1. Introduction
Research has shown that when bilingual speakers switch from one language to the other, it incurs a processing cost. For instance, it takes longer to switch between languages than to repeat the same language in a picture-naming task (e.g., Meuter & Allport, Reference Meuter and Allport1999). It is understood that the switching cost in bilinguals is the result of the language selection process, in which the operating language control system (i.e., inhibitory control; Green, Reference Green1998) prevents transient, trial-to-trial cross-language interference (for reviews, see Declerck & Philipp, Reference Declerck and Philipp2015; Kiesel et al., Reference Kiesel, Steinhauser, Wendt, Falkenstein, Jost, Philipp and Koch2010). Neuroimaging studies have shown the engagement of executive function networks, such as prefrontal cortex, anterior cingulate cortex, basal ganglia and inferior parietal lobule, in language switching (e.g., Abutalebi et al., Reference Abutalebi, Annoni, Zimine, Pegna, Seghier, Lee-Jahnke, Lazeyras, Cappa and Khateb2008, Reference Abutalebi, Della Rosa, Ding, Weekes, Costa and Green2013; de Bruin et al., Reference de Bruin, Roelofs, Dijkstra and FitzPatrick2014; Garbin et al., Reference Garbin, Costa, Sanjuan, Forn, Rodriguez-Pujadas, Ventura, Belloch, Hernandez and Ávila2011; Guo et al., Reference Guo, Liu, Misra and Kroll2011; Hernandez et al., Reference Hernandez, Dapretto, Mazziotta and Bookheimer2001; Hosoda et al., Reference Hosoda, Hanakawa, Nariai, Ohno and Honda2012; Wang et al., Reference Wang, Xue, Chen, Xue and Dong2007), indicating the involvement of domain-general cognitive control in language control (for reviews, see Abutalebi & Green, Reference Abutalebi and Green2007, Reference Abutalebi and Green2008, Reference Abutalebi and Green2016; Green & Abutalebi, Reference Green and Abutalebi2013).
However, existing studies have mostly focused on forced language selection, a process driven by external demands rather than the volition of the bilingual speaker. In experimental contexts, forced language selection is typically examined with paradigms involving artificial language cues (e.g., colours or shapes) that are arbitrarily associated with languages (Abutalebi & Green, Reference Abutalebi and Green2007, Reference Abutalebi and Green2008, Reference Abutalebi and Green2016; Green & Abutalebi, Reference Green and Abutalebi2013). Nonetheless, forced language selection might be an intrinsically different process from free language selection. In a seminal study, Gollan and Ferreira (Reference Gollan and Ferreira2009) showed that switching costs could be the result of the “forced nature” (i.e., cue-directed) of the experimental task. Several studies have found that switching costs are reduced or even vanished when bilingual speakers are free (or relatively free) to select the languages to speak (e.g., Blanco-Elorrieta & Pylkkänen, Reference Blanco-Elorrieta and Pylkkänen2017; de Bruin et al., Reference de Bruin, Samuel and Duñabeitia2018; Gollan & Ferreira, Reference Gollan and Ferreira2009; Gollan et al., Reference Gollan, Kleinman and Wierenga2014; Gross & Kaushanskaya, Reference Gross and Kaushanskaya2015; Jevtović et al., Reference Jevtović, Duñabeitia and de Bruin2020; Jiao et al., Reference Jiao, Gao, Schwieter, Li, Zhu and Liu2022; Kleinman & Gollan, Reference Kleinman and Gollan2016; Reverberi et al., Reference Reverberi, Kuhlen, Seyed-Allaei, Greulich, Costa, Abutalebi and Haynes2018; Zhang et al., Reference Zhang, Wang, Huang, Li, Qiu, Shen and Xie2015; Zhu et al., Reference Zhu, Blanco-Elorrieta, Sun, Szakay and Sowman2022).
