Chromatin was sonicated to an average length ranging from 150–500 bp in length and pre-cleared with salmon sperm DNA-treated protein G agarose beads (40 μl, 50 % slurry, sc-2003). The mixture of pre-cleared chromatin preparations and 2 μg of primary antibody (H3 lysine 9 acetylation, ab4441, Abcam) were incubated overnight at 4°C. A negative control was included with normal IgG. Protein G agarose beads (40 μl, 50 % slurry, sc-2003; Santa Cruz) were added to capture the immunoprecipitated chromatin complexes. Finally, DNA fragments were released from the immunoprecipitated complexes by reverse cross-linking at 65°C for 1 h, and quantitative real-time PCR was used to quantify the fragments of target gene promoters with specific primers using purified immunoprecipitated DNA as the template (Table 2).
Statistical analysis
All data are presented as means with their standard errors. One-way ANOVA with a Bonferroni post hoc test was applied to estimate the differences among the three groups. P < 0·05 was considered statistically significant.
Results
Sodium butyrate reduces high-fat diet-induced body weight gain and improves glucose tolerance and insulin signalling pathway
Rats fed the high-fat diet had significantly (P < 0·05) higher body weight compared with rats fed the control diet, which was significantly (P < 0·05) reduced in the HFB group treated with NaB for 7 weeks (Fig. 1(A)). The increased serum glucose level in the HF group was partially (P = 0·053) alleviated by the NaB treatment (Fig. 1(B)), which was supported by normalised glucose tolerance (Fig. 1(C) and (D)). Although AKT protein expression level was not affected by the high-fat diet (Fig. 2(A) and (B)), NaB treatment restored the protein content of p-AKT, IRS1 and PI3K in the liver that was significantly decreased in the HF group (Figs. 2(C)–(H)).

Fig. 1. Sodium butyrate reduces body weight gain and improves glucose tolerance. (A) Body weight when sample collecting. (B) Fasting plasma glucose levels. (C) Mean blood glucose levels following glucose tolerance test (GTT) (n 6). (D) Area under the glucose–time curve (AUC). CON, normal (control) diet; HF, high-fat diet; HFB, high-fat diet gavage with sodium butyrate. Values are means, with standard errors represented by vertical bars. a,b Mean values with unlike letters were significantly different (P < 0·05).

Fig. 2. Sodium butyrate improves insulin signalling pathway. (A) and (B) Protein expression of protein kinase B (AKT) in liver (n 6). (C) and (D) Protein expression of phospho-AKT (p-AKT) in liver (n 6). (E) and (F) Protein expression of insulin receptor substrate 1 (IRS1) in liver (n 6). (G) and (H) Protein expression of phosphoinositide 3-kinase (PI3K) in liver (n 6). CON, normal (control) diet; HF, high-fat diet; HFB, high-fat diet gavage with sodium butyrate; GAPDH, glyceraldehyde-3-phosphate dehydrogenase. Values are means, with standard errors represented by vertical bars. a,b Mean values with unlike letters were significantly different (P < 0·05).
Sodium butyrate ameliorates high-fat diet-induced oxidative stress
The enzyme activity of GSH (Fig. 3(A)) and SOD (Fig. 3(B)) was significantly (P < 0·05) reduced in the HF group, which was completely restored (P < 0·05) in the HFB group. Also, NaB significantly (P < 0·05) abolished the increase of malondialdehyde content induced by high-fat diet in the HF group (Fig. 3(C)). Moreover, the decreased hepatic expression of GCLC, NQO-1 and HO-1 mRNA in the HF group was rescued by the NaB treatment in the HFB group (Figs. 3(D)–(F)). Although GCLM mRNA was not affected by the high-fat diet, it was significantly increased (P < 0·05) in NaB-treated obese rats (Fig. 3(G)).

Fig. 3. Sodium butyrate inhibits high-fat diet-induced oxidative stress. (A) Glutathione (GSH) enzyme activity in rat liver (n 6). (B) Superoxide dismutase (SOD) enzyme activity in rat liver. (C) Malondialdehyde (MDA) concentration in rat liver. (D) mRNA expression of glutamate-cysteine ligase C (GCLC) in rat liver. (E) mRNA expression of NAD(P)H:quinone oxidoreductase 1 (NQO-1) in rat liver. (F) mRNA expression of haeme oxygenase 1 (HO-1) in rat liver. (G) mRNA expression of glutamate-cysteine ligase M (GCLM) in rat liver. CON, normal (control) diet; HF, high-fat diet; HFB, high-fat diet gavage with sodium butyrate; prot, protein. Values are means, with standard errors represented by vertical bars. a,b Mean values with unlike letters were significantly different (P < 0·05).
Sodium butyrate suppresses histone deacetylase 1 expression and increases nuclear factor E2-related factor 2 histone acetylation and expression
The decreased Nrf2 expression at both protein (Fig. 4(A)) and mRNA (Fig. 4(C)) levels in the HF group was completely normalised in the HFB group. HDAC1 was significantly (P < 0·05) up-regulated in the HF group, which was reduced to control level by NaB treatment (Fig. 4(B)). Moreover, histone H3 acetyl K9 (H3K9Ac) detected on the Nrf2 gene promoter was significantly increased (P < 0·05) in the HFB group as compared with the HF group (Fig. 4(D)).

