Introduction
South America hosts an impressive diversity of extant carnivorans, represented by approximately 45 species (Burgin et al. Reference Burgin, Colella, Kahn and Upham2018). However, this high diversity is a recent phenomenon. For most of the Cenozoic, terrestrial carnivorans were not present in South America. Instead, the terrestrial carnivore guild was primarily composed of a wide variety of non-placental and nonmammalian carnivores, including several groups of metatherians (including sparassodonts; see Croft et al. Reference Croft, Engelman, Dolgushina and Wesley2018 and references therein; Prevosti and Forasiepi Reference Prevosti and Forasiepi2018 and references therein), cariamiform birds (Alvarenga et al. Reference Alvarenga, Chiappe, Bertelli, Dyke and Kaiser2011; Degrange et al. Reference Degrange, Noriega, Areta, Vizcaíno, Kay and Bargo2012; Tambussi and Degrange Reference Tambussi, Degrange, Tambussi and Degrange2013), and terrestrial sebecid crocodyliformes (Pol et al. Reference Pol, Leardi, Lecuona and Krause2012). It is not until the late Miocene that fossils of terrestrial carnivorans—specifically procyonids—are found in South America. These earliest procyonids include two closely related and apparently endemic genera: Cyonasua (late Miocene–middle Pleistocene, Huayquerian to Ensenadan South American Land Mammal Ages [SALMAs]) and Chapalmalania (late early to late Pliocene, Chapadmalalan–Vorohuean SALMAs), hereafter referred to as the “Cyonasua group” following previous authors (e.g., Patterson and Pascual Reference Patterson and Pascual1968; Baskin Reference Baskin1982; Soibelzon and Prevosti Reference Soibelzon, Prevosti, Pons and Vicens2007; Forasiepi et al. Reference Forasiepi, Soibelzon, Gomez, Sánchez, Quiroz, Jaramillo and Sánchez-Villagra2014; Tarquini et al. Reference Tarquini, Vilchez Barral, Soibelzon, Agnolin, Lio, Brissón Egli, Chimento and Novas2016).
Cyonasua, as traditionally defined, appears to be paraphyletic relative to Chapalmalania (Baskin Reference Baskin2004; Forasiepi et al. Reference Forasiepi, Soibelzon, Gomez, Sánchez, Quiroz, Jaramillo and Sánchez-Villagra2014); Chapalmalania is first recorded in the late early Pliocene (Chapadmalalan SALMA) and is thought to have originated via an in situ speciation from a species of Cyonasua (Kraglievich and de Olazabal Reference Kraglievich and de Olazabal1959; Marshall et al. Reference Marshall, Butler, Drake, Curtis and Tedford1979; Baskin Reference Baskin2004; Forasiepi et al. Reference Forasiepi, Soibelzon, Gomez, Sánchez, Quiroz, Jaramillo and Sánchez-Villagra2014). Cyonasua and Chapalmalania do not appear to be closely related to the modern radiation of South American procyonids (Forasiepi et al. Reference Forasiepi, Soibelzon, Gomez, Sánchez, Quiroz, Jaramillo and Sánchez-Villagra2014), and their dispersal to the continent appears to have been separate from that of other procyonid lineages which do not appear in the South America fossil record until much later (Rodriguez et al. Reference Rodriguez, Soibelzon, Rodrigues, Morgan, Bernardes, Avilla and Lynch2013; Forasiepi et al. Reference Forasiepi, Soibelzon, Gomez, Sánchez, Quiroz, Jaramillo and Sánchez-Villagra2014; Prevosti and Forasiepi Reference Prevosti and Forasiepi2018; Ruiz-Ramoni et al. Reference Ruiz-Ramoni, Rincón and Montellano-Ballesteros2019). Two other genera, Parahyaenodon and Tetraprothomo, are also considered to belong to this group but are poorly distinguished from Cyonasua and are probably synonymous with this taxon (Forasiepi et al. Reference Forasiepi, Martinelli and Goin2007).
Cyonasua-group procyonids ranged across South America, from Venezuela and Colombia in the north (Forasiepi et al. Reference Forasiepi, Soibelzon, Gomez, Sánchez, Quiroz, Jaramillo and Sánchez-Villagra2014) to Argentina and Uruguay in the south (Reguero and Candela Reference Reguero, Candela, Salfity and Marquillas2011; Tarquini et al. Reference Tarquini, Vilchez Barral, Soibelzon, Agnolin, Lio, Brissón Egli, Chimento and Novas2016; Soibelzon et al. Reference Soibelzon, Rinderknecht, Tarquini and Ugalde2019). Most specimens of Cyonasua-group procyonids come from sites of late Miocene to early Pliocene age (Huayquerian to Chapadmalalan SALMAs), but the group as a whole is last recorded during the early Pleistocene (based on the Ensenadan Cyonasua meranii; Ameghino and Kraglievich Reference Ameghino and Kraglievich1925; Soibelzon et al. Reference Soibelzon, Gasparini, Zurita and Soibelzon2008; Prevosti et al. Reference Prevosti, Forasiepi and Zimicz2013). The ancestors of Cyonasua-group procyonids reached South America by 7.3 Ma (the age of the oldest well-dated Cyonasua-group procyonid fossil; Reguero and Candela Reference Reguero, Candela, Salfity and Marquillas2011), likely by island hopping or over-water dispersal (Simpson Reference Simpson1950; Marshall Reference Marshall and Nitecki1981; Webb Reference Webb, Stehli and Webb1985; O'Dea et al. Reference O'Dea, Lessios, Coates, Eytan, Restrepo-Moreno, Cione, Collins, de Queiroz, Farris, Norris, Stallard, Woodburne, Aguilera, Aubry, Berggren, Budd, Cozzuol, Coppard, Duque-Caro, Finnegan, Gasparini, Grossman, Johnson, Keigwin, Knowlton, Leigh, Leonard-Pingel, Marko, Pyenson, Rachello-Dolmen, Soibelzon, Soibelzon, Todd, Vermeij and Jackson2016; but see Montes et al. Reference Montes, Cardona, Jaramillo, Pardo, Silva, Valencia, Ayala, Pérez-Angel, Rodriguez-Parra, Ramirez and Niño2015), making them the earliest securely dated North American mammals to participate in the great American biotic interchange (GABI; Webb Reference Webb, Stehli and Webb1985; Woodburne Reference Woodburne2010; but see Prothero et al. Reference Prothero, Campbell, Beatty and Frailey2014 and references therein). Cyonasua-group procyonids were the only carnivorans in South America for nearly 4.3 Myr (until the arrival of canids and mustelids in the late Pliocene Vorohuean SALMA, ~2.9 Ma; Prevosti and Forasiepi Reference Prevosti and Forasiepi2018) and the only ones to coexist with sparassodonts, the primary group of endemic mammalian carnivores (Forasiepi et al. Reference Forasiepi, Martinelli and Goin2007; Prevosti et al. Reference Prevosti, Forasiepi and Zimicz2013; Prevosti and Forasiepi Reference Prevosti and Forasiepi2018). Most groups of carnivorans, including felids and ursids, do not appear in the South American fossil record until the Pleistocene. However, exactly how and why Cyonasua-group procyonids were able to establish themselves in South America nearly 4 million years earlier than other carnivorans (and long before most other groups of North American mammals) has never been examined in detail.