To date, only a few neuroimaging studies have examined the neural mechanisms underlying language control when bilinguals are free to select the language (for functional magnetic resonance imaging [fMRI] studies, see Reverberi et al., Reference Reverberi, Kuhlen, Seyed-Allaei, Greulich, Costa, Abutalebi and Haynes2018; Zhang et al., Reference Zhang, Wang, Huang, Li, Qiu, Shen and Xie2015; for magnetoencephalography [MEG] studies, see Blanco-Elorrieta & Pylkkänen, Reference Blanco-Elorrieta and Pylkkänen2017; Zhu et al., Reference Zhu, Blanco-Elorrieta, Sun, Szakay and Sowman2022). These studies have taken different aspects of free language selection as regions of interest, focusing on a plausible decomposition of language control processes, including goal maintenance, conflict monitoring, interference suppression, salient cue detection, selective response inhibition, task disengagement and engagement and opportunistic planning (Green & Abutalebi, Reference Green and Abutalebi2013). For example, Reverberi et al. (Reference Reverberi, Kuhlen, Seyed-Allaei, Greulich, Costa, Abutalebi and Haynes2018) teased apart language selection and language execution with a delayed naming task in which German–English bilinguals freely chose the naming language. This design had the merit of temporally differentiating the intention to speak a language from actually producing that language. Results showed that language selection (i.e., the intention to use a language) involved the domain-general cognitive control network while language execution relied on language-specific control network. Taking the advantage of high temporal resolution of MEG, Blanco-Elorrieta and Pylkkänen (Reference Blanco-Elorrieta and Pylkkänen2017) examined language selection in highly ecological experimental settings. In the bilingual interlocutor context, bilinguals were completely free to choose the output language upon the presentation of a face cue (i.e., a face of a bilingual speaker without an obvious language preference). In the monolingual interlocutor context, bilinguals had to choose the language that matched a face cue (i.e., a face of a monolingual speaker). In the coloured cue context, bilinguals were forced to name pictures in the language corresponding to a colour cue. Increased activations in prefrontal areas and behavioural effects of switching cost were observed in the coloured cue context whereas the effect was absent in the interlocutor contexts, suggesting a dissociation in the functional status of the language control system between free and forced language selection.
Previous studies on language switching showed that the superior frontal gyrus/supplementary motor area (SMA) is involved in suppressing the non-target language (Guo et al., Reference Guo, Liu, Misra and Kroll2011; Rodriguez-Fornells et al., Reference Rodriguez-Fornells, Lugt, Rotte, Britti, Heinze and Münte2005) and lexical access in the target language (Alario et al., Reference Alario, Chainay, Lehericy and Cohen2006; Van Heuven et al., Reference Van Heuven, Schriefers, Dijkstra and Hagoort2008). In the “what, when, whether” model of volitional behaviour, Brass and Haggard (Reference Brass and Haggard2008) suggest that the pre-SMA/SMA is responsible for determining the timing of an action's execution, critical in the planning of self-initiated behaviours (Lau et al., Reference Lau, Rogers, Haggard and Passingham2004). Studies have established the parietal cortex as a key region in language switching (for reviews, see Abutalebi & Green, Reference Abutalebi and Green2007; Green & Abutalebi, Reference Green and Abutalebi2013), but more specifically, it has been associated with the development of intentions (e.g., Desmurget et al., Reference Desmurget, Reilly, Richard, Szathmari, Mottolese and Sirigu2009; Lau et al., Reference Lau, Rogers, Haggard and Passingham2004; Soon et al., Reference Soon, Brass, Heinze and Haynes2008). The caudate nucleus is a well-established subcortical structure in language control (Abutalebi & Green, Reference Abutalebi and Green2007; Green & Abutalebi, Reference Green and Abutalebi2013). Activation in the caudate has been associated with a collection of cognitive functions in the management of bilingual's two languages (Crinion et al., Reference Crinion, Turner, Grogan, Hanakawa, Noppeney, Devlin, Aso, Urayama, Fukuyama and Stockton2006; Green & Abutalebi, Reference Green and Abutalebi2013). It is proposed that, as part of the basal ganglia, the caudate nucleus gates the link between prefrontal cortex and posterior cortical regions.
