Introduction
Acute interstitial pneumonia (AIP) is one of a wider family of diseases known as interstitial lung diseases (ILD). Using its most broad definition, an ILD can include any condition that primarily or secondarily affects the interstitium of the lung – the tissue that comprises the alveolar and interlobular septa. While this family of diseases is diverse and heterogeneous, the scope of this review will predominantly focus on ILD's that induce the disease through acute and diffuse damage to alveolar epithelial cells with a primary focus on the pathological characteristics of AIP in feedlot cattle. AIP is one of the most common causes of feedlot mortalities. Losses to AIP have a significant deleterious effect on the health and well-being of feedlot cattle as well as on the overall profitability of the beef industry. The tendency of AIP to affect the most valuable animals in the feedlot population (i.e. well-conditioned animals late in the feeding period), along with the relative lack of understanding regarding its pathogenesis in the feedlot environment, and the paucity of effective control measures, highlights the need for further research into this topic.
Acute ILDs most commonly elicit a histomorphologic pattern known as diffuse alveolar damage (DAD), resulting from injury to pneumocytes and/or vascular endothelium. The potential triggers for initiation of DAD are myriad but, regardless of the cause, the disease usually progresses through 3 defined phases (Caswell and Williams, Reference Caswell, Williams and Maxie2016). First, an acute exudative phase is elicited as damage to type I and II pneumocytes and vascular endothelium occurs. This leads to leakage of interstitial fluid and plasma proteins into alveoli. This phase correlates to the histological findings of proteinaceous alveolar fluid and hyaline membranes consisting of heterogenous amalgamations of polymerizing fibrin, other plasma and surfactant proteins, and cellular proteins and debris. The accumulation of fluid is worsened by damage to the pneumocytes, which normally form a barrier to fluid leakage from the interstitium and use aquaporin channels to resorb fluid from the alveolus. Furthermore, the presence of protein in the alveolar lining fluid interferes with surfactant function, thereby reducing lung compliance. Surfactant dysfunction can be further compounded by the production of secretory phospholipase A2 in the early phases of disease which degrades the phophatidylglycerol molecules within the surfactant. The subacute proliferative phase is initiated when type II pneumocytes proliferate to repair damaged alveolar epithelium. However, type II pneumocytes are poorly suited for effective gas exchange and, if the injurious stimulus persists, differentiation of these cells into functional type I pneumocytes will be halted or hindered. Progression to a chronic stage involves either organization of fibrinous alveolar exudates into fibrous tissue and/or the production of fibrosis-promoting cytokines by interstitial macrophages and fibroblasts. Compared to other forms of ILD in domestic animals, DAD is well characterized, and its many causes are summarized in Table 1. While a comprehensive description of ILDs is outside the scope of this review, others have highlighted the comparative lack of characterization in other forms of veterinary ILDs. Recently, a system modified from that used in human ILD patients was used to classify canine and feline ILDs into three groups: idiopathic interstitial pneumonias (IIP), ILDs secondary to known causes, and miscellaneous ILDs (Travis et al., Reference Travis, King and Bateman2002; Reneiro, Reference Reinero2019a, Reference Reinero2019b). Under this system, an entity referred to as AIP is classified as an IIP, and is distinguished by its acute onset, histological lesions of DAD, and its temporal homogeneity consistent with a single initiating insult.
Table 1. Causes of acute interstitial lung disease
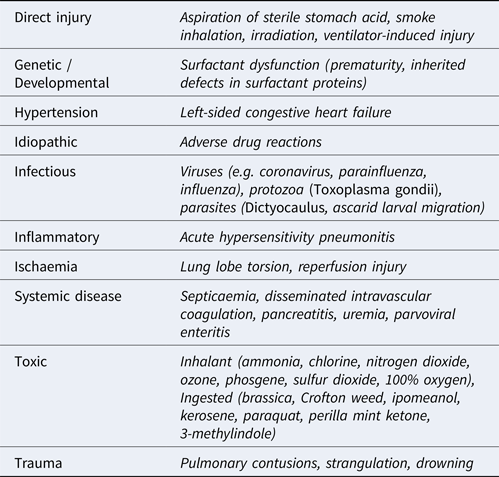
Acute interstitial pneumonia in pastured cattle (Fog Fever)
AIP, also known as atypical interstitial pneumonia, acute bovine pulmonary emphysema and edema, and colloquially as fog fever, is an acute ILD principally recognized in mature pastured cattle grazing the lush growth that follows haylage or silage harvesting (Blood, Reference Blood1962). Historically, the disease has been associated with abrupt transitions onto lush pasture, particularly if previous forage was of low quality; experimental replications of this scenario have substantiated this association (Hammond et al., Reference Hammond, Bradley, Yokoyama, Carlson and Dickinson1979).
The earliest clinical characterizations of AIP described two forms: acute and chronic (Blood, Reference Blood1962). The acute form is typified by a sudden onset of shallow, rapid breathing accompanied by a loud expiratory grunt, and with affected animals usually dying within 2–3 days of onset. Surviving animals may progress to a chronic stage characterized by longstanding congestive heart failure and ill thrift. Additionally, Blood, (Reference Allen1962) described secondary bacterial bronchopneumonia as a major feature of the chronic form of AIP, but work in feedlot cattle suggests that bronchopneumonia may be more likely to precede, and potentially precipitate, the onset of AIP (Loneragan et al., Reference Loneragan, Gould, Mason, Garry, Yost, Miles, Hoffman and Mills2001b; Woolums et al., Reference Woolums, Mason, Hawkins, Brown, Williams, Gould, Fox, Sturgeon, Anderson, Duggan, Sanchez, Barrett and Chitwood2004). In groups of AIP-affected animals, morbidity may exceed 50% and the case fatality rate ranges from 30 to 100% (Doster, Reference Doster2010).