In this study, we test the hypothesis that ecological dissimilarity to incumbent metatherian carnivores was an important contributing factor to the early appearance of Cyonasua-group procyonids in South America. We do so by examining the functional morphology of the dentition of Cyonasua-group procyonids relative to other late Miocene–early Pliocene South American mammalian carnivores, particularly sparassodonts, and evaluating their positions in ecological morphospace. Based on this information, we reconstruct the positions of these taxa within the late Miocene–Pliocene South American predator guild and infer the degree to which there may have been ecological (specifically dietary) overlap between these groups. The results are discussed in the context of other factors that may have affected the propensity of Cyonasua-group procyonids to disperse to and establish themselves in South America.
Materials and Methods
For this analysis, we began with the mostly genus-level data set of Croft et al. (Reference Croft, Engelman, Dolgushina and Wesley2018), which coded nearly all sparassodonts, most predatory late Neogene didelphoids, and a broad comparative sample of extant carnivorans for 16 characters describing the functional morphology of the dentition and 1 character describing body mass. To this data set, we added one new character, the number of upper molars with grinding surfaces (described later), and coded seven additional taxa: the late Neogene South American procyonids Cyonasua and Chapalmalania, FMNH P14407 (a sparassodont from the late Miocene or Pliocene of Corral Quemado, Argentina), and four extinct North American procyonids (also detailed later). This resulted in a data set of 18 characters (listed in Supplementary Table 1) and 365 taxa. Additional modifications to the data set of Croft et al. (Reference Croft, Engelman, Dolgushina and Wesley2018) are described in Supplementary Table 2, and specimens examined for comparative purposes and used to add new codings and taxa are detailed in Supplementary Table 3. The complete data set used for this study is presented in Supplementary Table 4. Following previous studies (e.g., Wesley-Hunt Reference Wesley-Hunt2005; Prevosti et al. Reference Prevosti, Forasiepi and Zimicz2013; Croft et al. Reference Croft, Engelman, Dolgushina and Wesley2018; Tarquini et al. Reference Tarquini, Chemisquy and Prevosti2018b) the P4/m1 of carnivorans and the penultimate upper/ultimate lower molar in non-carnivoramorphan carnivores (M3/m4 in carnivorous metatherians) were considered to be functionally analogous.
The new character developed for this analysis (18: number of upper teeth with grinding surfaces) was incorporated to more accurately reflect the functional morphology of metatherian dentitions. Examination of the parent data set found that the most strongly loaded character on the first axis was the “number of post-carnassial upper teeth” (character 14). However, this character showed strong phylogenetic signal, as the primary carnassials in metatherians are almost always M3/m4, and thus in metatherians there is always one upper molar posterior to the carnassial regardless of dietary habits. Similarly, the fact that metatherians often have grinding surfaces on molars anterior to the primary carnassials (i.e., on M/m1-2) were unaccounted for in the parent study. Teeth were coded having a grinding surface if there was at least one structure (e.g., protocone) that could function in occlusion.
Body-mass estimates for Cyonasua and Chapalmalania were taken from Tarquini et al. (Reference Tarquini, Toledo, Soibelzon and Morgan2018a) and Prevosti and Forasiepi (Reference Prevosti and Forasiepi2018), respectively, which can be consulted for additional information. Details of other character codings for Cyonasua and Chapalmalania are included in Supplementary Table 5.
FMNH P14407, a sparassodont specimen from the late Miocene or Pliocene of Corral Quemado, Argentina, was added to this analysis, because it appears to represent a distinct taxon from this time period. The taxonomy of this specimen is uncertain: Riggs (Reference Riggs1934) referred it to Acrohyaenodon acutidens, whereas Marshall (Reference Marshall1978: pp. 65–66) tentatively referred “A.” acutidens to the borhyaenid genus Eutemnodus. Forasiepi et al. (Reference Forasiepi, Martinelli and Goin2007) considered FMNH P14407 to represent an indeterminate “prothylacynine” (basal borhyaenoid). Our observations of FMNH P14407 agree with Forasiepi et al. (Reference Forasiepi, Martinelli and Goin2007); the morphology of this specimen (including the absence of a metaconid and presence of a multicusped talonid) is more similar to that of borhyaenoids such as Prothylacynus than borhyaenids or thylacosmilids. Despite the uncertain taxonomic status of FMNH P14407, its morphology is distinct from other sparassodonts of this age. We could not examine the holotype of “A.” acutidens firsthand, and whether this specimen pertains to the same taxon as FMNH P14407 is beyond the scope of this study.
Four genera of extinct North American procyonids, Arctonasua, Bassaricyonoides, Probassariscus, and Protoprocyon, were added to this analysis to better represent the diversity of Procyonidae, the focus of this study. Body masses of Protoprocyon and Probassariscus were coded based on extant, similar-sized Bassariscus spp. (Baskin Reference Baskin1982) and body mass of Bassaricyonoides was coded based on extant Bassaricyon (Baskin Reference Baskin2003). Body mass of Arctonasua was estimated at ~9.5 kg using the occipito-orbital length regression equation of Van Valkenburgh (Reference Van Valkenburgh, Damuth and MacFadden1990) and a measurement of 89.92 mm from AMNH F:AM 50054 (Arctonasua fricki; Baskin Reference Baskin1982).