While existing evidence has revealed that language selection, as a volitional act (MacIntyre, Reference MacIntyre2007; Schmidt, Reference Schmidt2014), recruits distinctive neural mechanisms between free and forced language contexts, previous studies have almost exclusively relied on the use of language cues to either directly (e.g., colours) or indirectly (e.g., faces or national flags) indicate to the bilingual participants the target language of the experiment. However, performance in cue-directed language tasks is, in essence, externally guided language behaviour, which involves cue detection and selective response inhibition, cognitive processes that are absent or less prominent in truly free language behaviour (Blanco-Elorrieta & Pylkkänen, Reference Blanco-Elorrieta and Pylkkänen2017; Zhang et al., Reference Zhang, Wang, Huang, Li, Qiu, Shen and Xie2015; Zhu et al., Reference Zhu, Blanco-Elorrieta, Sun, Szakay and Sowman2022). Therefore, experiments adopting an event-related design by using language cues cannot fully establish a free or voluntary language selection context, although the “forced-nature” of the experiment has been reduced with more ecological cues (e.g., faces).
The purpose of the present study is to examine how voluntariness (forced or free) affects language selection in bilinguals and the underlying neural mechanisms that are associated with the effects. The present study adopts a block design, in which the language(s) used in each block was predetermined by the instructions rather than using language cues. In each trial, two pictures of common objects were presented one after the other. In the non-switch block, Chinese–English bilingual participants named the two pictures with the same language. In the forced-switch block, the naming language altered between the first and the second picture. In the free-naming block, the participants determined the naming language(s) on their own will, but they were instructed to try to use both languages (i.e., either switch or non-switch) in a random order (Arrington & Logan, Reference Arrington and Logan2004; Zhang et al., Reference Zhang, Wang, Huang, Li, Qiu, Shen and Xie2015). To increase the comparableness of the language selection process in the three conditions, the naming language for the first picture was predetermined in all conditions.
We are particularly interested in the prefrontal cognitive control regions, such as the dorso-lateral prefrontal cortex (e.g., Abutalebi et al., Reference Abutalebi, Annoni, Zimine, Pegna, Seghier, Lee-Jahnke, Lazeyras, Cappa and Khateb2008, Reference Abutalebi, Della Rosa, Ding, Weekes, Costa and Green2013; de Bruin et al., Reference de Bruin, Roelofs, Dijkstra and FitzPatrick2014; Garbin et al., Reference Garbin, Costa, Sanjuan, Forn, Rodriguez-Pujadas, Ventura, Belloch, Hernandez and Ávila2011; Guo et al., Reference Guo, Liu, Misra and Kroll2011; Hernandez et al., Reference Hernandez, Dapretto, Mazziotta and Bookheimer2001; Hosoda et al., Reference Hosoda, Hanakawa, Nariai, Ohno and Honda2012; Wang et al., Reference Wang, Xue, Chen, Xue and Dong2007, Reference Wang, Kuhl, Chen and Dong2009; Zhang et al., Reference Zhang, Wang, Huang, Li, Qiu, Shen and Xie2015, Reference Zhang, Wang, Yue, Gao, Huang, Wang, Wen, Qiu and Wu2019), because these brain areas have been shown to be sensitive in language switching tasks. We also expected that free naming, as compared to forced naming conditions (i.e., forced-switch and non-switch), would activate brain regions associated with naming volition, such as middle frontal cortex and medial prefrontal cortex (e.g., Demanet et al., Reference Demanet, De Baene, Arrington and Brass2013; Forstmann et al., Reference Forstmann, Brass, Koch and Von Cramon2006; Orr & Banich, Reference Orr and Banich2014; Wisniewski et al., Reference Wisniewski, Goschke and Haynes2016). Moreover, to differentiate language switching from meaning switching (Abutalebi et al., Reference Abutalebi, Annoni, Zimine, Pegna, Seghier, Lee-Jahnke, Lazeyras, Cappa and Khateb2008; Hernandez et al., Reference Hernandez, Dapretto, Mazziotta and Bookheimer2001; Zhang et al., Reference Zhang, Wang, Yue, Gao, Huang, Wang, Wen, Qiu and Wu2019), the present study also manipulated the repetition of pictures within each trial (i.e., picture-repeat or picture-switch conditions) orthogonally to the naming language. We predicted the involvement of the prefrontal cortex in meaning switching, based on findings of previous studies on bilingual speech production (Abutalebi et al., Reference Abutalebi, Annoni, Zimine, Pegna, Seghier, Lee-Jahnke, Lazeyras, Cappa and Khateb2008; Hernandez et al., Reference Hernandez, Dapretto, Mazziotta and Bookheimer2001; Zhang et al., Reference Zhang, Wang, Yue, Gao, Huang, Wang, Wen, Qiu and Wu2019) and comprehension (Chee et al., Reference Chee, Soon and Lee2003; Crinion et al., Reference Crinion, Turner, Grogan, Hanakawa, Noppeney, Devlin, Aso, Urayama, Fukuyama and Stockton2006; Klein et al., Reference Klein, Zatorre, Chen, Milner, Crane, Belin and Bouffard2006).
