1 Introduction
As climate change increases the likelihood of competition for water among agricultural, municipal, and environmental users, the need to confront growing risks related to water quality, quantity, and an increasingly fragile infrastructure becomes more urgent (Reference FamigliettiFamiglietti, 2014; Reference GleickGleick, 1993; Reference Kundzewicz, Mata, Arnell, Doll, Jimenez and MillerKundzewicz et al., 2008; Reference Melillo, Richmond and YohoMelillo, 2014; U.S. Global Change Program, 2014). Adapting to these risks requires political will and public support for strategies such as decreasing water use, accepting recycled water (converting wastewater into water that can be used for other purposes), and paying for infrastructure improvements. In this study, we investigate how university students think about the entire system of water supply, delivery, and treatment in order to uncover any prevailing misperceptions about how this vital and complex system functions. Understanding how these young adults coarsely interpret the complex reality of the water system is an important step for environmental education, and can help identify ways to protect our water system without overloading cognition.
Although functional and effective water systems are taken for granted (Reference LundLund, 2015), many recent events have placed water quality, quantity, and infrastructure at risk. For example, in January 2014, an industrial solvent spilled into West Virginia’s Elk river and contaminated 15% of the state’s potable water, causing a “do not use” order on residential water for ten days (Reference Whelton, McMillan, Connell, Kelley, Gill and WhiteWhelton et al., 2015). Related to quantity, California’s Governor Brown issued an emergency executive order on April 2015 mandating a 25% statewide reduction in urban potable water use to address diminishing water supplies caused by the ongoing drought (Reference BrownBrown, 2015). The American Society of Civil Engineers gave a D+ grade to the U.S. for the state of its infrastructure (ASCE, 2017), and the U.S. Environmental Protection Agency (EPA) estimates that approximately $130 million is needed to maintain existing water infrastructure (EPA, 2003; Reference Tanellari, Bosch, Boyle and MykereziTanellari, Bosch, Boyle & Mykerezi, 2015). Repairing the aging water infrastructure should be, according to the EPA, “one of our Nation’s top water priorities” (EPA, 2012a).
To address these increased risks, different approaches to water management are being considered, such as reusing wastewater to augment freshwater sources (Reference Po, Nancarrow and KaercherPo, Nancarrow & Kaercher, 2003; Reference Rozin, Haddad, Nemeroff and SlovicRozin, Haddad, Nemeroff & Slovic, 2015; Reference Vörösmarty, McIntyre, Gessner, Dudgeon, Prusevich and GreenVörösmarty et al., 2010; Reference Wester, Timpano, Lieberman, Fieldstone and BroadWester et al., 2015). Public perception is a significant barrier to implementing wastewater reuse for potable water on a municipal level. Some potential users report feelings of disgust, i.e. the “yuck factor,” associated with what is referred to as “toilet to tap” water (Reference Rozin, Haddad, Nemeroff and SlovicRozin et al., 2015). These users likely do not understand that communities with surface water sources are already participating in this cycle: upstream communities discharge their treated wastewater into nearby creeks, rivers, and lakes, which then become part of the water supply for downstream communities (EPA, 2012b; Reference HartleyHartley, 2006). Another potential strategy for addressing shortages is changing water prices to reflect water scarcity, which can effectively decrease urban water use (Reference Olmstead and StavinsOlmstead & Stavins, 2009), but public acceptance for such policies is also lacking.
To understand how residential water users think about water delivery, we take an approach that utilizes mental models and systems thinking. While some research communities use these terms to explain cognitive processes, and others apply them to the understanding of system dynamics (Reference ForresterForrester, 1961; Reference Meadows and WrightMeadows & Wright, 2008; Reference Sterman and SweeneySterman & Sweeney, 2002), we use mental models and systems thinking to represent participant understanding of how a system works.
A large body of research uses structured interviews to elicit mental models from experts and novices to see where there are gaps in understanding (Reference Morgan, Fischhoff, Bostrom and AtmanMorgan, Fischhoff, Bostrom & Atman, 2002), and these methods have been used in a variety of environmental domains. For example, Reference Bostrom, Morgan, Fischhoff and ReadBostrom, Morgan, Fischhoff & Read (1994) and Read et al. (1994) highlighted that participants in their initial studies of mental models of climate change tended to confuse stratospheric ozone depletion with the greenhouse effect and weather with climate. Their follow-up work found that some of the initial misperceptions were corrected over time, such as participants not mentioning ozone depletion as a direct cause of climate change (Reference Reynolds, Bostrom, Read and MorganReynolds, Bostrom, Read & Morgan, 2010).
Systems thinking research has also been used to investigate resource conservation and understanding. Kempton (1986) investigated mental models of how thermostats work, and found that 25-50% of Americans are “valve theorists” (thermostat controls the amount of heat) rather than the more accurate “feedback theorists” (thermostat senses the temperature and turns the furnace on or off to maintain an even temperature). This inaccuracy can lead to significant increases in energy use, as valve theorists are inclined to use a higher heat setting to heat up a house faster, an inefficient practice. The work of Gentner and Gentner (1982), tested how using different analogical models for electrical circuits (as flowing fluids or crowds of moving objects) could improve participant performance on solving electricity problems. Thus, identifying lay mental models can reveal surprising misperceptions that have implications for evidence-based policies.