The expanded data set was analyzed via correspondence analysis (CA) in PAST v. 3.21 (Hammer et al. Reference Hammer, Harper and Ryan2001) following the methodology of Croft et al. (Reference Croft, Engelman, Dolgushina and Wesley2018) and other studies of carnivore ecomorphology (Wesley-Hunt Reference Wesley-Hunt2005; Werdelin and Wesley-Hunt Reference Werdelin, Wesley-Hunt, Goswami and Friscia2010; Werdelin and Lewis Reference Werdelin and Lewis2013). CA has been used in these studies rather than principal components analysis because the data used here consist of a combination of both continuous (e.g., shape of canine, premolar dimensions, carnassial blade length) and discrete (e.g., shape of incisor row, number of premolars, shape of carnassial, number of molars) characters.
Missing data in correspondence analyses are typically handled via column average substitution. However, this can result in “centroid slippage” (Flannery Sutherland et al. Reference Flannery Sutherland, Moon, Stubbs and Benton2019), in which incomplete taxa plot closer to the origin than they should. This can be especially problematic when more than one major clade is being analyzed, as in this study, as missing values are reconstructed using all groups, potentially overinflating morphological disparity and creating phylogenetically incongruous scenarios. To minimize such issues in this analysis, missing values were substituted with the clade average rather than the column (i.e., entire data set) average; specifically, separate average values were calculated manually for carnivorans, sparassodonts, dasyuromorphians, and didelphimorphians and used to fill missing values.
Institutional Abbreviations
AMNH, American Museum of Natural History, New York, N.Y., U.S.A.; FMNH, The Field Museum, Chicago, Ill., U.S.A.; MLP, Museo de La Plata, La Plata, Argentina.
Anatomical Abbreviations
P/p, upper and lower premolars; M/m, upper and lower molars.
Results
The results of the CA (Fig. 1) broadly resemble those of previous studies (Wesley-Hunt Reference Wesley-Hunt2005; Werdelin and Wesley-Hunt Reference Werdelin, Wesley-Hunt, Goswami and Friscia2010; Werdelin and Lewis Reference Werdelin and Lewis2013; Croft et al. Reference Croft, Engelman, Dolgushina and Wesley2018). The first axis (CA 1), representing 41.5% of the total variation, generally correlates with diet, with hypocarnivores exhibiting highly positive values and hypercarnivores generally exhibiting highly negative values. The second axis (CA 2), representing 16.0% of the total variation, correlates most strongly (negatively) with body mass, the angle of upper carnassial cusps in occlusal view, and, to a lesser extent (positively), with the number and robustness of premolars (Fig. 2). The first two axes represent 57.5% of the total variation. A complete list of axis eigenvalues and taxon scores can be found in Supplementary Tables 6 and 7, respectively.
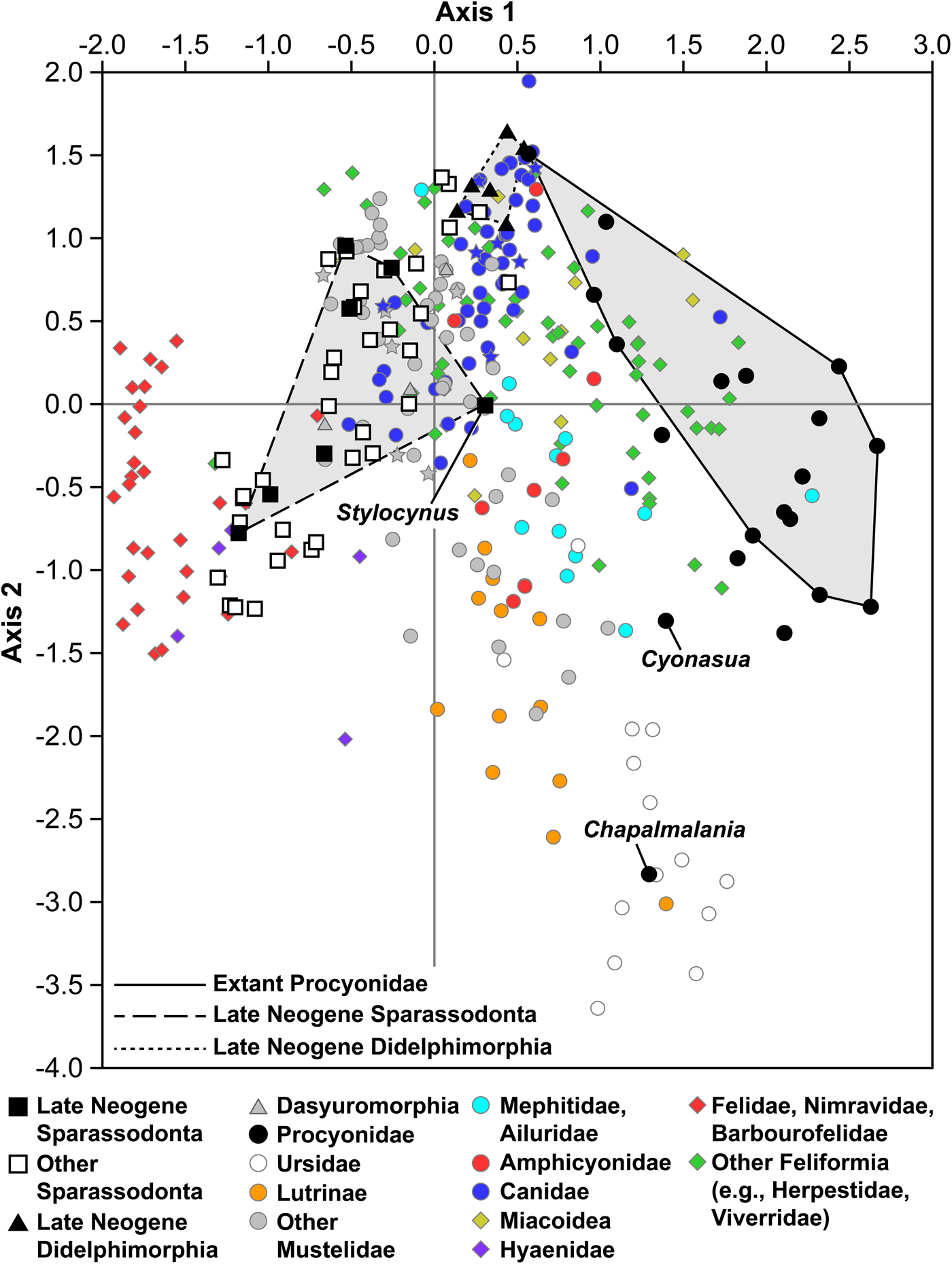
Figure 1. Plot of the first two axes of the correspondence analysis of all taxa used in this analysis. Carnivoran placentals are represented by circles and diamonds (caniformians and feliformians/miacoids, respectively). Cerdocyonin canids, ictonychine mustelids, and Eira are denoted by stars (see “Discussion”).