2. Methods
2.1. Participants
Twenty-nine Chinese–English bilingual students from the Southwest University of China participated in the experiment. All participants were right-handed and had normal or corrected-to-normal vision. Participants gave written consent and received payment for their participation. No participant had a history of neurological or psychiatric disorders. The experiment was approved by the Research Ethical Committee of Southwest University. Data from four participants were excluded from fMRI analyses due to excessive head motion inside an MRI scanner (>3 mm of maximum displacement and 3° of angular motion). The final data consisted of 25 participants (14 females) aged from 18 to 25 years (M = 20.2, SD = 2.1).
All participants were bilinguals with Chinese as L1 and English as L2. Participants were educated in China and learned English in school. A modified version of the Language Experience and Proficiency Questionnaire (Marian et al., Reference Marian, Blumenfeld and Kaushanskaya2007) was used to assess participants' language use and proficiency level. Table 1 shows that there was a significant difference between Chinese and English on all measurements (all ps <.001), suggesting that the participants were unbalanced bilinguals.
Table 1. Assessment of language proficiency, exposure and daily use of Chinese and English
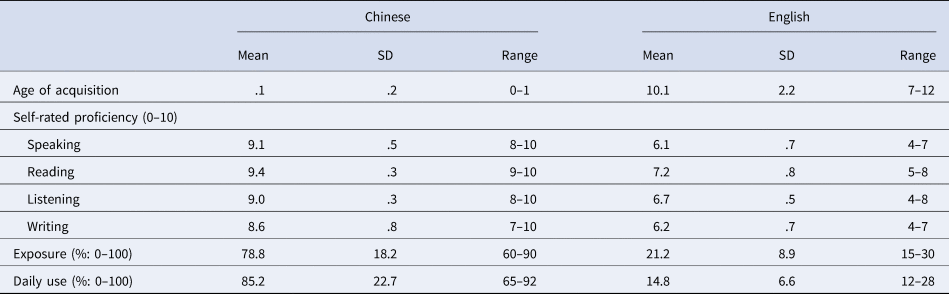
2.2. Materials
Thirty-six black-and-white line drawings (Zhang et al., Reference Zhang, Wang, Yue, Gao, Huang, Wang, Wen, Qiu and Wu2019) were selected from the database of Snodgrass and Vanderwart (Reference Snodgrass and Vanderwart1980). The pictures depicted frequently encountered, concrete objects. Picture names from the same semantic categories were kept at least five trials in between to avoid the cumulative semantic interference effect (Costa et al., Reference Costa, Strijkers, Martin and Thierry2009).
2.3. Design
The experiment employed an overt picture-naming task which participants performed over three separate sessions. In each session, there were three language selection conditions (i.e., non-switch, forced-switch and free-naming) which were presented in blocks, the order of which was counterbalanced across participants. Within each block, the naming language (i.e., Chinese and/or English) and switching directions (L1–L2 and L2–L1, if there were language switches) were counterbalanced across 4 mini-blocks of 12 experimental trials. Each trial started with a 200-ms fixation cross, and a picture (picture 1) was presented for 800 ms followed by another 200-ms fixation cross. The second picture (picture 2) was then presented for 800 ms. The inter-stimuli-interval was a blank screen of 2000 ms. Picture 2 was either identical to (the picture-repeat condition) or different from (the picture-switch condition) picture 1. Therefore, the study conforms to a 2 (meaning switching: picture-repeat, picture-switch) × 3 (language selection: non-switch, forced-switch, free-naming) within-subject design, with a total number of 432 trials (i.e., 72 trials in each condition).