The proposed causes of AIP in pastured cattle are numerous but many of the known etiologies are too rare or circumstantially specific to explain the high frequency of AIP. Examples include exposures to noxious gases such as silo gas, nitrogen dioxide, chlorine, and zinc dioxide (Cutlip, Reference Cutlip1966; Macdonald et al., Reference MacDonald, Lamoureux, Van den Brink, Whenham and Brisbane1971; Brightwell, Reference Brightwell1972; Hilderman and Taylor, Reference Hilderman and Taylor1974). Hypersensitivity-mediated mechanisms have also been previously proposed as a cause of AIP in pastured cattle with incriminated antigens including fungal organisms from moldy hay, Dictyocaulus viviparus, Ascaris suum, and Ascaris lumbricoides; however, these etiologies either lack experimental evidence or produce histological lesions that are distinct from those observed in AIP (Allen, Reference Allen1962; Greenway and McCraw, Reference Greenway and McCraw1970; Pirie et al., Reference Pirie, Dawson, Breeze, Selman and Wiseman1971; Breeze et al., Reference Breeze, Pirie, Selman and Wiseman1974).
The most convincing evidence for AIP etiology stems from studies investigating it as a pneumotoxicant-mediated disease. Compounds such as 4-ipomeanol from moldy sweet potatoes infected with Fusarium solani and 3-furyl isoamyl ketone from Perilla frutescens (perilla mint) have reliably induced AIP both naturally and experimentally (Doster et al., Reference Doster, Mitchell, Farrell and Wilson1978; Garst et al., Reference Garst, Wilson, Kristensen, Harrison, Corbin, Simon, Philpot and Szabo1985; Medeiros et al., Reference Medeiros, Simões, Tabosa, Nóbrega and Riet-Correa2001). Despite this, a different toxicant – 3-methylindole (3-MI) – is considered to be the putative cause of most AIP cases in pastured cattle. 3-MI was first implicated in the pathogenesis of AIP during a study in which oral administration of D- and L-tryptophan was incidentally found to induce AIP, with later studies identifying the L-isomer as the causative compound (Dickinson et al., Reference Dickinson, Spencer and Gorham1967; Carlson et al., Reference Carlson, Yokoyama and Dickinson1972; Yokoyama et al., Reference Yokoyama, Carlson and Dickinson1975). Subsequent studies then highlighted the prominent role of the ruminal microbiome in the development of AIP. A plausible biochemical pathway from L-tryptophan ingestion to 3-MI toxicosis has been demonstrated in which ruminal bacteria are capable of fermenting tryptophan to indoleacetic acid (IAA) and then subsequently decarboxylating this compound into 3-MI by means of Lactobacillus sp. bacteria (Yokoyama et al., Reference Yokoyama, Carlson and Holdeman1977; Carlson and Breeze, Reference Carlson and Breeze1984). This 3-MI-producing species of Lactobacillus sp., which has been referred to as Lactobacillus sp. strain 11201, remains to be fully characterized. Production of 3-MI by this species is evidently dependent on other members of the ruminal microbiome as this species, which uses IAA as its substrate, is not (in isolation) capable of executing the entire biochemical pathway from L-tryptophan to 3-MI. Other bacterial species isolated from swine manure such as Olsenella scatoligenes and Clostridium spp. (namely C. drakei and C. scatologenes) have also demonstrated the ability to decarboxylate IAA to 3-MI; in fact, the latter species, unlike Lactobacillus sp. strain 11201, are capable of 3-MI production regardless of whether it is incubated with IAA or L-tryptophan (Whitehead et al., Reference Whitehead, Price, Drake and Cotta2008; Li et al., Reference Li, Jensen, Højberg, Canibe and Jensen2015). Thus, it seems the composition of the ruminal microbiome may be the most important factor in the development of AIP, especially since there appears to be no correlation between pasture tryptophan levels and disease development (Mackenzie et al., Reference Mackenzie, Ford and Scott1975). Rather, it seems likely that there is a minimal threshold of L-tryptophan levels in pasture (such as those encountered in lush growth), above which the risk of AIP development is largely determined by the composition of the ruminal microbiome. The necessity for L-tryptophan degradation by ruminal microbes in the pathogenesis of AIP was further illustrated by studies in which intravenous administration of L-tryptophan or IAA failed to provoke respiratory damage, whereas intravenous administration of 3-MI rapidly induced clinical disease (Carlson et al., Reference Carlson, Yokoyama and Dickinson1972; Pirie et al., Reference Pirie, Breeze, Selman and Wiseman1976). Further implicating the ruminal production of 3-MI as key feature of AIP pathogenesis in pastured cattle, it has been demonstrated that ruminal fluid from cattle affected by AIP frequently contains 3-MI levels in excess of what is required to elicit clinical disease in experimental settings (Hammond et al., Reference Hammond, Bradley, Yokoyama, Carlson and Dickinson1979).
3-Methylindole as a cause of acute interstitial pneumonia
3-MI – molecular formula C9H9N – is a naturally occurring organic compound of the indole family; it has a molecular weight of 131.17 g mol−1, a boiling point of 266°C, a melting point of 97.5°C, and a strong fecal odor at high concentrations (Budavari, Reference Budavari1996; Pubchem, 2019). As a family, indoles are characterized by their bicyclic structure consisting of a fused benzene and pyrrole ring. Indoles are recognized as an essential factor in bacterial physiology, acting as intercellular signaling molecules that regulate spore formation, plasmid stability, anti-microbial resistance, biofilm formation, and virulence (Lee and Lee, Reference Lee and Lee2010).