Figure 2. Vector plot of the eigenvalues of the first two axes for the 18 characters used in the correspondence analysis, showing the extreme negative weight of body mass (BM) on axis 2. Abbreviations: BCA, angle of lower carnassial cusps in buccal view; BM, body mass; CAN, shape of upper canines; DIA, presence of diastemata among upper premolars; INC, shape of upper incisor row; LBL, lower carnassial relative blade length; LCA, angle of lower carnassial cusps in occlusal view; LPS, shape of last lower premolar anterior to carnassial; #MO, number of upper molars; MOC, shape of cusps of first upper molar; MOS, shape of first upper post-carnassial tooth; RGA, relative grinding area of lower molars; UBL, upper carnassial relative blade length; UCA, angle of upper carnassial cusps in occlusal view; UCS, shape of upper carnassial; UGR, number of upper teeth with grinding surfaces; #UP, number of upper premolars anterior to the carnassial; UPS, shape of last upper premolar anterior to carnassial.
Both Cyonasua and Chapalmalania have high CA 1 values and low CA 2 values (Cyonasua: 1.394, −1.305; Chapalmalania: 1.293, −2.832) and plot farther from extant procyonids than other extinct members of this group. On CA 1, Cyonasua and Chapalmalania plot within the range of values spanned by extant procyonids (Fig. 1), between Bassariscus and all other extant species. By contrast, Cyonasua and Chapalmalania score more negatively on CA 2 than extant procyonids and fall outside the convex hull of morphospace occupied by extant members of this group. The more negative positions of Cyonasua and Chapalmalania on CA 2 are likely due to size; this is the only character for which the scores of Cyonasua or Chapalmalania are outside the range of extant procyonids, and body mass is the character most strongly correlated (negatively) with CA 2 (Fig. 2).
Cyonasua plots in an area occupied by various extant hypocarnivores, including ursids, mephitids (e.g., Conepatus spp.), mustelids (e.g., Meles and Taxidea), and the extant binturong (Arctictis binturong) (Fig. 1). Chapalmalania plots just within the morphospace of extant ursids, to the right of non-hemicyonine ursids that are known or have been considered to have more predatory habits (Agriotherium, Arctodus, Ursus maritimus) and to the left of more omnivorous ursid species (Ursus spp. other than U. maritimus, Melursus ursinus, Tremarctos ornatus). The four extinct North American procyonids added for this analysis plot close to extant species; Bassaricyonoides, Probassariscus, and Protoprocyon plot between Bassariscus and all other extant procyonids, whereas Arctonasua plots close to Procyon pygmaeus and other extant species.
The new character added for this study (character 18) resulted in sparassodonts being more widely distributed on CA 1 than in the analysis of Croft et al. (Reference Croft, Engelman, Dolgushina and Wesley2018). Similarly, didelphimorphians plot more positively on CA 1 than in Croft et al. (Reference Croft, Engelman, Dolgushina and Wesley2018), close to canids such as Vulpes spp. and Urocyon spp. Sparassodont morphospace can generally be described as a relatively narrow band that spans the lower left, upper left, and a small portion of the upper right quadrants of Figure 1, with higher CA 1 values generally correlating with higher CA 2 values. Nevertheless, four main clusters of sparassodonts can be identified (see Supplementary Fig. 2) that correlate with decreasing specializations for carnivory: (1) Proborhyaena and Paraborhyaena (Proborhyaenidae) plus Australohyaena and Arctodictis (Borhyaenidae), which show the greatest specializations for carnivory and are adjacent to felid morphospace; (2) other highly specialized carnivorous borhyaenoids (Prothylacynus, Thylacosmilidae, remaining proborhyaenids and borhyaenids), in an area of morphospace sparsely populated by carnivorans but including hyaenids and the euplerid Cryptoprocta; (3) remaining borhyaenoids (e.g., Lycopsis), larger hathliacynids such as Cladosictis and Acyon, and some smaller hathliacynids such as Notogale and all late Neogene taxa, occupying a region alongside hypercarnivorous mustelids (Gulo, Mellivora, Mustela) and hypercarnivorous canids (e.g., Aelurodon, Canis lupus); and (4) smaller sparassodonts (e.g., Pseudonotictis, Patene, Sipalocyon, Perathereutes) in an area mostly occupied by canids at the threshold between mesocarnivory and hypercarnivory (e.g., Vulpes velox, Pseudalopex culpaeus, Pseudalopex griseus). The basal sparassodonts Hondadelphys and Stylocynus are outliers and plot more positively on CA 1 than other sparassodonts. Late Neogene sparassodonts cluster into three widely spaced groups: larger borhyaenoids (Thylacosmilus, Borhyaenidae indet., and FMNH P14407), the former two plotting within group 2 and the third in between groups 2 and 3; small hathliacynids (Borhyaenidium, Notictis, and Notocynus), which all fall within group 3; and Stylocynus, which as mentioned earlier, is an outlier to all of the groups listed above. It is possible the well-defined separation between groups observed here is an artifact of binning the data and that the actual separation between taxa may be more of a gradient.
There is almost no overlap among late Neogene sparassodonts, didelphimorphians, and procyonids, except for the procyonid Bassariscus astutus, which plots close to Didelphis but is not within didelphimorphian morphospace. This position may be due to the fact that smaller animals seem to cluster together regardless of dietary habits, which may be due to how the algorithm used by PAST analyzes the data, or may reflect smaller mammals with disparate habits being more generalized in morphology than larger ones. There is some overlap between didelphimorphian and sparassodont morphospace, but sparassodonts and didelphimorphians in this region do not overlap temporally (the sparassodonts are Paleogene or early Miocene taxa like Pseudonotictis or Patene). Small-bodied late Neogene taxa (e.g., Borhyaenidium, Notocynus) are positioned more distantly in morphospace.