2.4. Procedure
In the beginning of the experiment were practice trials with eight pictures that were not used for the rest of the experiment. The practice trials included all conditions to familiarize participants with the task. After the practice trials, the experiment started following a block order that was counterbalanced across participants within each session. In the beginning of each block, an instruction was presented on the screen. In the forced-switch condition, participants were instructed to name picture 2 with the different language from picture 1. In the non-switch condition, participants were instructed to name picture 2 with the same language as picture 1. In the free-naming condition, participants were instructed to name picture 2 either with the same or the different language as picture 1 at the participants' own will. However, to avoid participants always naming both pictures with the same language (i.e., non-switch) or with one language (e.g., L1) much more often than the other language, as found in our pilot study, the instruction specified to “try to use both languages equally often and switch in a random order” (Arrington & Logan, Reference Arrington and Logan2004; Zhang et al., Reference Zhang, Wang, Huang, Li, Qiu, Shen and Xie2015). Within the block, each mini-block started with one pair of pictures presented one after another as an exemplar, showing participants the naming language of picture 1 in that mini-block. Participants were instructed to name the picture accurately and quickly but in a low voice with minimal head movement (Heim et al., Reference Heim, Amunts, Mohlberg, Wilms and Friederici2006). Participants were informed that their naming would be recorded via an interphone device to encourage optimal performance, but in fact the oral responses could not be recorded from inside the scanner due to technical constraints (e.g., Abutalebi et al., Reference Abutalebi, Annoni, Zimine, Pegna, Seghier, Lee-Jahnke, Lazeyras, Cappa and Khateb2008). To acquire the behavioural data, participants repeated the identical experimental procedure outside the scanner in a dimly lit room 30 min after the scanning (Abutalebi et al., Reference Abutalebi, Annoni, Zimine, Pegna, Seghier, Lee-Jahnke, Lazeyras, Cappa and Khateb2008; Guo et al., Reference Guo, Liu, Misra and Kroll2011; Wang et al., Reference Wang, Xue, Chen, Xue and Dong2007, Reference Wang, Kuhl, Chen and Dong2009; Zhang et al., Reference Zhang, Wang, Huang, Li, Qiu, Shen and Xie2015, Reference Zhang, Wang, Yue, Gao, Huang, Wang, Wen, Qiu and Wu2019). There was a 12-s break between mini-blocks, a 60-s break between blocks and a 3-min break between sessions. All data collection was carried out on the same day.
2.5. fMRI data acquisition and analysis
fMRI scanning was performed with a Siemens Trio 3-Tesla scanner using a 12-channel transmit/receive gradient head coil. In each block, a T2*-weighted gradient-echo planar imaging (EPI) sequence was acquired (TR, 2000 ms; TE, 30 ms; FOV, 64 × 64 mm2; 32 interleaved descending slices; flip angle, 90°; voxel size, 3.44 × 3.44 × 3.00 mm3 with a 1-mm intra-slice gap). The anatomical images were acquired using a T1-weighted three-dimensional gradient-echo sequence (TR, 1900 ms; TE, 2.52 ms; flip angle, 9°; FOV, 256 × 256 mm2; voxel size, 1 × 1 × 1 mm3). Image processing and statistical analysis were performed using SPM 12 (Wellcome Department of Imaging Neuroscience, Institute of Neurology, London, UK) and Matlab 2015a. For each participant, the EPI time series data underwent realignment, slice timing correction, anatomic-functional image co-registration, segmentation, normalization and smoothing with an isotropic 6-mm full-width-half-maximum Gaussian kernel to increase the signal-to-noise ratio. The preprocessed functional images were then subjected to fixed-effects analyses for each participant using a general linear model (GLM) on a voxel-by-voxel basis across the whole brain. We used a blocked rather than event-related approach to the data analysis: instead of performing comparisons on each trial, we compared linear derivation of the haemodynamic responses between the non-switch, forced-switch and free-naming blocks, which were systematically different only in the language selection contexts. Regressors were constructed in the GLM from six combinations of the following factors: meaning switching (picture-repeat and picture-switch) and language selection (non-switch, forced-switch and free-naming). The blood-oxygen-level-dependent (BOLD) response for each regressor was modelled with the canonical haemodynamic response function and time derivative. The motion correction parameters were entered as confound regressors in the first-level, single-subject design matrices to correct for potential movement artefacts.