How important is systems thinking for environmental education? Do citizens need to understand the basic structure, function, and interconnections in resource systems to make informed decisions at the individual and societal level? Orion (2002) argues that a scientific understanding of how these system work and are interconnected is a necessary foundation for a citizen’s ability to contribute to environmental preservation. Resource conservation decisions can range from adopting effective conserving behaviors in the home (Reference Inskeep and AttariInskeep & Attari, 2014) to accepting alternative price structures for resources (Reference Olmstead, Hanemann and StavinsOlmstead, Hanemann & Stavins, 2007) to altering voting behavior related to environmental management. If citizens believe that water magically comes out of the tap and electricity from a wall socket – without understanding, at least in some sense, the integrated system behind the tap and wall – then, if parts of that unseen but expected system weaken, reacting and adapting to those weaknesses could be slow and potentially catastrophic. A recent example is the lead contamination water crisis in Flint, Michigan, where introducing a more corrosive water source into an aging water system without corrosion control led to elevated blood lead levels in children (Reference Hanna-Attisha, LaChance, Sadler and Champney SchneppHanna-Attisha, LaChance, Sadler & Champney Schnepp, 2016). Thus, without some application of systems thinking to vital resources, identifying and responding to system-level problems will be a formidable challenge.
In our study we elicit water system drawings from expert and university student participants. We use drawings rather than other means (such as open-ended structured interviews or closed-ended surveys) to gain a view into participants’ mental constructs. This use of participant drawings to gauge system thinking has been previously applied to environmental questions. Focusing on system dynamics, Sterman (2008) asked university student participants to sketch out the emissions trajectory that would be required to stabilize carbon dioxide concentration. He showed that 84% of his student participants drew patterns of emissions that violated the principles of accumulation; if emissions followed the modal path drawn by these students, concentrations would continue to rise rather than stabilize. Sterman (2008) went on to argue that the general public’s intuitive understanding of systems dynamics (including stocks and flows) is poor, but improving this understanding is vital for effective action on important issues such as stabilizing atmospheric carbon dioxide concentration.
Closely related to the research endeavor at hand, elicited drawings have also been used to examine how students understand the water cycle (Reference Assaraf, Eshach, Orion and AlamourAssaraf, Eshach, Orion & Alamour, 2012; Reference Assaraf and OrionAssaraf & Orion, 2005; Reference BarBar, 1989; Reference Dove, Everett and PreeceDove, Everett & Preece, 1999; Reference Shepardson, Wee, Priddy, Schellenberger and HarborShepardson, Wee, Priddy, Schellenberger & Harbor, 2007a). Reference Assaraf and OrionAssaraf and Orion (2005) asked high school juniors to draw the water cycle, finding that many students focus exclusively on the atmospheric component of the cycle (evaporation, condensation, and rainfall) while ignoring the groundwater component.
Reasons why people fail to accurately understand natural and anthropogenic systems may include lack of everyday experience with separate system components in addition to limited comprehension of the complex structural, functional, and behavioral elements that interact to form these systems (Reference Hmelo-Silver, Marathe and LiuHmelo-Silver, Marathe & Liu, 2007). For example, people rarely see the underground water pipeline infrastructure for drinking water and wastewater treatment plants. Even students who take courses related to water systems may have difficulty comprehending the full set of processes, as systems thinking is rarely reflected in water-related higher education textbooks. The water system is frequently presented in these textbooks as disaggregated processes (e.g., water treatment or wastewater treatment processes) rather than interrelated and integrated parts of a functioning whole (J. E. Drinan & F. Spellman, 2012; EPA, 1991; Reference NationalNational Research Council, 2006); see SI Text for detailed examples). In K-12 education, water tends to appear in science classes idiosyncratically (Reference Gunckel, Covitt, Salinas and AndersonGunckel, Covitt, Salinas, & Anderson, 2012; Sadler, Nguyen & Lankford, 2016), with some focus on the water cycle, but little attention to the built water system.
Here, we move beyond investigating understanding of the water cycle and focus instead on student understanding of the system of interconnected processes required to deliver potable water to the home and manage that water once it leaves the home. By eliciting drawings, we gain the advantage of allowing participants to reflect on their mental models and represent them non-verbally. Drawings also enable the presentation of concepts independent from scientific terminology, allowing participants to have more confidence in their answers (Reference Fischer and YoungFischer & Young, 2007).
1.1 Research questions
Our research explores the following questions:
-
1 How do university students’ mental models of the water system match those of water experts? Where along the system does student understanding fail?