Fig. 4. Sodium butyrate suppresses histone deacetylase 1 (HDAC1) expression and increases nuclear factor E2-related factor 2 (Nrf2) histone acetylation. (A) Protein expression of Nrf2 in liver (n 6). (B) Protein expression of HDAC1 in liver (n 6). (C) mRNA expression of Nrf2 in rat liver (n 6). (D) Histone modifications on the gene promoter of Nrf2 (n 3). CON, normal (control) diet; HF, high-fat diet; HFB, high-fat diet gavage with sodium butyrate; H3K9Ac, histone H3 acetyl K9. Values are means, with standard errors represented by vertical bars. a,b Mean values with unlike letters were significantly different (P < 0·05).
Small interfering RNA against Nrf2 (siNrf2) blocks the effect of sodium butyrate on oxidative stress alleviation
AML12 cells were treated with different levels of NaB and OA. NaB at 5 and 10 mM and OA at 500 μM significantly (P < 0·05) decreased cell viability (Figs. 5(A) and (B)). OA treatment at 400 μM significantly (P < 0·05) increased TAG content in AML12 cells. NaB at 0·5 mM tended to (P = 0·08) alleviate the increased TAG content, while NaB at 1 mM significantly (P < 0·05) alleviated OA-induced increase in TAG content (Fig. 5(C)). Therefore, OA at 400 μM and NaB at 1 mM were used in further analysis. NaB significantly alleviated OA-induced increase in ROS production, whereas Nrf2 knockdown blocked the alleviating effect of NaB (Figs. 5(D) and (E)). Concurrently, Nrf2 knockdown abolished the effect of NaB on restoring the Nrf2 mRNA expression that was suppressed by OA (Fig. 5(F)). In addition, GSH and SOD activities were decreased significantly (P < 0·05) in the OA group, which was completely (P < 0·05) rescued by NaB treatment. However, Nrf2 knockdown blocked the restoration effect of NaB on GSH and SOD activities (Figs. 5(G) and (H)). Similarly, the alleviation effects of NaB on GCLC, HO-1 and NQO-1 mRNA expression were significantly abolished (P < 0·05) in the Nrf2 knockdown group (Fig. 5(I)).