Neither Cyonasua nor Chapalmalania are close to the morphospace occupied by didelphimorphians or late Neogene sparassodonts (or the area occupied by Sparassodonta as a whole, for that matter). Among sparassodonts, the late middle Miocene Hondadelphys plots closest to Procyonidae. Stylocynus, the sparassodont genus often considered to have been most similar to Cyonasua and Chapalmalania in its habits (Marshall Reference Marshall, Hecht, Goody and Hecht1977; Prevosti et al. Reference Prevosti, Forasiepi and Zimicz2013; Prevosti and Forasiepi Reference Prevosti and Forasiepi2018), does not plot close to these genera in our morphospace analysis, though it does plot closer to Cyonasua-group procyonids than any other sparassodont. Instead, Stylocynus plots near the origin, close to several mesocarnivorous or hypercarnivorous carnivorans such as Canis lupus, Chrysocyon brachyurus, and Spilogale spp.
Discussion
Dietary Habits of Cyonasua-Group Procyonids
Overall, the positions of Cyonasua and Chapalmalania in the CA suggest that these taxa were hypocarnivores more specialized for carnivory than most living procyonids, in agreement with previous studies (Berman Reference Berman1994; Soibelzon Reference Soibelzon2011; Reyes et al. Reference Reyes, Poiré, Soibelzon, Zurita and Arrouy2013); Cyonasua and Chapalmalania plot to the left of large-bodied procyonids such as Nasua and Procyon on CA 1, suggesting more carnivorous habits, but to the right of the extant Bassariscus, among other hypocarnivores (e.g., ursids). Omnivorous habits are supported by the gross morphology of the dentitions of Cyonasua and Chapalmalania, which show well-developed grinding surfaces and little development of shearing features compared with most carnivorans. The only non-hypocarnivores that plot close to Cyonasua and Chapalmalania are otters (e.g., Enhydra, Aonyx) and polar bears (U. maritimus); otters tend to have well-developed crushing features on their molars related to their consumption of hard-shelled invertebrates, and polar bears show few dental specializations for carnivory (Sacco and Van Valkenburgh Reference Sacco and Van Valkenburgh2004; Oldfield et al. Reference Oldfield, McHenry, Clausen, Chamoli, Parr, Stynder and Wroe2011), likely due to their unusual pinniped-heavy diet (Oldfield et al. Reference Oldfield, McHenry, Clausen, Chamoli, Parr, Stynder and Wroe2011) and their relatively recent divergence from the omnivorous brown bear (Ursus arctos) (Lindqvist et al. Reference Lindqvist, Schuster, Sun, Talbot, Qi, Ratan, Tomsho, Kasson, Zeyl, Aars, Miller, Ingólfsson, Bachmann and Wiig2010). It is unlikely that the well-developed grinding surfaces of Cyonasua and Chapalmalania are adaptations for specialized bone- or hard object–feeding diet (although there is evidence of scavenging behavior by Chapalmalania; Reyes et al. Reference Reyes, Poiré, Soibelzon, Zurita and Arrouy2013), as the teeth of both Cyonasua and Chapalmalania are relatively small in relation to body and skull size (Prevosti and Forasiepi Reference Prevosti and Forasiepi2018; Tarquini et al. Reference Tarquini, Toledo, Soibelzon and Morgan2018a). Relatively small teeth are unexpected for a durophagous specialist but are seen in some extant frugivores (Kay Reference Kay1975; Gingerich and Smith Reference Gingerich, Smith and Jungers1985; Strait Reference Strait1993).
The positions of Cyonasua and Chapalmalania on CA 1 fit the prediction of Werdelin and Wesley-Hunt (Reference Werdelin, Wesley-Hunt, Goswami and Friscia2010) that the large gap between Bassariscus spp. and other extant procyonids in their morphospace analysis is the result of the extinction of procyonids of intermediate dental morphology. Cyonasua and Chapalmalania partially fill this gap, at least on CA 1, which supports this idea. Additionally, three of the four fossil North America procyonids added to this analysis, Protoprocyon, Probassariscus, and Bassaricyonoides, plot between Bassariscus and all other extant procyonids.
Cyonasua and Chapalmalania also exhibit a mosaic of character codings relative to other procyonids, with some features (e.g., incisor row shape, upper carnassial relative blade length, relative grinding area) more closely resembling Bassariscus and others (shape of the upper carnassial, lower carnassial relative blade length) more closely resembling “typical” procyonids such as Bassaricyon, Procyon, and Nasua. Although Cyonasua and Chapalmalania plot outside the morphospace occupied by extant procyonids, this is mainly due to their larger size; changing the body-mass codings for Cyonasua and Chapalmalania to state 1 (<1.5 kg, similar to Bassariscus) results in these two taxa plotting just outside extant procyonid morphospace rather than far from it. The fact that Chapalmalania plots within the ursid morphospace is not surprising, given that this species has often been considered to be morphologically and ecologically similar to this group (Kraglievich and Olazabal Reference Kraglievich and de Olazabal1959; Forasiepi et al. Reference Forasiepi, Soibelzon, Gomez, Sánchez, Quiroz, Jaramillo and Sánchez-Villagra2014).
Competition between Cyonasua-Group Procyonids and South American Metatherians
It was once thought that the endemic South American carnivores, particularly the sparassodonts, were driven to extinction by competition with Northern Hemisphere carnivorans during the GABI (Simpson Reference Simpson1950, Reference Simpson1980; Patterson and Pascual Reference Patterson and Pascual1968; Marshall Reference Marshall1976; Werdelin Reference Werdelin1987). However, it has become apparent in recent years that there was little temporal overlap between sparassodonts and carnivorans; most sparassodonts are not recorded in the same intervals as their purported Northern Hemisphere replacements, making hypotheses of competitive displacement unlikely (Marshall Reference Marshall, Hecht, Goody and Hecht1977; Forasiepi et al. Reference Forasiepi, Martinelli and Goin2007; Prevosti et al. Reference Prevosti, Forasiepi and Zimicz2013; Prevosti and Forasiepi Reference Prevosti and Forasiepi2018). Competition between didelphimorphians and sparassodonts is still an open question, though most studies have suggested ecological partitioning and passive replacement of small sparassodonts by didelphimorphians in the Neogene (Engelman and Croft Reference Engelman and Croft2014; Zimicz Reference Zimicz2014; but see Beck and Taglioretti Reference Beck and Taglioretti2019). This is supported by the results of this study; there is no overlap among late Neogene sparassodonts and didelphimorphians, but late Miocene–Pliocene didelphimorphians do overlap Paleogene–early Miocene sparassodonts.