-
2 Do environmental students have a more accurate understanding of the water system?
-
3 Which risks are most salient to students – those related to water quality, quantity, or infrastructure?
Here we investigate these research questions by (a) constructing a diagram of the drinking water system that reflects the consensus of expert water scientists, (b) using the consensus expert diagram to code students drawings of the entire drinking water system, and (c) identifying factors that predict student accuracy in understanding the drinking water system, including perceptions of water-related risks.
Understanding and identifying the prevailing misperceptions related to water systems thinking could lead to improved educational tools and increased public support for adaptation to risks related to water quality, quantity, and infrastructure. This information may also guide acceptance of technologies, such as recycled water, and more effective and efficient water pricing mechanisms to increase adaptive capacity.
2 Methods
2.1 Participants
Expert Participants: Between September and December of 2014, Indiana water experts (N = 15) were interviewed in person for an expert elicitation. Experts included professors at Indiana University and Purdue University as well as professionals who run public water utilities in the state. Our participants had expertise in the following areas: environmental engineering, water supply and wastewater treatment, nutrient transport in water, environmental toxicology, water quality, watershed hydrology, groundwater modeling, water management, politics of water development, and natural resource science.
Student Participants: In April 2014, students participated in an in-class paper survey administered at Indiana University Bloomington (N = 578). Email requests to conduct the survey at the beginning of class were sent to more than 15 professors at the University, chosen based on environmental versus non-environmental course offerings, and in some cases, chosen based on whether the authors knew the professors to make this request. The courses taught by the professors ranged from environmental science to performing arts. The survey was then conducted in 19 separate classes. The surveys were distributed during class within a two-week period. Although survey responses were collected from 578 participants, only 457 participants completed the first question (instructing them to draw a water system), thus we restrict our analysis to this subsample. Students may have chosen not to complete the survey because it was voluntary and uncompensated. For this study sample (N = 457), the median age of participants was 21 years old, 50.1% were female, and 9.7% were international students. The median level of education was a third-year undergraduate (junior) in college. Approximately 84.6% of the participants were at the undergraduate level, 14.5% were at the master’s level, and 1.1% were at the doctoral level. Given that this is a sample of convenience, our figures indicate some selection bias, although the demographic characteristics are somewhat representative of the students at the university: 10.7% masters, 9.7% doctoral, and 10% international (Indiana University School of Education, 2014; University Institutional Research and Reporting, 2013).
2.2 Expert elicitation
We examined many environmental engineering and water textbooks to search for an accurate systems drawing by which to score the student drawings (see this supplement). None of the drawings we found were adequate for our project because textbooks tend to focus on component processes (such as the specific processes involved in wastewater treatment) rather than how all the natural and human processes fit together in an integrated system (Reference Drinan and SpellmanDrinan & Spellman, 2012). We therefore created our own drinking water system diagram by interviewing 15 Indiana water experts. Experts were visited in their offices or were requested to visit our lab one at a time. Each expert was given the following instructions verbally:
Please draw a diagram illustrating your understanding of the processes by which clean water reaches the tap in the average home in the United States. Please draw how water reaches the home from its original sources and is then returned to the natural environment.
Diagrams were drawn on either on a piece of paper or on a white-erase board.Footnote 1 The experts were then asked to explain how their system diagrams worked and to justify why each process was included. After the first expert elicitation, we produced a working version of the simplified diagram. Each of the subsequently interviewed experts were asked to critique the simplified expert diagram at the end of their elicitation, resulting in some changes in the level of detail in the simplified diagram. After all the expert elicitations were complete, we produced a final version of the simplified diagram, then re-visited two of the experts interviewed earlier to verify the processes included in the simplified diagram.
2.3 Student survey
The first question in the paper survey prompted participants to draw a diagram of a typical U.S. drinking water system:
Please draw a diagram illustrating your understanding of the processes by which clean water reaches the tap in the average home in the United States. Please draw how water reaches the home from its original source(s) and is then returned to the natural environment. Show all of the processes that the water goes through. You are encouraged to label your drawing and add any explanations you believe will help convey your understanding of the water system.
The rest of the page was blank, allowing participants space to draw their diagrams.
Following the diagram elicitation, participants were asked if they believed there are risks associated with drinking water quality. If participants answered yes, they were asked to identify the greatest risk they perceived to be associated with drinking water quality. The next two questions followed this same format, focusing on perceived risks associated with water quantity and water infrastructure. Participants were also asked to indicate how adequate they found drinking water in terms of quality, quantity, and infrastructure using a 5-point rating scale, ranging from highly inadequate to highly adequate.
Participants were then asked whether the water they received from their tap was generally safe or likely to contain harmful contaminants. If participants identified their water as likely to contain contaminants, they were asked to list any contaminants that came to mind. Participants were then asked to identify the frequency with which they thought about home water use using a 5-point rating scale: never, rarely, occasionally, a moderate amount, and a great deal. Participants were asked to list which activities made them most aware of the quantity of water used in the home.