In mammalian biology, 3-MI occurs naturally as a by-product of tryptophan metabolism within the gastrointestinal tract. While the primary focus of this review is on the role of 3-MI as a pneumotoxicant, particularly as it pertains to the development of AIP in ruminants, this compound has also been exhaustively researched for its roles in other biological systems. Firstly, 3-MI (also known as skatole) is recognized as one of two major malodorants that contribute to boar taint, a major issue in the pork industry, in which excessive levels of 3-MI and androstenone in the adipose tissues of uncastrated males bestows an undesirable odor to the meat (Wesoly and Weiler, Reference Wesoly and Weiler2012; Squires et al., Reference Squires, Bone and Cameron2020). Various methods to reduce the prevalence and/or severity of boar taint in entire males have been explored including dietary modification, vaccination against sex-regulating hormones (immunocastration), flavor masking, and genetic selection (Aaslyng and Koch, Reference Aaslyng and Koch2018; Drag et al., Reference Drag, Hansen and Kadarmideen2018; Heyrman et al., Reference Heyrman, Millet, Tuyttens, Ampe, Janssens, Buys, Wauters, Vanhaecke and Aluwé2018; Han et al., Reference Han, Zhou, Cao, Du, Meng, Bu, Kong, Huang and Zeng2019; Morlein et al., Reference Mörlein, Meier-Dinkel, Gertheiss, Schnäckel and Mörlein2019). However, despite the apparent ample production of 3-MI that occurs in porcine physiology, 3-MI-mediated respiratory disease has not been documented in pigs. This observation may reflect important species differences in which the cytochrome P450 isoforms capable of metabolizing 3-MI into pneumotoxic metabolites are abundant in ruminants but absent in pigs (Diaz and Squires, Reference Diaz and Squires2000; Wiercinska et al., Reference Wiercinska, Lou and Squires2012). In rodents and horses, 3-MI has been recognized as a cause of injury to both olfactory and bronchial mucosa when administered by either oral or intra-peritoneal routes (Turk et al., Reference Turk, Breeze and Gallina1983; Kratskin et al., Reference Kratskin, Hummel, Hastings and Doty2000; Miller and O'Bryan, Reference Miller and O'Bryan2003; Miller et al., Reference Miller, Kottler, Ramos-Vara, Johnson, Ganjam and Evans2003). Additionally, the potent insect attractant properties of 3-MI have been utilized to develop chemically baited ovitraps for some species of mosquito (Baak-Baak et al., Reference Baak-Baak, Rodríguez-Ramírez, García-Rejón, Ríos-Delgado and Torres-Estrada2013).
Biochemistry and metabolism of 3-methylindole in ruminants
When ruminants ingest feeds containing the essential amino acid tryptophan, specifically its L-isomer, ruminal degradation results in the production of 3-MI. Upon ingestion of L-tryptophan, it initially undergoes deamination into IAA and, once IAA is formed, ruminal bacteria such as Lactobacillus sp. are then responsible for its decarboxylation into 3-MI (Hammond and Carlson, Reference Hammond and Carlson1980; Carlson and Breeze, Reference Carlson and Breeze1984). In cattle, it is estimated that approximately 23% of ingested tryptophan undergoes conversion into 3-MI (Hammond et al., Reference Hammond, Huntington and Breeze1983). In vitro studies targeted at preventative therapeutics for AIP have documented the ability of certain feed additives, such as monensin, to drastically reduce the conversion of L-tryptophan into 3-MI by the ruminal microbiome (Hammond et al., Reference Hammond, Carlson and Breeze1978). Similarly, in feedlot cattle populations at high risk of metabolic disease, higher dosages of monensin (⩾44 ppm dry matter basis) can reduce mortality from metabolic diseases including AIP (unpublished observations, Kent Fenton, Feedlot Health Management Services, Okotoks, Alberta). 3-MI is a lipophilic compound that is readily absorbed into the bloodstream via the ruminal mucosa. The vast majority of 3-MI is absorbed in the forestomachs: following oral administration of 0.4 g of tryptophan/kg of bodyweight, only 1.0% of the total 3-MI produced as a result of ruminal degradation was detected in the duodenum (Hammond et al., Reference Hammond, Glenn, Huntington and Breeze1984). Using blood samples obtained directly from the portal circulation, the absorption rates of 3-MI from ruminal fluid have been estimated to be as high as 1.05 g hr−1 with approximately 29% of all ingested tryptophan being accounted for in the form of 3-MI within 48 h of ingestion (Hammond et al., Reference Hammond, Huntington and Breeze1983). Once within the bloodstream, 3-MI is rapidly removed. Over 80% of absorbed 3-MI is excreted via the urine within 24 h. While the remaining 3-MI does not appear to selectively concentrate in the lungs of ruminants, this tissue does appear to accumulate higher levels of 3-MI metabolites when compared to the liver, kidney and other selected tissues (Bradley and Carlson, Reference Bradley and Carlson1982). This finding, along with those of similar studies, suggests that there is preferential metabolism of 3-MI in the lung tissues of ruminants when compared to other tissues and to other species (Nocerini et al., Reference Nocerini, Carlson and Yost1985a, Reference Nocerini, Yost, Carlson, Liberato and Breeze1985b).
The cytochrome P450 (CYP450) family has been heavily implicated in the metabolism of 3-MI in many species, including humans (Ruangyuttikarn et al., Reference Ruangyuttikarn, Appleton and Yost1991; Thornton-Manning et al., Reference Thornton-Manning, Nichols, Manning, Skiles and Yost1993; Wiercinska et al., Reference Wiercinska, Lou and Squires2012). The mechanisms by which 3-MI exerts its pneumotoxicity will be elaborated later but, in brief, it is believed that bioactivation by specific CYP450 enzymes results in the production of a pneumotoxic methylene imine electrophile called 3-methyleneindolenine (3-MEIN) (Nocerini et al., Reference Nocerini, Carlson and Yost1985a, Reference Nocerini, Yost, Carlson, Liberato and Breeze1985b). However, 3-MEIN is not the only potential metabolite of 3-MI. The precise metabolite that is produced from 3-MI is strongly dependent on the specific CYP450 enzyme that effectuates its metabolism. For example, depending on the participating CYP450 enzyme, potential metabolite pathways include hydroxylation to indole-3-carbinol or 6-hydroxy-3-methylindole, ring epoxidation to 3-methyloxindole, or dehydrogenation to 3-MEIN. Importantly, it has been demonstrated that the affinity of a CYP450 enzyme to execute a particular molecular alteration is highly governed by the specific isoform of the involved enzyme and the presence of co-factors that enhance 3-MI metabolism (e.g. cytochrome b5A) (Thornton-Manning et al., Reference Thornton-Manning, Appleton, Gonzalez and Yost1996; Wiercinska et al., Reference Wiercinska, Lou and Squires2012).