Cyonasua and Chapalmalania are the only South American carnivorans currently known to overlap sparassodonts in time and may have been the only terrestrial carnivorans to directly interact with them. It has been suggested that significant competitive interaction may have occurred between Cyonasua-group procyonids and some sparassodonts, particularly the genus Stylocynus (Marshall Reference Marshall, Hecht, Goody and Hecht1977; Soibelzon Reference Soibelzon2011; Prevosti et al. Reference Prevosti, Forasiepi and Zimicz2013; Engelman and Croft Reference Engelman and Croft2014), due to similar habits and these animals overlapping in time. Cyonasua and Chapalmalania are positioned very far from the morphospace occupied by South America metatherians (Didelphimorphia and Sparassodonta), which suggests little to no ecological overlap with these groups (Fig. 1), in agreement with most previous studies. Although the degree to which sparassodonts were omnivorous has been debated (Marshall Reference Marshall, Hecht, Goody and Hecht1977; Prevosti et al. Reference Prevosti, Forasiepi and Zimicz2013; Croft et al. Reference Croft, Engelman, Dolgushina and Wesley2018), virtually all authors agree that no South American metatherian (sparassodont or didelphimorphian), with the possible exception of Stylocynus, was as omnivorous as extant procyonids or ursids. However, even Stylocynus plots outside the hypocarnivore morphospace occupied by Ailurus, procyonids, and ursids (as well as Cyonasua-group procyonids), closer to mesocarnivorous or even hypercarnivorous taxa such as Canis lupus, Chrysocyon brachyurus, and Spilogale spp. Prevosti et al. (Reference Prevosti, Forasiepi and Zimicz2013) considered Stylocynus to be hypocarnivorous based on relative grinding area but did not exclude the possibility of a mesocarnivorous diet. The results of this analysis and Croft et al. (Reference Croft, Engelman, Dolgushina and Wesley2018) support the idea of a more mesocarnivorous diet for Stylocynus.
The specific ways in which the dentitions of Stylocynus and Cyonasua-group procyonids differ from one another give some insight into how these animals’ diets may have differed. Cyonasua-group procyonids have more robust premolars than Stylocynus and upper carnassials with blunt cusps that are well built for crushing (Fig. 3). By contrast, the premolars of Stylocynus are labiolingually narrower and have much smaller posterobasal heels than Cyonasua-group procyonids. Similarly, the molars of Stylocynus have well-developed paracristids and tall trigonid cusps, particularly on m3-4 (Fig. 4), whereas the lower carnassial of Cyonasua-group procyonids (the functionally equivalent tooth) has almost no blade at all and a trigonid that is only slightly more prominent than that of Nasua and Procyon. The mandibular symphysis of Cyonasua-group procyonids is also less extensive and more vertical than in Stylocynus; in both Cyonasua and Chapalmalania the symphysis ends at the p1/2 embrasure and is dorsoventrally much taller than anteroposteriorly long, whereas in Stylocynus the symphysis ends below the anterior root of p3 and is anteroposteriorly much longer than dorsoventrally tall. However, the surface of the symphysis of Cyonasua-group procyonids is much more rugose than that of Stylocynus, in which it is smooth (state 2/3 vs. state 1 of Scapino [Reference Scapino1981]). The morphology of the symphysis in Cyonasua-group procyonids appears to be an apomorphy; the mandibular symphysis is unfused and smooth in most extant procyonids aside from Potos and Procyon (Scott et al. Reference Scott, Hogue and Ravosa2012). These features suggest that, even though procyonids may have had more ecological overlap with Stylocynus than other sparassodonts, they occupied distinct ecological niches; Stylocynus was more specialized for feeding on meat (likely mesocarnivorous), whereas Cyonasua-group procyonids were more specialized for feeding on other foods, such as fruit, vegetation, and invertebrates (hypocarnivorous).

Figure 3. Lower premolar rows of Cyonasua-group procyonids and Stylocynus (left p1-4 and p1-3, respectively) in occlusal view, showing the more robust lower premolars of Cyonasua-group procyonids and more sectorial teeth of Stylocynus. A, Cyonasua lutaria (AMNH 117419); B, Chapalmalania altaefrontis (MLP 91-VI-5-1); C, Stylocynus paranensis (MLP 41-XII-13-1112, reversed). Anterior is to the right. Scale bar, 10 mm.

Figure 4. Posterior molars of Cyonasua-group procyonids and Stylocynus (left m1-2 and m3-4, respectively) in lateral view, showing the well-developed trigonid and paracristid in Stylocynus compared with the more bunodont morphology in procyonids. The m1 of carnivorans and the m4 of meat-eating metatherians are generally considered to be functionally analogous teeth. A, Cyonasua lutaria (AMNH 117419); B, Chapalmalania altaefrontis (MLP 91-VI-5-1); C, Stylocynus paranensis (MLP 11-94). Anterior is to the left. Scale bar, 10 mm.