The group-averaged effects were computed with a random-effects model. For group analysis, only clusters larger than 30 voxels activated above a threshold of p < .001 (false-discovery rate [FDR], FDR-corrected for multiple comparisons) were considered significant (e.g., Zhou et al., Reference Zhou, Zhao, Qi, Geng, Yao, Kendrick, Wager and Becker2021). The images in all whole-brain analyses derived from the first-level analyses were entered into the model in a subject-wise fashion. Firstly, to examine the brain mechanisms underlying the meaning switching and language selection context, we computed the main effects of the two variables and their interaction. Since no interaction was found, we then collapsed picture-repeat and picture-switch conditions in the following analyses. To further examine effects of language selection context, we contrasted both the forced-switch and free-naming conditions against the non-switch condition which was considered as the baseline control condition. Then, we performed both a contrast analysis and a conjunction analysis with the outcomes of the above contrasts (p < .001 uncorrected with an extent threshold of 50 continuous voxels applied to all contrasts) to reveal the distinct and overlapping brain regions between forced and free language selection contexts. To examine the meaning switching effect, we contrasted the picture-switch against picture-repeat condition.
However, there might exist a confound in switching frequency when directly comparing forced against free language selection contexts, because the forced switching block involves 100% language switching, the free-naming block involves approximately 50% language switching (as in the instructions) and the non-switch block involves no language switching. To address this difference, we also took a different approach to fMRI data analysis: we first performed a conjunction analysis between the forced-switch and non-switch conditions to specify the neural mechanisms associated with forced language selection independent of language switching. We then contrasted the conjunction analysis results with the free-naming condition which also contains a relatively balanced switching and non-switch trails. The results, therefore, dissociated how forced and free language selection contexts affect language control in bilinguals, while minimizing the influences of language switching frequency.
3. Results
3.1. Behavioural results
Only the naming latency of picture 2 in each trial was included for behavioural analysis (Zhang et al., Reference Zhang, Wang, Yue, Gao, Huang, Wang, Wen, Qiu and Wu2019). Excluded from analysis were naming latencies of incorrect trials, including: (A) when there was no response; (B) when the naming language or the naming word was wrong; (C) when there were voice key errors such as hesitation voice and half-way changes of naming and (D) when the response latencies were three standard derivations above or below the average for each condition. Additionally, in the post-experiment verification procedure, the participants were found to choose to switch between languages in 45.8% of the trials in the free-naming condition. Within the 45.8% language switching trials, 58.4% were switching from English into Chinese and 41.6% were switching from Chinese into English. As the error rate was only 2.01%, accuracy was not analysed further.
A two-way repeated measures analysis of variance on the naming latencies was conducted with meaning switching (picture-repeat and picture-switch) and language selection contexts (non-switch, forced-switch and free-naming) as within-subject variables (see Table 2). The main effect of meaning switching was significant [F (1, 24) = 417.99, p < .001, η 2 = .946] with the picture-switch (M = 922, SD = 112) slower than the picture-repeat (M = 302, SD = 111), indicating meaning switching effects. The main effect of language selection context was also significant [F (1, 24) = 9.67, p = .001, η 2 = .457] with the free-naming (M = 631, SD = 87) and forced-switch (M = 620, SD = 89) condition being slower than the non-switch (M = 584, SD = 84) condition, indicating classic switching cost effects, but there was no significant difference between the two effects (47 ms versus 36 ms; p > .05). Moreover, there was no interaction between language selection and meaning switching [F < 1].
Table 2. Reaction times (RTs) in millisecond (ms) and error rates in percentage (%) as a function of language selection (non-switch, forced-switch and free-naming) and meaning switching (picture-repeat and picture-switch)
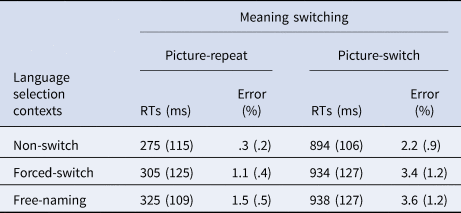
Standard deviations (SD) in parentheses.