Mechanisms of 3-methylindole pneumotoxicity in ruminants
The organ- and species-specific toxicity of 3-MI in the lungs of ruminants appears to be a product of selective expression of CYP450 enzymes that are capable of carrying out the necessary dehydrogenation of 3-MI into the pneumotoxic 3-MEIN (Gram, Reference Gram1997). Several CYP450 enzymes have been investigated for their potential role in 3-MI bioactivation. Orthologs from several species have been found to be capable of catalysing the 3-MI to 3-MEIN reaction; these include goat CYP2F3, human CYP2F1, human CYP2A6, human CYP1A1, human CYP1A2, mouse CYP1A2, and rabbit CYP4B1. However, many of these enzymes preferentially produce much greater levels of alternative 3-MI metabolites than of 3-MEIN. That being said, the CYP2F enzymes have been identified as a subfamily that exclusively produces 3-MEIN, while also being selectively expressed in the lungs (Thornton-Manning et al., Reference Thornton-Manning, Appleton, Gonzalez and Yost1996; Wang et al., Reference Wang, Lanza and Yost1998; Lanza and Yost, Reference Lanza and Yost2001). These observations implicate enzymes such as CYP2F3 and CYP2F1 as potential contributors to the pneumotoxic properties of 3-MI in ruminants. Illustrating the pivotal role of CYP450 enzymes in 3-MEIN-mediated cytotoxicity, in vitro experiments utilizing goat lung microsomes have demonstrated increases in covalent binding of 3-MEIN to endogenous proteins following incubation with compounds known to induce CYP450 activity, and an inverse effect when incubated with CYP450 inhibitors (Merrill and Bray, Reference Merrill and Bray1983; Huijzer et al., Reference Huijzer, Adams, Jaw and Yost1989). Once 3-MEIN is produced, its electrophilic properties permit covalent binding with cellular macromolecules, forming adducts with proteins and nucleic acids that precipitate cytotoxicity (Ruangyuttikarn et al., Reference Ruangyuttikarn, Skiles and Yost1992). Another factor that seemingly contributes to the extent of 3-MEIN cytotoxicity is the presence of nucleophilic conjugating agents such as glutathione. Such compounds reduce the covalent binding of reactive 3-MI metabolites to cellular proteins by conjugating the metabolites during phase 2 metabolism (Bray et al., Reference Bray, Carlson and Nocerini1984; Nocerini et al., Reference Nocerini, Carlson and Yost1985a, Reference Nocerini, Yost, Carlson, Liberato and Breeze1985b). In vivo studies suggest that lung levels of glutathione are inversely correlated with 3-MI pneumotoxicity (Kubow and Bray, Reference Kubow and Bray1988). However, dietary supplementation with antioxidants (such as vitamin E) that work synergistically with glutathione, and meal that is high in glutathione precursors (i.e. cysteine in feather meal), has failed to demonstrate an economically viable protective effect against AIP when used in a commercial feedlot setting (Stanford et al., Reference Stanford, McAllister, Ayroud, Bray and Yost2007).
Enzymatic interactions with 3-methylindole
Several studies have demonstrated the ability of 3-MI to not only act as an enzyme substrate, but also as an inducer of certain types of enzymes. Indeed, 3-MI induces the expression of the same enzymes that catalyzes its metabolism. Of particular interest are the interactions of 3-MI with CYP450 and prostaglandin H synthase enzymes, respectively.
In addition to the role of CYP450 enzymes in the metabolism of 3-MI, a reciprocal dependency of certain CYP450 enzymes may also exist. Studies performed in both animals and humans have illustrated the capability of 3-MI to induce the transcription and expression of some subtypes of CYP450 enzymes in an in vitro setting. In pigs, CYP2E1 and CYP2A6 have both been shown to be induced by 3-MI which, in turn, also acts as a substrate for these enzymes. Interestingly, these same enzymes are inhibited by the testicular steroidal hormone androstenone, thus providing a possible mechanism for the accumulation of 3-MI in the adipose tissues of boars – also known as boar taint (Doran et al., Reference Doran, Whittington, Wood and McGivan2002; Chen et al., Reference Chen, Cue, Lundstrom, Wood and Doran2008). Similarly, in humans, CYP1A1, CYP1A2, CYP1B1 and CYP2F1 have all been shown to be induced by 3-MI exposure. Additionally, the mechanisms of induction do not appear to be uniform across all CYP450 subfamilies. While CYP1A1, CYP1A2 and CYP1B1 expression is tied to the agonistic capabilities of 3-MI on the transcription factor aryl hydrocarbon receptor (AHR), antagonism of this receptor does not inhibit the transcription of CYP2F1 (Weems and Yost, Reference Weems and Yost2010; Rasmussen et al., Reference Rasmussen, Balaguer, Ekstrand, Daujat-Chavanieu and Gerbal-Chaloin2016). In addition to AHR, several other nuclear receptors, including constitutive androstane receptor, pregnane X receptor, and farnesoid X receptor, have demonstrated their capability to upregulate the expression of numerous CYP450 isoforms following exposure of porcine hepatocytes to 3-MI (Gray and Squires, Reference Gray and Squires2013). Conversely, and further highlighting the complexity of 3-MI and CYP450 enzyme interactions, recent work has also described the ability of 3-MI to inhibit the function of certain CYP450s. For example, the activity of human CYP11A1 – a steroid-synthesizing enzyme – is significantly reduced by sub-physiological concentrations of 3-MI, a process that may contribute to pathogenesis of chronic inflammatory bowel diseases in humans (Mosa et al., Reference Mosa, Gerber, Neunzig and Bernhardt2016). Clearly, the interactions between 3-MI and the numerous CYP450 isoforms are highly complex, variable, and warrant further investigation.