Cyonasua-group procyonids and Stylocynus apparently also differed from one another in body mass, which would have further reduced competition (Prevosti et al. Reference Prevosti, Forasiepi and Zimicz2013; Prevosti and Forasiepi Reference Prevosti and Forasiepi2018). Body mass plays an important role in resource partitioning in modern carnivores, with larger species generally being dominant in competitive interactions (Creel and Creel Reference Creel and Creel1996; Palomares and Caro Reference Palomares and Caro1999; Linnell and Strand Reference Linnell and Strand2000). The mass of Cyonasua is generally estimated at 16–21 kg (though at least one larger individual has been noted; Tarquini et al. Reference Tarquini, Toledo, Soibelzon and Morgan2018a), whereas Stylocynus was probably at least one-third larger (28–35 kg; Croft et al. Reference Croft, Engelman, Dolgushina and Wesley2018; Prevosti and Forasiepi Reference Prevosti and Forasiepi2018), and even larger individuals have been documented (e.g., MLP 41-XII-13-1112 appears to belong to a larger individual than MLP 11-94, the specimen from which most body-mass estimates of Stylocynus have been calculated; Marshall Reference Marshall1979). The disparity in body size (Hutchinson's ratio) between Cyonasua and Stylocynus is at minimum 1.33, which is above the critical threshhold of similarity for ecological coexistence between similar taxa (usually considered to be 1.3 or less; Hutchinson Reference Hutchinson1959; Tambussi and Degrange Reference Tambussi, Degrange, Tambussi and Degrange2013), suggesting these animals were not direct competitors. Chapalmalania was far larger than both Cyonasua and Stylocynus, with a body mass estimated at 120–180 kg (Prevosti and Forasiepi Reference Prevosti and Forasiepi2018), but this taxon is first recorded approximately 2 million years after the last record of Stylocynus (Prevosti et al. Reference Prevosti, Forasiepi and Zimicz2013), suggesting that these taxa may not have overlapped in time. The new evidence presented here reinforces conclusions of previous studies that competitive exclusion was not a significant factor in the extinction of the Sparassodonta (e.g., Marshall Reference Marshall, Hecht, Goody and Hecht1977; Forasiepi et al. Reference Forasiepi, Martinelli and Goin2007; Prevosti et al. Reference Prevosti, Forasiepi and Zimicz2013; Engelman and Croft Reference Engelman and Croft2014; Zimicz Reference Zimicz2014; López-Aguirre et al. Reference López-Aguirre, Archer, Hand and Laffan2017; Croft et al. Reference Croft, Engelman, Dolgushina and Wesley2018; Prevosti and Forasiepi Reference Prevosti and Forasiepi2018).
Colonization of South America by Cyonasua-Group Procyonids
Why Cyonasua-group procyonids were able to colonize South America long before most other groups of carnivorans remains an unanswered question in studies of the GABI. This colonization is even more unusual in that the oldest record of Cyonasua-group procyonids dates to the late Miocene, when the endemic carnivore guild of South America was ecologically diverse (Vizcaíno and De Iuliis Reference Vizcaíno and De Iuliis2003; Tambussi and Degrange Reference Tambussi, Degrange, Tambussi and Degrange2013; Zimicz Reference Zimicz2014; Prevosti and Forasiepi Reference Prevosti and Forasiepi2018) and theoretically more robust to invasion, whereas the arrival and diversification of other carnivorans appears to postdate a late Pliocene collapse of the native carnivore guild (including the extinction of the Sparassodonta), resulting in low carnivore diversity and many open ecological niches during the late Pliocene–early Pleistocene (Van Valkenburgh Reference Van Valkenburgh1991; Vizcaíno et al. Reference Vizcaíno, Fariña, Zárate, Bargo and Schultz2004; Forasiepi et al. Reference Forasiepi, Martinelli and Goin2007; Mitchell et al. Reference Mitchell, Bray, Bover, Soibelzon, Schubert, Prevosti, Prieto, Martin, Austin and Cooper2016; Prevosti and Forasiepi Reference Prevosti and Forasiepi2018).
One possible explanation for the apparent early dispersal of Cyonasua-group procyonids to South America relative to other carnivores is that this is an artifact of the fossil record. In support of this idea, it been suggested (based on genetic evidence) that some other groups of carnivorans (i.e., non–Cyonasua-group procyonids such as Nasua and some mustelids like Galictis and Pteronura) colonized South America in the middle or late Miocene (Koepfli et al. Reference Koepfli, Gompper, Eizirik, Ho, Linden, Maldonado and Wayne2007; Eizirik Reference Eizirik, Patterson and Costa2012). Although such a scenario cannot be ruled out completely, it seems quite unlikely based on the current fossil record. Several well-studied middle to late Miocene sites are known from equatorial South America (e.g., Acre, La Venta, Urumaco) and represent a diversity of paleoenvironments, some of which preserve the remains of small, arboreal mammals similar to small procyonids (e.g., bats, platyrrhine primates, and didelphids; Czaplewski Reference Czaplewski1996; Fleagle et al. Reference Fleagle, Kay, Anthony, Kay, Madden, Cifelli and Flynn1997; Goin Reference Goin, Kay, Madden, Cifelli and Flynn1997). La Venta is particularly noteworthy in this regard; located only about 600 km from the Panamanian Isthmus, it is among the most heavily sampled fossil sites in South America (Kay et al. Reference Kay, Madden, Cifelli and Flynn1997 and references therein) and has yet to produce any remains of terrestrial North American mammal groups. It is highly unlikely that Cyonasua-group procyonids (or any North American mammals for that matter) were present in South America at the time this locality's sediments were deposited (~13–12 Ma; Flynn et al. Reference Flynn, Guerrero, Swisher, Kay, Madden, Cifelli and Flynn1997).
There is no evidence of non–Cyonasua-group procyonids in South America before the late Pliocene. By contrast, 39 specimens of Cyonasua-group procyonids are known from this interval (Prevosti and Forasiepi Reference Prevosti and Forasiepi2018; Soibelzon et al. Reference Soibelzon, Rinderknecht, Tarquini and Ugalde2019), including specimens from low-latitude sites (Forasiepi et al. Reference Forasiepi, Soibelzon, Gomez, Sánchez, Quiroz, Jaramillo and Sánchez-Villagra2014). Thus, the absence of other carnivorans during this interval is not simply a preservation bias against carnivores or carnivorans or a restricted geographic or ecological distribution of Cyonasua-group procyonids. Assuming Cyonasua-group procyonids were the earliest carnivorans to successfully colonize South America, the question becomes why these animal succeeded when other carnivorans did not.