Participants were asked to characterize whether they would describe their local water supply as safe to drink using a 5-point scale: far below average, below average, average, above average, and far above average. Participants were then asked to list the factors that caused them to think about water quality in their home.
The next set of questions assessed the information available to the participants with regard to their local water supply. Participants were asked how difficult it was for them to find out information about their local water supply system, then asked to indicate whether they obtain system information from the following sources: public utilities, news articles, personal observation, class, word of mouth, water bill, none, or other. Participants were also asked to indicate whether they had observed the following system parts in the past year: water main, storage tank, surface runoff, drinking water treatment, surface water, reservoir, pump station, wastewater treatment, water meter, or none.
Finally, participants were asked to identify their local drinking water source. Demographic questions concluded the survey. The entire survey is presented in a supplement.
3 Results
3.1 Expert elicitation and accurate systems diagram
Experts were asked to draw their representations of the entire drinking water system. Experts varied in their interpretations of these instructions. Some experts focused on specific details of water treatment and wastewater treatment, while others focused on how their expertise related to the water system. Figure 1 shows an example of one of the expert drawings, chosen for legibility and clarity in including details of both natural and human processes.

Figure 1: An expert elicited drawing of an average U.S. drinking water system showing an integration of the natural and human system.
Although there were differences in how the experts interpreted the question, the expert drawings are broadly similar and converged, leading to the consensus diagram shown in Figure 2. One coder coded each of the 12 expert drawings that we photographed. Ten experts illustrated at least seven common major components of drinking water systems: water source, drinking treatment, distribution, household use, collection, wastewater treatment, and return to environment. One expert drew only six major components, missing “return to environment,” and another drew a diagram that focused exclusively on that expert’s specialization, without drawing the larger water system. Thus the consensus diagram comprises seven major categories of processes involved in getting water from the environment to the average household in the U.S. and back to the environment. A second supplement shows three additional expert drawings.

Figure 2: Simplified drinking water system diagram created after expert elicitation. The seven major categories for analysis are listed on the top of the diagram. (Note that leaks occur throughout the water system, but are shown only in one place on our simplified system diagram.)
Figure 2 shows that drinking water sources are divided into surface water (such as lakes and rivers) and ground water. Drinking water can be publically supplied by utilities, as is the case for 85% of households in the U.S., or privately supplied via onsite household treatment of groundwater, as is the case for 15% of households in the U.S. (U.S. Geological Survey, 2014). In the public system, water is pumped from surface water, ground water, or a combination of the two, and then sent to a drinking water treatment plant where it undergoes treatment. Public water systems use a variety of methods to treat water; the most common steps include coagulation and flocculation (which enhances the ability of the treatment process to remove particles), sedimentation (allows particles to settle), filtration (removes the particles), and disinfection (kills any remaining germs).
Treated drinking water then leaves the treatment plant to enter the city distribution network. Once the water reaches the household, it is used in indoor and outdoor applications. In the U.S., each person uses about 80–100 gallons of water per day across the two categories of indoor and outdoor use (USGS, 2015). In a non-representative sample of 737 homes in the U.S., DeOreo, Mayer, Dziegielewski & Kiefer (2016) found that average indoor water use was 58.6 gallons per capita per day (gpcd). The authors disaggregated total indoor water use via flow meters, finding the major water use applications to be toilets (14.2 gpcd), showers (11.1 gpcd), faucets (11.1 gpcd), clothes washers (9.6 gpcd), and leaks (7.9 gpcd). The remaining water is used outdoors, primarily for irrigation, and then returns, untreated, to the natural environment (Reference StephensStephens, 2010). Water that has been used indoors is sent to a collection system before it is treated. In a public system, this treatment can be achieved via a combined sewer system or a separate sanitary system. In a combined sewer system, wastewater and storm water are collected in the same pipes (Reference Drinan and SpellmanDrinan & Spellman, 2012). Public systems send collected wastewater to a wastewater treatment plant, where it usually undergoes preliminary treatment (removal of large solids and trash), primary treatment (separates out solids and grease from the liquid), secondary treatment (biological treatment of wastewater to break down organic material), and disinfection treatment (typically adding chlorine to kill any remaining harmful microorganisms). The treated water is then discharged back into the natural environment.
3.2 Analysis of student drawings
Although there are many possible approaches to analyzing the drawings produced by the student participants, we restricted our main analysis to whether or not the drawings included each of the seven major categories identified by the experts (listed across the top of Figure 2). Two separate coders coded each participant drawing. The coders assigned a 1 or 0 score for whether or not each of seven major categories identified as vital in the expert elicitation consensus model (Figure 2) were included in each of the student diagrams. After coding a set of diagrams, the two coders compared scores to settle on an agreed-upon final score. When coding the first subset of diagrams, the coders noticed a series of systematic misperceptions, specifically relating to not including a water treatment plant or wastewater treatment plant, including filtration as the only form of treatment, and wastewater being returned untreated to the natural environment. Separate codes for these specific misperceptions were created for analysis. Figure 3 shows three examples of student drawings that cover a range of degrees of understanding of the built water system.