Prostaglandin H synthase (PHS) is another enzyme that is capable of affecting the metabolism of 3-MI. PHS is a vital component in biological systems; using polyunsaturated fatty acids (PUFAs) – such as arachidonic acid – as substrates, this membrane-bound enzyme is chiefly responsible for the production of prostanoids (prostaglandin, prostacyclin, and thromboxane). PHS effectuates the PUFAs to prostanoid conversion via two major reactions: an initial oxidation performed by a cyclooxygenase component, followed by reduction by a hydroperoxidase component (Bray and Kirkland, Reference Bray and Kirkland1990). This sequence of reactions necessitates a reducing agent to act as an electron donor for the hydroperoxidase-mediated reduction reaction. Thus, it has been demonstrated that 3-MI is capable of significantly increasing the activity of PHS by acting as the necessary reducing agent needed for the synthesis of prostanoids – a process that presumably also facilitates the dehydrogenation of 3-MI into 3-MEIN. This finding has been replicated in both goat lung and ram seminal vesicle microsomes, the latter being rich in PHS with comparatively minimal CYP450 enzymes. Prostaglandin synthesis was found to increase by as much as 70% in the presence of 3-MI in the study using goat lung microsomes and, in both experiments, an unidentified reactive intermediate capable of covalently binding microsomal proteins was produced as a result of the dehydrogenation of 3-MI. Additionally, these studies established that PHS-mediated bioactivation of 3-MI was significantly greater in goat lung microsomes when compared to goat liver microsomes, and that this alternative route of 3-MI bioactivation was independent of the process catalysed by CYP450 enzymes (Formosa and Bray, Reference Formosa and Bray1988; Formosa et al., Reference Formosa, Bray and Kubow1988). In keeping with this, in an experimental setting, PHS inhibitors – such as aspirin – appeared to be protective against AIP when administered prior to, but not after, intravenous injection of 3-MI (Acton et al., Reference Acton, Boermans and Bray1992). However, this effect has proven difficult to replicate in a clinical setting (Loneragan et al., Reference Loneragan, Morley, Wagner, Mason, Yost, Thoren, Wittum and Bray2002). Clearly, further work is needed to characterise the respective contributions of PHS and CYP450s to AIP pathogenesis.
Acute interstitial pneumonia in feedlot cattle
AIP is frequently diagnosed in feedlot cattle without exposure to forages traditionally associated with AIP in pastured cattle. As such, the precise etiology of AIP in feedlot cattle has, to date, been described in less detail and appears to correlate with a variety of potential predisposing factors. Parallels have been drawn to the disease as it occurs in pastured cattle but, given the fundamental differences in nutrition and management, unifying pathogenesis seems unlikely. Nonetheless, it is generally thought that 3-MI or a similar pneumotoxicant may be responsible.
Health and economic impacts
Comprehensive surveys have underlined the health and economic impacts of AIP in feedlot systems. For the purposes of these surveys, and by a general convention, AIP is considered a separate entity to bovine respiratory disease complex (BRDC), the latter encompassing the myriad of infectious causes of respiratory disease in cattle. Feedlot surveys reported AIP as the second most common disease requiring treatment behind BRDC and the third most common cause of mortality after BRDC and gastrointestinal disease – affecting 1.28% (morbidity) and contributing to the deaths of 0.13% (mortality) of all placed cattle, respectively (Woolums et al., Reference Woolums, Loneragan, Hawkins and Williams2005a, Reference Woolums, Loneragan, Hawkins and Williams2005b). These findings resembled those of the United States Department of Agriculture National Animal Health Monitoring 2011 survey, which found that AIP occurred in 2.8% of all placed cattle with 60.2% of affected cattle receiving treatment at an average cost of $21.70 per treatment (USDA, 2011). The epidemiological features of AIP will be discussed in greater detail later; however, it is worth highlighting that the economic repercussions of AIP losses are amplified by the tendency to occur late in the feeding period (Ayroud et al., Reference Ayroud, Popp, VanderKop, Yost, Haines, Majak, Karren, Yanke and McAllister2000; Loneragan et al., Reference Loneragan, Gould, Mason, Garry, Yost, Lanza, Miles, Hoffman and Mills2001a, Reference Loneragan, Gould, Mason, Garry, Yost, Miles, Hoffman and Mills2001b; Woolums et al., Reference Woolums, Mason, Hawkins, Brown, Williams, Gould, Fox, Sturgeon, Anderson, Duggan, Sanchez, Barrett and Chitwood2004, Reference Woolums, Loneragan, Hawkins and Williams2005a, Reference Woolums, Loneragan, Hawkins and Williams2005b). The investment in animal care and feeding accrued by the time an animal dies of AIP, in contrast to deaths from BRDC which typically occur early in the feeding period, represents a significant and disproportionate economic loss.
Etiology and pathogenesis
As detailed earlier, the clinical manifestation of AIP depends on the development of DAD as a result of widespread damage to alveolar epithelial cells. While 3-MI is highly implicated in the development of AIP in pastured cattle, the pathogenesis of this disease in feedlot cattle remains less clear given the absence of lush forages in feedlot rations. Nonetheless, there is some evidence for the involvement of 3-MI in feedlot AIP; it has been reported that plasma concentrations of the pneumotoxic metabolite 3-MEIN in heifers with a clinical diagnosis of AIP were over twice that of control animals, while urinary concentrations of a secondary 3-MI metabolite – 3-MI mercapturate – were significantly lower (Ayroud et al., Reference Ayroud, Popp, VanderKop, Yost, Haines, Majak, Karren, Yanke and McAllister2000). However, the same study found no measurable 3-MI within ruminal fluid in either group, nor were there detectable differences in 3-MEIN levels in lung tissues. These results differed slightly from similar studies, which found elevated 3-MEIN levels in blood and lung tissue of both AIP-affected and bronchopneumonia-affected animals when compared to healthy animals (Loneragan et al., Reference Loneragan, Gould, Mason, Garry, Yost, Lanza, Miles, Hoffman and Mills2001a). For 3-MI to be truly implicated in AIP of feedlot cattle, further research defining the source and metabolic pathway of its production in the absence of lush forage is required. Feedlot cattle late in their feeding periods are typically fed highly fermentable, grain-based diets such as mixed rations of barley grain and barley silage (Ayroud et al., Reference Ayroud, Popp, VanderKop, Yost, Haines, Majak, Karren, Yanke and McAllister2000). Such diets are prone to induction of subclinical ruminal acidosis secondary to the accumulation of volatile fatty acids (Nagaraja and Titgemeyer, Reference Nagaraja and Titgemeyer2007). Plausibly, this may create a ruminal microenvironment that selectively permits proliferation of acid-tolerant bacteria such as Lactobacillus – a species previously discussed to be involved in the conversion of IAA into 3-MI (Yokoyama et al., Reference Yokoyama, Carlson and Holdeman1977; Yokoyama and Carlson, Reference Yokoyama and Carlson1981; Carlson and Breeze, Reference Carlson and Breeze1984; Honeyfield and Carlson, Reference Honeyfield and Carlson1990; Tannock, Reference Tannock2004). Despite this, relevant studies in feedlot cattle have found no correlation between increased levels of ruminal Lactobacillus and the risk of AIP development, which suggests other bacterial species, such as those discussed previously, may be key components in a ruminal microbiome that favours 3-MI production (Ayroud et al., Reference Ayroud, Popp, VanderKop, Yost, Haines, Majak, Karren, Yanke and McAllister2000).