Carnivorans in general are considered to be poor over-water dispersers compared with other mammals (Meiri et al. Reference Meiri, Dayan and Simberloff2004: pp. 474–475; Lyras et al. Reference Lyras, van der Geer and Rook2010), with most extant island carnivorans representing populations on continental islands that became isolated by rising sea levels at the end of the last ice age (rather than dispersing over water to these locations) or, perhaps, were introduced by humans (e.g., Urocyon littoralis; Rick et al. Reference Rick, Erlandson, Vellanoweth, Braje, Collins, Guthrie and Stafford2009). However, several aspects of procyonid ecology suggest that they may be better over-water dispersers than most other carnivorans. First, nearly all known procyonids, both living and extinct, have at least some climbing or grasping ability (Tarquini et al. Reference Tarquini, Toledo, Morgan and Soibelzon2017), which might increase their chances of being swept out to sea on a floating tree or a mat of vegetation. Second, strong swimming abilities might further increase the chances of a procyonid successfully making it to shore in a chance over-water dispersal. Raccoons (Procyon spp.) are strong swimmers (Bigler et al. Reference Bigler, Hoff and Johnson1981; Zeveloff Reference Zeveloff2002) and coatis (Nasua spp.) have also been reported to swim (Gompper Reference Gompper1997). Third, the opportunistic (omnivorous) dietary habits of procyonids may have made them more likely to survive a multiday over-water dispersal event than a mammal more dependent on meat. Such habits also allow hypocarnivorous species like procyonids to exist at greater population densities than mesocarnivores or hypercarnivores. For example, in their analysis of Neotropical mammalian community structure, Robinson and Redford (Reference Robinson and Redford1986) found that population densities of hypocarnivorous carnivorans like procyonids and Conepatus were generally at least an order of magnitude greater than those of mesocarnivorous or hypercarnivorous taxa like Eira, Galictis, and felids. Higher population densities may have increased the probability of a sweepstakes dispersal event by a procyonid rather than another North American carnivoran. At least three island procyonid populations are considered to represent natural over-water dispersals: the Cozumel island raccoon (P. pygmaeus), the Tres Marias raccoon (Procyon lotor insularis), and the Cozumel island coati (Nasua narica nelsoni) (Helgen and Wilson Reference Helgen, Wilson, Sánchez-Cordero and Medellín2005; McFadden et al. Reference McFadden, Gompper, Valenzuela and Morales2008; Cuaron et al. Reference Cuaron, Valenzuela-Galvan, Garcia-Vasco, Copa, Bautista, Mena, Martinez-Godinez, Gonzalez-Baca, Bojorquez-Tapia, Barraza, Grammont, de, Galindo-Maldonado, Martinez-Morales, Vazquez-Dominguez, Andresen, Benitez-Malvido, Perez-Salicrup, McFadden and Gompper2009). The Tres Marias raccoon demonstrates that procyonids are capable of dispersing over at least 25 km of open water, the shortest distance between the Tres Marias archipelago and the mainland during the Pleistocene (Ortiz-Ramírez et al. Reference Ortiz-Ramírez, Sánchez-González, Castellanos-Morales, Ornelas and Navarro-Sigüenza2018)
In addition to dispersing to a new environment, successful colonization requires establishing a viable population. In this regard, the ecological uniqueness of procyonids relative to the endemic South American biota may have favored their establishment in South America long before other carnivores. Studies of historical invasions of extant species have found that ecological dissimilarity between the dispersing species and the species of the new community is an important predictor of successful establishment (Darwin's naturalization hypothesis; see Sih et al. Reference Sih, Bolnick, Luttbeg, Orrock, Peacor, Pintor, Preisser, Rehage and Vonesh2010; Pearson et al. Reference Pearson, Ortega and Sears2012; Azzurro et al. Reference Azzurro, Tuset, Lombarte, Maynou, Simberloff, Rodríguez-Pérez and Solé2014; Skóra et al. Reference Skóra, Abilhoa, Padial and Vitule2015; Vidal-García and Keogh Reference Vidal-García and Keogh2017). Ecological dissimilarity allows immigrant species to exploit the environment in ways that native species do not, thereby avoiding direct competition. This situation is analogous to that inferred for procyonids and metatherian carnivores in Cenozoic South America. No South American metatherian seems to have been a large (>1.5 kg), specialized hypocarnivore similar to a bear, procyonid, or the modern red panda (Werdelin Reference Werdelin1987; Wroe et al. Reference Wroe, Argot and Dickman2004; Orcutt Reference Orcutt2015), though some extinct armadillos may have had hypocarnivorous dietary habits similar to Cyonasua-group procyonids (e.g., Macroeuphractus; Vizcaíno and De Iuliis Reference Vizcaíno and De Iuliis2003).
The hypothesis that ecological dissimilarity played a role in the early colonization of South America by Cyonasua-group procyonids is supported by comparing the position of procyonids in the results of the CA to canids, ictonychine (= galictine) mustelids, and the mustelid Eira, three groups that likely had dispersal abilities similar to those of procyonids but did not disperse to South America until much later, after the last record of sparassodonts. Canids and ictonychines in particular are the earliest known carnivorans in South America aside from Cyonasua-group procyonids (with the earliest records dating to ~2.9 Ma; Prevosti and Forasiepi Reference Prevosti and Forasiepi2018) and are some of the few groups of carnivorans known to make successful dispersals over water (van der Geer et al. Reference Van der Geer, Vos de and Dermitzakis2011). Procyonids plot outside sparassodont morphospace; whereas canids, ictonychines, and Eira plot within sparassodont morphospace, as would be expected if ecological dissimilarity was a factor in successful colonization.
The early appearance of procyonids in South America is probably the result of several factors. Occupation of an ecological niche distinct from those occupied by incumbent South American mammals may have been the most important factor, but several other aspects of procyonid ecology may have favored over-water dispersal. We find no evidence for significant competition between Cyonasua-group procyonids and endemic South American carnivorous mammals such as sparassodonts, in support of other studies that have suggested that the extinction of sparassodonts was due to other factors (e.g., Marshall Reference Marshall, Hecht, Goody and Hecht1977; Forasiepi et al. Reference Forasiepi, Martinelli and Goin2007; Prevosti et al. Reference Prevosti, Forasiepi and Zimicz2013; Engelman and Croft Reference Engelman and Croft2014; Zimicz Reference Zimicz2014; López-Aguirre et al. Reference López-Aguirre, Archer, Hand and Laffan2017; Croft et al. Reference Croft, Engelman, Dolgushina and Wesley2018; Prevosti and Forasiepi Reference Prevosti and Forasiepi2018). The successful establishment of procyonids in South America was undoubtedly partly due to luck but perhaps principally due to the simple fact that these animals were entering a previously unoccupied ecological niche.
Acknowledgments
We thank L. H. Soibelzon for pictures of the lower dentition of Chapalmalania; M. J. Babot for pictures of Patene; R. M. D. Beck for useful discussions; J. Galkin and J. Meng (AMNH), W. Simpson and K. Angielczyk (FMNH), and M. Reguero (MLP) for access to specimens in their care; R. McCord (Arizona Museum of Natural History) for loans from the Larry Marshall Marsupial Dentition Collection; and J. Tarquini and two anonymous reviewers for comments that greatly improved this article.