Figure 3: Panels A, B, and C show examples of student drawings ranging from near perfect sophistication and understanding (panel A) to a less complete understanding (panel C). Panel A shows a drawing that scored a 6 out of 7 for inclusion of water system components (water source, drinking treatment, distribution, household use, collection system, wastewater treatment). The drawing in Panel B scored a 4 (featured components are water source, household use, collection system, wastewater treatment). Finally, panel C scored a 3 as it only includes water source, distribution, and household use. (Images were edited for ease of viewing without altering any content.)
Overall, the drawings revealed mental models that ranged from nearly accurate to a complete lack of understanding of how the system works. A perfect score of 7 (showing all categories) was only obtained by 7.2% of participants in our sample. On average, students identified approximately four categories in their diagrams out of seven categories (mean number of categories = 4.18, SE = 0.07) – see Table 1.
Table 1. Descriptive statistics for entire sample.
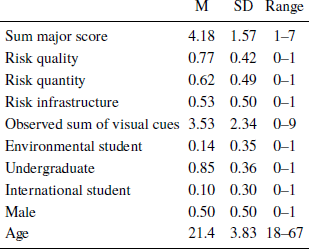
Students were categorized into “environmental” if their degree required natural resource management, environmental, or water-related courses and “non-environmental” otherwise. Based on our classification, 14% (N = 65) were environmental students and 86% (N = 392) of our sample was non-environmental. On average, environmental students identified significantly more major categories out of seven (M = 5.32, SE = 0.17) than non-environmental students (M = 3.99, SE = 0.08). The difference in the sum of major categories for the two groups differed significantly (pooled t-test t(455)= 6.65, p <0.0001).
Figure 4 shows the percentage of students that included each major category by environmental and non-environmental groups. Pairwise t-tests revealed significant differences between environmental and non-environmental students for all categories shown in Figure 4 except household use, Ps <0.05.
Figure 4 shows that for many students, the drinking water system stops at the home (with low inclusion of collection system, wastewater treatment, and return to the environment). We grouped the first three categories together (source, drinking treatment, and distribution) to create “before home” (M = 2.25, SE = 0.04) and compared to the last three categories (collection system, wastewater treatment, and return to environment) “after home” (M = 1.00, SE = 0.05). Before home and after home variables showed significant differences between environmental and non-environmental students (Ps <0.001). We note that this finding may indicate that students read the first sentence of the drawing instructions (“Please draw a diagram illustrating your understanding of the processes by which clean water reaches the tap in the average home in the United States.”) and completed their illustration without reading the second sentence of the instructions (“…and is then returned to the natural environment”).
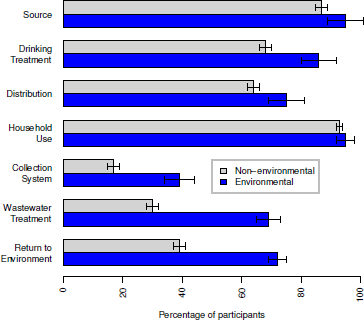
Figure 4: Percentage of students to include each major category in their water system diagram, separated by environmental (N = 65) and non-environmental (N = 392) students. Error bars correspond to 1 standard error of the mean in each direction.
Other faulty perceptions include 29% of the participants not including a water treatment plant, 19.7% of participants indicating filtration as the only form of water treatment before the water reached the home, and 64% not including a wastewater treatment plant. Approximately 1 in 5 participants (21%) depicted untreated wastewater returning to the environment.
3.3 Correlates of accuracy
To investigate which factors correlate with including more components in the system diagram, we created a “sum major score” variable, which is equal to the number of system components (out of a maximum of seven) depicted in each student-drawn diagram. Table 1 provides descriptive statistics of variables of interest, including sum major score.
As shown in Table 1, the variables “risk quality,” “risk quantity,” and “risk infrastructure” are binary, indicating whether or not the participant perceived risks associated with these categories. The variable “observed sum of visual cues” is an aggregate score that indicates how many of the following nine components participants had seen in the past year: water main, storage tank, surface runoff, drinking water treatment, surface water, reservoir, pump station, wastewater treatment, and water meter. We also constructed binary variables indicating undergraduate status (otherwise masters-level or doctoral students), international student status, and gender, along with a continuous variable for age.
Table 2 shows a correlation matrix for all the variables presented in Table 1. Perceived risk quality, risk quantity, risk infrastructure, observed sum of visual cues, environmental, graduate, and older students all correlated positively to the sum major score.
Table 2. Correlation matrix to show individual relationships.