AIP in feedlot cattle has several other identified risk factors. There is a strong trend for cases of feedlot AIP to occur in hot and dusty conditions and, as such, is most frequently diagnosed in spring and summer months (Jensen et al., Reference Jensen, Pierson, Braddy, Saari, Lauerman, England, Benitez, Horton and McChesney1976; Ayroud et al., Reference Ayroud, Popp, VanderKop, Yost, Haines, Majak, Karren, Yanke and McAllister2000; Woolums et al., Reference Woolums, Mason, Hawkins, Brown, Williams, Gould, Fox, Sturgeon, Anderson, Duggan, Sanchez, Barrett and Chitwood2004); however, in isolation, dusty conditions are not sufficient to cause AIP in ruminants (Purdy et al., Reference Purdy, Straus, Chirase, Parker, Ayers and Hoover2002, Reference Purdy, Straus, Chirase, Ayers and Hoover2003). Similarly, infectious agents such as bovine respiratory syncytial virus (BRSV, bovine orthopneumovirus) or bacteria may synergise with 3-MI to potentiate AIP development, but appear to be neither a sufficient nor necessary cause (Collins et al., Reference Collins, Jensen, Smith, Flack, Kerschen, Bennett, Jones and Alexander1988; Bingham et al., Reference Bingham, Morley, Wittum, Bray, West, Slemons, Ellis, Haines, Levy, Sarver, Saville and Cortese1999; Sorden et al., Reference Sorden, Kerr and Janzen2000; Loneragan et al., Reference Loneragan, Gould, Mason, Garry, Yost, Miles, Hoffman and Mills2001b; Woolums et al., Reference Woolums, Mason, Hawkins, Brown, Williams, Gould, Fox, Sturgeon, Anderson, Duggan, Sanchez, Barrett and Chitwood2004).
Epidemiology
Several factors have been linked to the development of AIP in feedlot cattle. Consistently, heifers are 1.6–3.1 times more likely to develop AIP than their male counterparts; however, there appears to be variation among feedlots in the impact of this sex predisposition, as feedlots that place high proportions of heifers are not necessarily more likely to report AIP-related mortalities (Ayroud et al., Reference Ayroud, Popp, VanderKop, Yost, Haines, Majak, Karren, Yanke and McAllister2000; Loneragan et al., Reference Loneragan, Gould, Mason, Garry, Yost, Lanza, Miles, Hoffman and Mills2001a, Reference Loneragan, Gould, Mason, Garry, Yost, Miles, Hoffman and Mills2001b; Woolums et al., Reference Woolums, Loneragan, Hawkins and Williams2005a, Reference Woolums, Loneragan, Hawkins and Williams2005b). Induction of enzymes responsible for 3-MEIN activation, such as cytochrome P450 or prostaglandin H synthase, by the estrus-suppressing feed additive melengestrol acetate (MGA) has been proposed as a cause of this sex disparity. However, while there is evidence that MGA feeding may increase the severity of experimentally induced AIP in ruminants, a repeatable association between MGA feeding and increased rates of AIP in feedlot systems is yet to be observed (Popp et al., Reference Popp, McAllister, Kastelic, Majak, Ayroud, VanderKop, Karren, Yost and Cheng1998; Ayroud et al., Reference Ayroud, Popp, VanderKop, Yost, Haines, Majak, Karren, Yanke and McAllister2000; Stanford et al., Reference Stanford, McAllister, Ayroud, Bray and Yost2006). In a comprehensive survey of management practices in US feedlots, other factors found to be associated with increased AIP prevalence included feedlot location, number of animals placed, and vaccination protocols (Woolums et al., Reference Woolums, Loneragan, Hawkins and Williams2005a, Reference Woolums, Loneragan, Hawkins and Williams2005b). Feedlots from southern compared to northern states were significantly more likely to recognize AIP-related morbidities and mortalities possibly reflecting the observation that AIP appears to be linked to seasonal weather conditions (Jensen et al., Reference Jensen, Pierson, Braddy, Saari, Lauerman, England, Benitez, Horton and McChesney1976). Feedlots that placed greater than 10,000 cattle per year were also significantly more likely to self-report AIP-related morbidities and mortalities as being a problem for their feedlot when compared to smaller feedlots (90% vs 62%). Lastly, this survey reported that a greater than 95% rate of vaccination for Mannheimia haemolytica and Pastuerella multocida was associated with lower reporting rates of AIP morbidity and mortality. Paired with microbiological studies, which reported higher rates of concurrent microbial infection in AIP-affected cattle when compared to controls, this last finding could suggest a contributing role of microbial agents in the development of AIP (Loneragan et al., Reference Loneragan, Gould, Mason, Garry, Yost, Miles, Hoffman and Mills2001b; Woolums et al., Reference Woolums, Mason, Hawkins, Brown, Williams, Gould, Fox, Sturgeon, Anderson, Duggan, Sanchez, Barrett and Chitwood2004). However, the protective effects of vaccination are potentially confounded by animal age given that, in this survey, feedlots with high rates of vaccination were more likely to be stocking younger cattle.