3.2. Neuroimaging results
3.2.1. Contrast analyses
Functional neuroimaging analyses showed main effects of meaning switching and language selection context with no interaction between the two factors. As compared to the non-switch condition, both forced-switch and free-naming conditions showed a pattern of bilateral activity in the fronto-parietal–caudate regions. Specifically, contrast analysis between the forced-switch and non-switch conditions showed bilateral activations in the middle frontal gyri (BA 6/9/32), inferior parietal lobules (BA 40) and caudate tail (see Figure 1A and Table S1a). The contrast analysis between free-naming and non-switch conditions showed bilateral activity in the middle frontal gyri (BA 6/9/10/32), inferior parietal lobules (BA 40) and caudate tail. Moreover, there were also bilateral activations in the fronto-parietal regions which extended into the superior frontal gyri (BA 8), superior parietal lobules (BA 7) and the left lateralized activations in inferior frontal gyri (BA 47; see Figure 1B and Table S1b) in the contrast analysis between free-naming and non-switch conditions. Finally, the contrast analysis between forced and free language selection contexts revealed activations in the middle/medial frontal gyri bilaterally (BA 8/9/10), the left superior temporal gyrus (BA 42) and the right insula (BA 13; see Figure 1C and Table S1c). The forced language selection context was established by the contrast analysis between forced-switch and non-switch conditions. The free language selection context was established by the contrast analysis between free-naming and non-switch conditions.
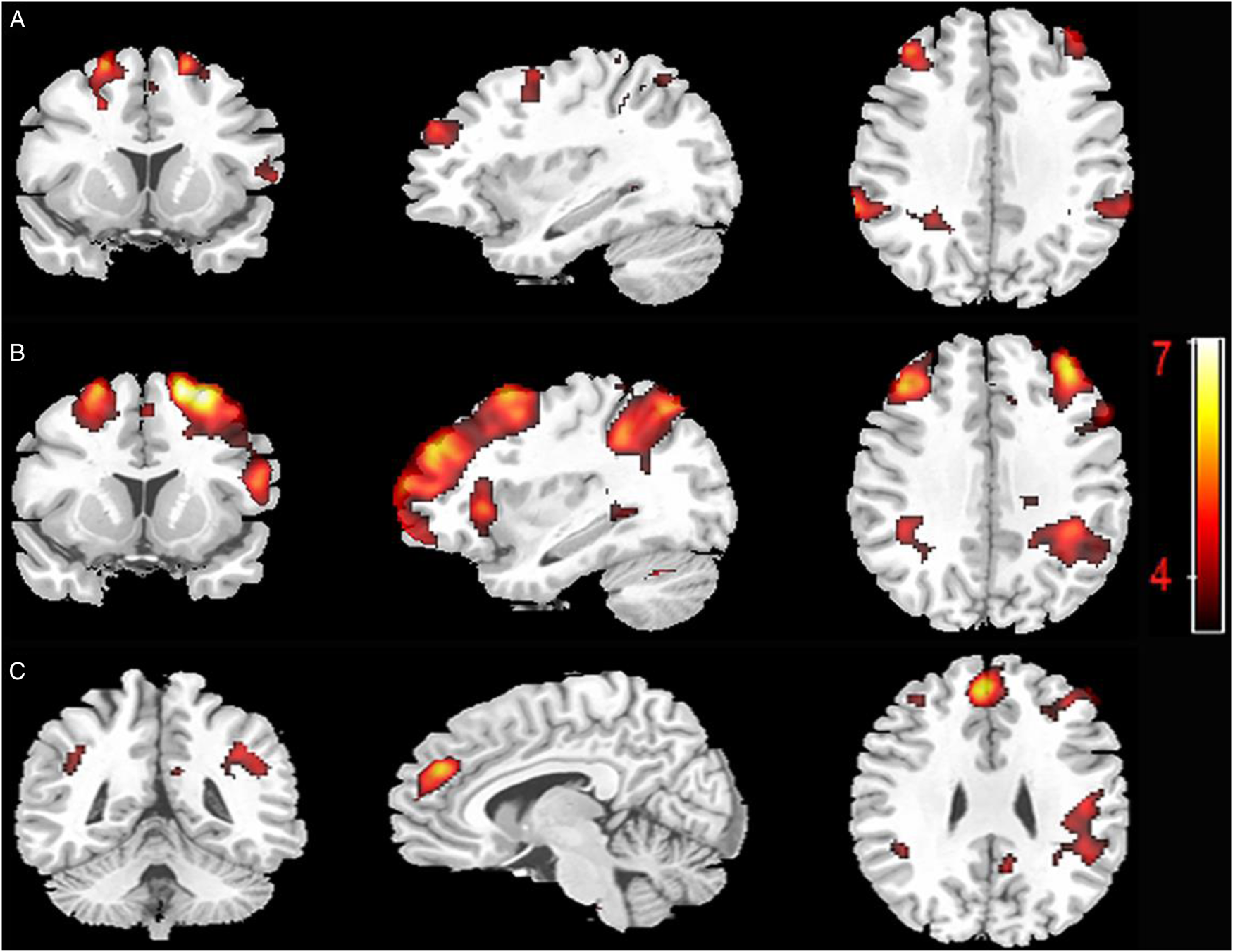
Figure 1. Brain activation maps of the contrast analysis results of (A) forced-switch versus non-switch, (B) free-naming versus non-switch and (C) forced language selection (the outcome of A) versus free language selection (the outcome of B).