Fig. 5. Small interfering RNA against nuclear factor E2-related factor 2 (siNrf2) reverses the effect of sodium butyrate (NaB) alleviating oxidative stress. (A) Cell activity treated with different doses of NaB. (B) Cell activity treated with different doses of oleic acid (OA). (C) Content of TAG in alpha mouse liver 12 (AML12) cells. (D) Immunofluorescence staining of reactive oxygen species (ROS) marker MitoSOX in AML12 cells. (E) ROS concentration in AML12 cells. (F) mRNA expression of Nrf2 in AML12 cells. (G) Glutathione (GSH) enzyme activity in AML12 cells. (H) Superoxide dismutase (SOD) enzyme activity in AML12 cells. (I) mRNA expression of glutamate-cysteine ligase C (GCLC), glutamate-cysteine ligase M (GCLM), haeme oxygenase 1 (HO-1) and NAD(P)H:quinone oxidoreductase 1 (NQO-1). CON, normal (control) diet; DAPI, 4′,6-diamidino-2-phenylindole; prot, protein. Values are means, with standard errors represented by vertical bars. a,b,c Mean values with unlike letters were significantly different (P < 0·05).
Discussion
Butyrate is an SCFA formed in the cecum and colon through fermentation of dietary fibre, which has been demonstrated to protect against obesity( Reference Brahe, Astrup and Larsen 34 ) and insulin resistance( Reference Gao, Yin and Zhang 14 , Reference Chriett, Zerzaihi and Vidal 35 ). Most previous studies show that butyrate has a preventive effect when administered during the induction process of a disease model( Reference Gao, Yin and Zhang 14 , Reference Henagan, Stefanska and Fang 17 ). However, the efficacy of butyrate in the treatment of obesity and insulin resistance in established obese models is rarely investigated. The model established in the present study better simulates adult-onset obesity in humans. In agreement with previous studies, the effects of NaB on high-fat diet-induced obesity and insulin resistance were observed in this model, as indicated by decreased body weight and glucose levels as well as improved glucose tolerance( Reference Gao, Yin and Zhang 14 ).
Oxidative stress induces insulin resistance by impairing insulin signal transduction( Reference Houstis, Rosen and Lander 36 ). Previous studies have shown that administering NaB attenuates oxidative stress in many tissues( Reference Ma, Abaker and Bilal 37 , Reference Hamer, Jonkers and Bast 38 ) and cells( Reference Li, Wang and Nie 39 ). PI3K and p-AKT play key roles in insulin signalling pathway. NaB increases PI3K and p-AKT protein levels( Reference Li, Wang and Nie 39 ) and alleviates the oxidative status in sub-acute ruminal acidosis through partial activation of antioxidant enzyme genes( Reference Ma, Abaker and Bilal 37 ). In agreement with these previous findings, we found that NaB restored the hepatic expression of IRS1, PI3K and p-AKT that was down-regulated by high-fat diet. Additionally, the reduced activities of the antioxidant enzymes GSH and SOD and the repressed expression of phase II detoxifying GCLC, GCLM, HO-1 and NQO-1 genes in the HF group were all restored in the HFB group.
Nrf2 is a central regulator of oxidative stress that transactivates the expression of antioxidant proteins to protect against oxidative damage triggered by injury and inflammation( Reference Itoh, Wakabayashi and Katoh 40 ). A deficiency of Nrf2 exacerbates obesity-induced oxidative stress, neuroinflammation and cognitive decline in mice( Reference Tarantini, Valcarcel-Ares and Yabluchanskiy 41 ). As a major nutraceutical component of green tea, epigallocatechin-3-gallate evokes Nrf2 nuclear translocation to ameliorate oxidative stress and insulin resistance in mice( Reference Mi, Zhang and Tian 42 ). Moreover, Endo et al. reported that MIYAIRI 588, a butyrate-producing probiotic, suppresses hepatic oxidative stress through enhanced Nrf2 expression by precluding ubiquitination and increasing the half-life of the Nrf2 protein( Reference Endo, Niioka and Kobayashi 43 ). In line with these results, NaB increased hepatic expression of Nrf2 at both mRNA and protein levels in the present study. We observed that the actions of NaB were completely abolished when Nrf2 was knocked down in vitro. Therefore, these results demonstrate that Nrf2 plays a key role in mediating the protective effect of NaB against high-fat diet-induced oxidative stress in the liver.
Butyrate exerts its biological effects mainly by activating G-protein-coupled receptors (GPR) or acting as an HDAC inhibitor to regulate gene expression by modulating histone acetylation. NaB may facilitate the transcription of 2 % of mammalian genes by inhibiting HDAC( Reference Davie 25 ). It is reported that NaB increases histone H3 acetylation on chromatin in proximity of IRS1 transcriptional start site, thereby increasing the expression of IRS1 and insulin sensitivity( Reference Chriett, Zerzaihi and Vidal 35 ). However, expression of GPR43 and GPR41, which are known to act as receptors for butyrate, were unchanged by NaB treatment in the present study (data not shown). Instead, NaB effectively normalised hepatic expression of the HDAC1 protein that was augmented in HF rats. We employed a ChIP analysis to detect H3K9Ac, a gene activation marker, on the Nrf2 promoter. H3K9Ac level was decreased on the Nrf2 promoter in the liver of obese rats, while NaB restored this activation marker, leading to up-regulation of Nrf2 expression and thereby transcriptional activation of its downstream antioxidant genes. Previous studies have shown that NaB alleviates H2O2-induced oxidative stress by increasing the phosphorylate glycogen synthase kinase 3β level and promoting the nuclear translocation of Nrf2 in HepG2 cells, which increases the expression of downstream antioxidant enzymes( Reference Xing, Jiang and Tang 22 ). A previous study indicated that MIYAIRI 588 up-regulates Nrf2 at the post-translational level by precluding ubiquitination to improve half-life of Nrf2 protein( Reference Endo, Niioka and Kobayashi 43 ); here we show that NaB regulates Nrf2 expression at the transcriptional level by inhibiting the HDAC1 activity.
In summary, our data provide a novel insight that NaB attenuates high-fat diet-induced oxidative stress and insulin resistance in the liver. Moreover, the present study indicates that Nrf2 is epigenetically regulated by NaB through inhibiting HDAC1 (Fig. 6). Therefore, Nrf2 may be a potential therapeutic target to treat insulin resistance. Future clinical investigations are needed to evaluate the potential application of NaB and its derivatives in the treatment of adult-onset obesity and insulin resistance in humans.

Fig. 6. A schematic diagram illustrates sodium butyrate (NaB) alleviates high-fat diet-induced oxidative stress through promoting expression of nuclear factor E2-related factor 2 (Nrf2). NaB acts as a histone deacetylase inhibitor to up-regulate Nrf2 expression with enhanced histone H3 acetyl K9 (H3K9Ac) modification on its promoter which improves expression of its downstream antioxidant enzyme genes. NQO-1, NAD(P)H:quinone oxidoreductase 1; HO-1, haeme oxygenase 1; GCLC, glutamate-cysteine ligase C; GCLM, glutamate-cysteine ligase M; HDAC1, histone deacetylase 1; ARE, antioxidant response element.
Acknowledgements
The present study was supported by the National Key Research and Development Program of China (2016YFD0500502), the National Basic Research Program of China (2012CB124703), the Priority Academic Program Development of Jiangsu Higher Education Institutions (PAPD), and Jiangsu Collaborative Innovation Center of Meat Production and Processing, Quality and Safety Control.
B. S. contributed to experiments and data analysis, discussion and writing of the manuscript. Y. J., S. Y., N. Z., Y. H., J. H. and S. G. provided technical support. R. Z. contributed to design of the study and critical revision of the manuscript.
The authors declare that there is no conflict of interest associated with this article.