Concurrent infections
In feedlots, AIP-related mortalities are often diagnosed with concurrent bronchopneumonia. This has prompted an investigation into the role of infectious agents in feedlot AIP. The post-mortem lesions of a cranioventral, often chronic, bronchopneumonia coupled with a caudodorsal interstitial pneumonia are a commonly reported finding and it is estimated that 32–97% of feedlot AIP cases may have concurrent bronchopneumonia (Hjerpe, Reference Hjerpe1983; Sorden et al., Reference Sorden, Kerr and Janzen2000; Woolums et al., Reference Woolums, Mason, Hawkins, Brown, Williams, Gould, Fox, Sturgeon, Anderson, Duggan, Sanchez, Barrett and Chitwood2004; Valles et al., Reference Valles, Apley, Reinhardt, Bartle and Thomson2016). Microbiological investigations of respiratory disease in feedlot cattle have lent support to the hypothesis that agents traditionally associated with bronchopneumonia may also be involved in the development of AIP. In these studies, AIP-affected cattle yielded positive bacterial and mycoplasmal cultures at significantly higher rates when compared to healthy controls; Pasteurella multocida and Mycoplasma spp. were most common, and one or both of these organisms were isolated from up to 42% of AIP-affected cattle (Loneragan et al., Reference Loneragan, Gould, Mason, Garry, Yost, Miles, Hoffman and Mills2001b; Woolums et al., Reference Woolums, Mason, Hawkins, Brown, Williams, Gould, Fox, Sturgeon, Anderson, Duggan, Sanchez, Barrett and Chitwood2004). Conversely, Booker et al. (Reference Booker, Abutarbush, Morley, Jim, Pittman, Schunicht, Perrett, Wildman, Fenton, Guichon and Janzen2008) found no positive correlation between infectious organisms and interstitial pneumonia, although this study relied entirely on immunohistochemistry for the identification of microbial pathogens.
Further debate surrounds the role of viral agents in the pathogenesis of feedlot AIP, particularly that of BRSV. The consensus from several studies appears to be that BRSV is not primarily responsible for AIP, as most cases fail to demonstrate a consistent and statistically significant presence of the organism, particularly when compared to healthy control animals (Sorden et al., Reference Sorden, Kerr and Janzen2000; Loneragan et al., Reference Loneragan, Gould, Mason, Garry, Yost, Miles, Hoffman and Mills2001b; Woolums et al., Reference Woolums, Mason, Hawkins, Brown, Williams, Gould, Fox, Sturgeon, Anderson, Duggan, Sanchez, Barrett and Chitwood2004; Fulton et al., Reference Fulton, Blood, Panciera, Payton, Ridpath, Confer, Saliki, Burge, Welsh, Johnson and Reck2009). Furthermore, if infectious agents truly did play a significant role in the pathogenesis of feedlot AIP, one would likely expect a temporal relationship between the occurrence of AIP mortalities and the time period at which risk of infectious lung disease is at its highest (i.e. the early feeding period); this is not the case, however. That being said, uncertainties still surround the assertion that infections do not contribute to feedlot AIP, as most of these studies investigating the role of pathogens performed analyses on tissues obtained post-mortem and clearance of a virus prior to the time of death could explain negative test results. Adding further complexity to this discussion, there have been conflicting reports as to whether BRSV can act synergistically with 3-MI to potentiate its pneumotoxicity (Castleman et al., Reference Castleman, Lacy, Slauson and Atz1990; Bingham et al., Reference Bingham, Morley, Wittum, Bray, West, Slemons, Ellis, Haines, Levy, Sarver, Saville and Cortese1999); details of experimental design, such as the use of young calves and attenuated viral strains, might explain these conflicting results.
Pathology of feedlot acute interstitial pneumonia
The gross and histopathological findings of AIP have been well-characterized and, with minor variations, descriptions of AIP in pasture-fed animals from the earliest reviews of the topic highlight the same core features as those described in more recent reviews of AIP in both feedlot and pasture-based systems (Blood, Reference Blood1962; Jensen et al., Reference Jensen, Pierson, Braddy, Saari, Lauerman, England, Benitez, Horton and McChesney1976; Curtis et al., Reference Curtis, Thomson and Sandals1979; Sorden et al., Reference Sorden, Kerr and Janzen2000; Doster, Reference Doster2010; Panciera and Confer, Reference Panciera and Confer2010; Woolums, Reference Woolums2015).
Upon post-mortem examination, the lungs of cattle dying from AIP fail to collapse when the chest is opened, and thus are markedly expanded and occupy much of the thoracic cavity (Fig. 1a). Lobules of palpably firm and rubbery lung tissue are often separated by marked emphysema and edema of interlobular tissue (Fig. 1b). Varying degrees of congestion and hyperinflation between neighboring lobules can create abrupt color and texture variations, colloquially termed a ‘checker-board pattern’, that ranges from grey-brown to pale pink through to dark red or purple. The entirety of the lungs are often affected, although gross lesions are usually most conspicuous in the caudodorsal lung. Emphysema of the mediastinum and dorsal subcutaneous tissue may also be occasionally observed. While the above description holds true for most cases of diffuse AIP, alternative descriptions of interstitial pneumonias with varying distributions have also been offered. Lesions of interstitial pneumonia in the caudodorsal lung occurring concurrently with a cranioventral bronchopneumonia have been described (Curtis et al., Reference Curtis, Thomson and Sandals1979; Sorden et al., Reference Sorden, Kerr and Janzen2000).