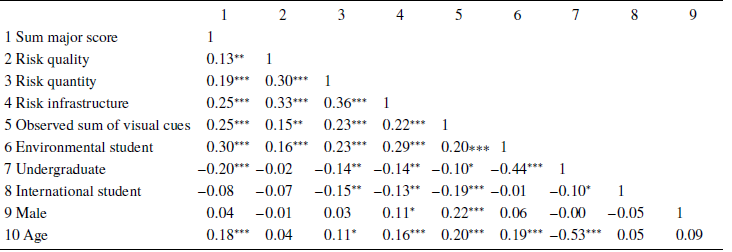
* p<.05;
** p<.01;
*** p<.001.
3.4 Risk perceptions
3.4.1 Closed-ended responses related to risk
As shown in Figure 4 and Table 2, “environmental student” is significantly correlated to students including more components in their drinking water system diagrams. To investigate environmental students further, we analyzed how having an environmental education relates to risk perceptions. Figure 5 shows percentage of participants who believe there are risks related to water quality, quantity, and infrastructure for environmental and non-environmental students. Environmental students have higher perceptions of risk for quantity, quality, and infrastructure in comparison to non-environmental majors. Mean scores of water quality risk are highest among risk categories for both groups of students, suggesting that drinking water quality is the top area of concern for students. Non-environmental students’ mean scores for risk related to quantity and infrastructure drop-off significantly compared to those of environmental students, suggesting non-environmental students are less concerned about these categories of risk.
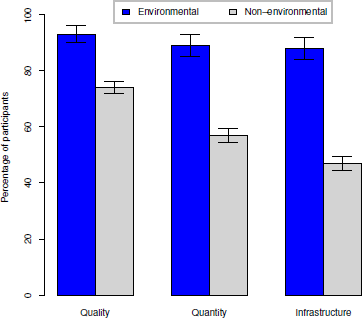
Figure 5: Percentage of participants believing that there are risks associated with water quality, water quantity, and water infrastructure, categorized by environmental and non-environmental majors. Error bars correspond to 1 standard error of the mean in each direction.
Additionally, a series of closed-ended questions asked participants to identify and rate a variety of attributes related to their water supply. The first question asked participants how often they think about the quantity of water they use in their home. About 33% of participants stated that they never or rarely (annually) think about water quantity used in their home, compared to 37% who report they think of water quantity a moderate amount (weekly) or a great deal (daily).
Participants were also asked to what extent they would describe their local water supply as safe to drink. An overwhelming majority of participants (92%) chose average, above average, or far above average in describing their local water supply as safe to drink (Lake Monroe in Bloomington, IN).
Finally, participants were asked how they would rate the level of difficulty they encounter in finding information about where their water comes from. The majority of participants (58%) perceive information on their local water source to be very difficult, difficult, or moderately difficult to find.
3.4.2 Open-ended responses related to risk
The first set of open-ended questions asked participants to identify the greatest risk associated with water quality, quantity, and/or infrastructure, if they perceived risks to exist in any of these areas. Two coders developed a coding scheme for the open-ended questions, with between 11 and 20 categories for each question. The two independent coders coded a maximum of three responses for each open-ended question (three “greatest risk” for each of quality, quantity, and infrastructure). Here we restrict our analysis to the first “greatest risk” mentioned by the participant. Assessment of inter-rater reliability suggests a high level of agreement between the two raters, with κ ranging between 0.81 and 0.88 across the three open-ended questions for the first risk coded (Reference Landis and KochLandis & Koch, 1977).
Results for the top five open-ended responses for participants who believed there were risks associated with quality, quantity, and infrastructure mentioned in their open-ended response are shown in Table 3. Close to 30% of the participants believe water quality risks to be associated with cleanliness (this category included responses using words such as cleanliness, purity, contamination, and pollution). Synthetic chemicals (15%) was the second most common risk identified and included words such as pesticides, fertilizers, and Atrazine (a herbicide commonly used in the Midwest; EPA, 2013). More than half of the participants associated drinking water quantity risks with limited supply/inadequate storage (38%) and inefficient use (27%). Water infrastructure received the lowest number of “yes” responses indicating perceived risk among participants (Figure 5). Of the participants who perceived a risk associated with drinking water infrastructure, the top response was inadequate treatment and contamination, followed by age of infrastructure. These results imply that participants believe that the risks associated with water infrastructure overlap with those for quality and quantity, which is also evident in the mention of infrastructure issues as risks to water quality and inadequate storage as a risk to quantity. Worthy of note is that the risks listed below in Table 3 are mostly causes, with very few effects of the risks mentioned.
Table 3. The top five open-ended responses for risks related to water quality, quantity and infrastructure, shown as percentages of total responses.
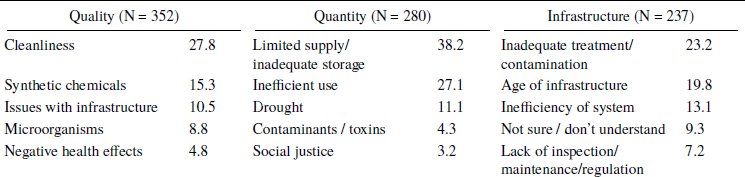
About 34% of participants agreed with the statement “water I get from my tap is likely to contain some harmful contaminants.” Participants who agreed with the statement were asked to list any contaminants that came to mind. The top three responses were heavy metals including arsenic, zinc, copper, lead, iron, and mercury (16%); not sure / I don’t know (14%); and contaminants associated with treatment including chlorine, chloramines, and bleach (10%).Footnote 2
Finally, participants were asked to identify their local water source, where the correct answer is a reservoir called Lake Monroe. Of those who answered the question and did not leave it blank (N = 412), only 18.8% of the participants listed this source correctly. Approximately 1 in 5 (19.9%) of the participants stated they did not know their local water source, and 13.6% stated that their local water source was their house (sink, fridge, and tap), which may reflect an alternative reading of the question.