Fig. 1. Acute interstitial pneumonia in a feedlot steer. (a) Lungs are poorly collapsible and occupy most of the thoracic cavity. Individual lobules and regions of the lung vary in their degree of congestion (dark red) or hyperinflation (pale pink). The lung parenchyma bulges slightly when incised. There is a small focus of pleural fibrous adhesion in the cranioventral lung, presumably the result of a resolved episode of focal pleuritis. (b) There is marked edema and emphysema expanding the interlobular septa and connective tissue around large arteries. White foamy fluid exudes from the parenchyma and airways.
The histological features of interstitial pneumonia progress typically through the three phases of acute ILD discussed earlier in this review; these lesions are well described in the literature (Jensen et al., Reference Jensen, Pierson, Braddy, Saari, Lauerman, England, Benitez, Horton and McChesney1976; Collins et al., Reference Collins, Jensen, Smith, Flack, Kerschen, Bennett, Jones and Alexander1988; Woolums et al., Reference Woolums, Loneragan, Gould and McAllister2001; Caswell and Williams, Reference Caswell, Williams and Maxie2016). The acute exudative phase is characterized by extensive intra-alveolar accumulations of deeply eosinophilic, wispy to dense and fibrillar material – consistent with fibrin – and deeply eosinophilic hyaline membranes that expand and outline alveolar margins (Fig. 2a). The composition of hyaline membranes is heterogenous and may consist of variable amalgams of plasma proteins, surfactant proteins, polymerizing fibrin, and components of necrotic cells that have accumulated secondarily to diffuse pneumocyte injury (Sun et al., Reference Sun, Ohtsuki, Fujita, Ishida, Yoshinouchi and Kohno2003; Peres e Serra et al., Reference Peres e Serra, Parra, Eher and Capelozzi2006); the predominant composition of these membranes in cases of feedlot AIP has not been investigated. While alveolar edema, fibrin exudation and hyaline membranes are the hallmark features of acute ILD, lesions such as interlobular emphysema, and intra-alveolar and interstitial hemorrhage and edema may also be frequently observed (Woolums et al., Reference Woolums, Loneragan, Gould and McAllister2001). Additionally, necrosis of the bronchiolar epithelium is prominent in many natural cases of feedlot AIP, despite this feature often being absent in natural cases in pastured cattle (Ayroud et al., Reference Ayroud, Popp, VanderKop, Yost, Haines, Majak, Karren, Yanke and McAllister2000). Expansion of alveolar septa by edema and variable numbers of neutrophils and/or eosinophils is reported in natural cases of pasture-associated AIP (Caswell and Williams, Reference Caswell, Williams and Maxie2016). In cases in which a concurrent bronchopneumonia is diagnosed, the lesions in this component of the lung may be either acute or chronic in nature. Bronchiolitis obliterans is a prominent feature in animals suffering concurrently from both interstitial pneumonia and bronchopneumonia, reflecting chronic damage to airways. Indeed, it has been found that animals with AIP in a feedlot setting are more likely to have histologically appreciable bronchiolitis obliterans compared to pen mates with no history of lung disease (Woolums et al., Reference Woolums, Mason, Hawkins, Brown, Williams, Gould, Fox, Sturgeon, Anderson, Duggan, Sanchez, Barrett and Chitwood2004). Animals that survive the acute exudative phase may develop a subacute proliferative phase in which cuboidal type II pneumocytes proliferate to replace injured alveolar epithelium (Fig. 2b). Finally, a chronic stage results in the eventual re-epithelialization of alveoli by type II pneumocytes and progressive fibrosis of the lung interstitium (Fig. 2c). The net result of this phase of the disease is the fibrous expansion of lung interstitium with subsequent reduced lung compliance and impedance of gas exchange.

Fig. 2. (a) Acute exudative phase of interstitial pneumonia in a feedlot steer. Hyaline membranes in the form of dense eosinophilic tissue covering the surface of alveoli and alveolar ducts. Wispy proteinaceous fluid and scant hemorrhage fills alveoli. Hematoxylin & eosin. Original objective 20x. (b) Subacute proliferative phase of interstitial pneumonia in a feedlot heifer. Alveoli are segmentally to circumferentially lined by cuboidal type II pneumocytes. Alveoli are filled with fibrin, increased numbers of macrophages, scattered cell debris, and proteinaceous fluid. Hematoxylin & eosin. Original objective 20x. (c) Chronic fibrosing phase of interstitial pneumonia in a feedlot heifer. The alveolar septa are markedly thickened by fibrous tissue, plump interstitial fibroblasts, and scattered mononuclear inflammatory cells. Alveoli are circumferentially lined by type II pneumocytes. Viable and degenerate neutrophils and fewer macrophages fill alveoli. Hematoxylin and eosin. Original objective 20x.
Conclusions
AIP in feedlot cattle remains an enigmatic condition with a poorly defined etiopathogenesis. While there have been significant efforts to equate this condition in feedlot populations to that seen in pasture-fed cattle, evidence of a similar cause and pathogenesis remains to be established. Given the established role of 3-MI in pasture-associated AIP, much focus has been dedicated to determining the role of 3-MI in feedlot AIP mortalities. However, based on the current body of literature, the relation of 3-MI to feedlot AIP is comparatively more nebulous. While there is certainly evidence to suggest a role of this pneumotoxicant in at least some cases of feedlot AIP, the specific factors that contribute to this condition are far more imprecisely defined, and likely more numerous, than those contributing to the pasture-associated disease. If mitigation of the animal welfare and economic impacts of AIP on feedlot systems is ever to be achieved, further research into the contribution of factors such as diet and dietary supplements, sex, infectious agents, concurrent disease (e.g. bronchopneumonia), animal genetics, seasonal factors, management factors, and preventive measures will be required.
Acknowledgements
We thank Feedlot Health Management Services in Okotoks, Alberta for their considerable expertise and assistance in the conduction of this review. In particular, we acknowledge the hard work and diligence of Dani Smerek.
Financial support
This work was supported by the Beef Cattle Research Council of the Canadian Cattleman's Association (ANH 13.17), and the Natural Sciences and Engineering Research Council of Canada (NSERC; RGPIN-2017-03872; RGPAS-2017-507803).