3.5 Alternative wording
After analysis of the first study was complete, we wanted to understand how the details in our drawing elicitation guided our participant drawings. Specifically, “how water reaches the home from its original source(s) and is then returned to the natural environment” may have caused student participants to think of and include specific features of the water system in their drawings that they would not have originally included without such detailed wording. Thus, to test the effect of the original wording used for the drawing elicitation, we simplified the wording and elicited responses from another group of primarily non-environmental students (N = 51). The wording in this version of the survey was as follows:
Please draw all processes by which drinking water reaches the average home in the United States and all processes after it is used. You are encouraged to label your drawing.
Restricting our main original sample to undergraduates (N = 387), the mean sum of major categories included in diagrams drawn in response to the original question was 4.05 versus 2.94 in this alternative wording group. In response to the reworded question, we find that many more participants drew bottled water as their main water source (14% in this sample compared to 2.3% in our total undergraduate main sample). These results imply that the level of detail specified in the main study was necessary to effectively guide participants to draw the drinking water system.
4 Discussion
Drinking water is the most essential among all resources, and yet water is often taken for granted in highly developed contexts. In the U.S., most people expect to have immediate access to safe drinking water, which appears from the tap as if by “magic”. Hidden from view, however, are the multiple parts of this complex and vital system that must work seamlessly together for drinking water to be made readily available. Given the present risks related to water quality, quantity, and infrastructure, informed decisions concerning drinking water and the policies and management structures that support it are critically important.
Our results show that most university students are unable to produce a complete or accurate depiction of the built water system. Environmental students are more likely than others to include a higher number of the seven major categories of the drinking water system in their drawings (i.e., water source, drinking treatment, distribution, household use, collection, wastewater treatment, and return to environment). In general, students who have higher perceptions of risk related to infrastructure and those that have noticed more visual cues related to the drinking water system have more complete knowledge about the water system. However, only 7.2% of participants had a perfect score of seven out of seven water system categories, 45% of which were environmental students, and 5.5% of participants only included one of the seven categories in their diagram, all of who were non-environmental students.
Our results also highlight many significant misperceptions. We find that 19.7% of our participants indicated filtration as the only form of water treatment before the water reached the home. Additionally, 29% of the participants did not draw a water treatment plant and 64% did not draw a wastewater treatment plant. One in five participants had untreated water returning to the natural environment. Perhaps some students would have benefitted from a follow-up question asking, “What do you think happens after the home?” to distinguish between participants’ lack of knowledge and their lack of response.
In relation to risk, a high percentage of environmental student participants believed that there are risks related to water quality, quantity, and infrastructure. Non-environmental participants had a significantly lower perception of risk than environmental participants. Non-environmental participants had the highest perception of risk for water quality, followed by water quantity, and lastly for water infrastructure. This finding may shed light on why the public is seemingly uninterested in water infrastructure issues.
4.1 Implications
Water systems thinking has implications for multiple audiences. Here, we focus on lay people and public policy makers. We posit that a greater understanding of the water system among lay people will be necessary in order for municipalities and larger levels of government to confront new and exceedingly complex risks to the water system, given that tax payers must approve price hikes to address water shortages and infrastructure upkeep (Reference Olmstead, Hanemann and StavinsOlmstead et al., 2007; Reference SabelliSabelli, 2006).
Systems thinking is also important in order for public policy makers to more fully understand which parts of the integrated system are most at risk. When systems thinking is limited, catastrophes ensue. With respect to water, lead contamination in Flint, Michigan is a clear example of systems thinking failure. Another example in the environmental domain is the Deepwater Horizon oil spill of 2010, during which managers misunderstood pressure data, which begun a catastrophic domino effect that led to the eventual explosion and spill. A similar lack of systems thinking can be seen in the Challenger disaster of 1986, in which O-ring failure, bad weather, and “go fever” contributed to the death of seven space shuttle crewmembers. Capacity to avoid such catastrophes through better decision making can improve to the extent that these systems and potential pitfalls are better understood as an integrated whole (Reference HammondHammond, 1996).
Our work contributes to the existing body of work focusing on systems thinking about the water cycle. For example, Reference Dove, Everett and PreeceDove, Everett, and Preece (1999) found that children draw rivers in rural rather than urban environments, Shepardson et al. (2007b) identified significant misperceptions about watersheds (such as a watershed is a “shed” to store water), and Covitt, Gunkel and Anderson (2009) show that although students have some understanding of water systems, most students fail to trace water through the complete system correctly. Our study contributes to this body of research by (a) creating an accurate expert model of the water system that practitioners can use as part of a communication and education outreach strategy; (b) showing that systems thinking falls off rapidly for non-environmental students when attempting to model the water system beyond the home, a misperception that needs to be corrected given that the cost of treating wastewater far exceeds that of treating drinking water; and (c) risk perceptions of infrastructure are the lowest for non-environmental students, potentially because infrastructure is an “out of sight, out of mind” investment.
This study also contributes to contemporary research in the field of water education and understanding. To our knowledge, ours is the first paper that investigates systems thinking of the natural and anthropogenic system together, rather than primarily focusing on the natural water cycle (Reference Assaraf, Eshach, Orion and AlamourAssaraf et al., 2012). By eliciting and analyzing drawings from university students, we are able to gauge students’ mental models of our current water system compared to an accurate expert-elicited model, without relying on technical terms.
Our research can also be used as a teaching tool. Some scholars have argued that all students ought to learn about the water system (Sadler et al., 2016). Educators focusing on the environment in general or water resources specifically could ask students to draw these diagrams before and after a course to test how understanding improves over the course’s duration. The Next Generation Science Standards, a multi-state effort to create new education standards, focuses on three themes: science and engineering practice, disciplinary core ideas, and crosscutting concepts (NRC, 2013). Under the heading of cross cutting concepts, understanding patterns, cause and effect, scales, systems and system models, structure and function, and how change moves through a system all relate to systems thinking (NRC, 2013). Thus, we argue that understanding the complex water system and focusing on systems thinking is crucial for STEM disciplines as well as an educated electorate.
4.2 Limitations
This study has several limitations. Among them are (1) using a convenience sample; (2) not asking follow-up questions; (3) constructing a consensus expert water system diagram that has not been independently validated; (4) focusing on correlations, which indicate associations, not causation; and (5) the problematic assumption that better system education will produce better problem solving. We examine each of these limitations below.
(1) Convenience sample:
We use a convenience sample of students in a large Midwestern University. Students in more drought-ridden areas or places where more time and effort are expended to collect water for residential use could have more accurate systems understanding. Additionally, students may be well educated but likely have limited real-world experience with the water system, such as not having to pay their own water bills. This limitation may affect the generalizability of our results.
(2) Method of eliciting systems thinking:
Although eliciting students’ drawings serves as a powerful tool to gauge their level of understanding, methods that use open-ended structured interviews could allow for a deeper understanding of misperceptions, due to the ability to ask probing follow-up questions when misperceptions are revealed. Capturing these deeper causal explanations could be invaluable information for environmental educators seeking to correct the misperceptions identified in our work. Deeper explanations could allow us to theorize how to effectively improve understanding of systems by assessing which explanations relate to accuracy (Reference KeilKeil, 2006; Lombrozo, 2006, 2007). Our study also uses a relative limited approach to systems thinking, as we capture interrelations between system processes but miss key features such as systems dynamics, time delays, or feedback. In the future, this limitation can be overcome by using structured interviews or other probes to more deeply understand water systems thinking.
(3) Validity of our expert water diagram:
We interviewed 15 Indiana water experts with backgrounds in water science and management to construct our consensus diagram. Future work can elicit drawings from a new group of independent experts to gauge whether or not the seven features we included in our consensus diagram are also included in their individual diagrams. The accurate water system diagram could have added layers of complexity including feedback loops and other human components such as water managers and engineers who manage and build the water system.
(4) Correlational study showing associations, not causation:
In this research we show that students specializing to some extent in environmental sciences identify more of the water system components shown in the expert portrayal of the water system, and that these students believe that there are more risks to the water system. This work shows association, but does not demonstrate causation. It is plausible that students with more prior awareness are drawn to environmental sciences. One way to tease this limitation apart would be to design a module for an introductory undergraduate course to teach systems thinking, and compare diagrams from students who were and were not exposed to the module.
(5) The problematic assumption that better systems education will produce better problem solving:
This last limitation is much harder to contend with and will need more follow-up work to overcome. It may be the case that people using a vital resource system do not need to know how that system or component of the system functions in order to rely on and use it. In fact, Keil (2003) argues that there may be benefits to using folk science to understand and interact with the increasingly complex world around us, where we know what we need to know in order to function effectively in everyday life.
4.3 Conclusion
Our research reveals specific systematic misperceptions of how the water system works by eliciting and analyzing drawings from university students in a convenience sample. Some highlights of the findings for non-environmental students include the water system halting at the home and low perceptions of risks related to water infrastructure. There are many research questions that stem from this work. Future research could investigate why specific misperceptions exist, how to correct faulty water systems thinking, and to what extent does correcting systems thinking lead to changes in willingness to accept adaptation strategies, such as wastewater reuse, and to accept changes in water prices to account for water scarcity and fortification of our crumbling infrastructure.