The contrast analysis between the picture-switch and picture-repeat conditions showed an extensive pattern of bilateral activity in the middle occipital gyri (BA 18/19), fusiform gyri (BA 37), superior parietal lobules (BA 7), middle frontal gyri (BA 6) and caudate tails (see Figure 2 and Table S2), revealing a meaning switching effect that was partially distinguished (i.e., the middle occipital gyri and fusiform gyri) from the language switching effect.
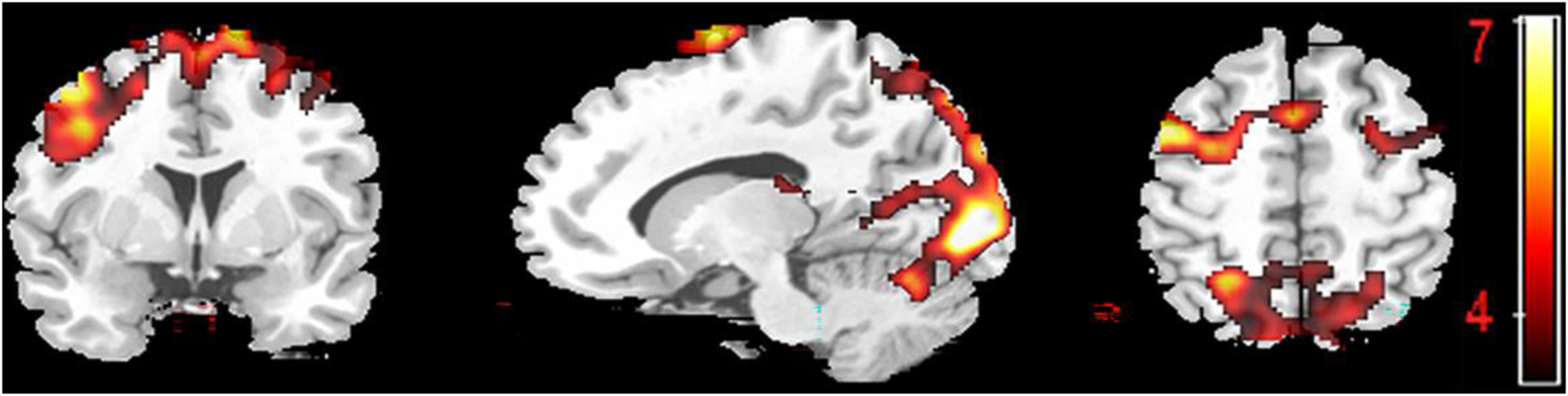
Figure 2. Brain activation maps of meaning switching as revealed by the contrast analysis of the picture-repeat versus picture-switch condition.
3.2.2. Conjunction analyses
To reveal the neural mechanisms associated with language switching, a conjunction analysis was performed between forced language selection context (as established by the contrast analysis between forced-switch versus non-switch) and free-switching (as established by the contrast analysis between free-naming versus non-switch condition). The results showed bilateral activations of the middle frontal gyri (BA 6/9/10), cingulate gyri (BA 32), superior/inferior parietal lobule (BA 7/40) and caudate tail, and activity in the right inferior frontal gyrus (BA 44; see Figure 3A and Table S3a).
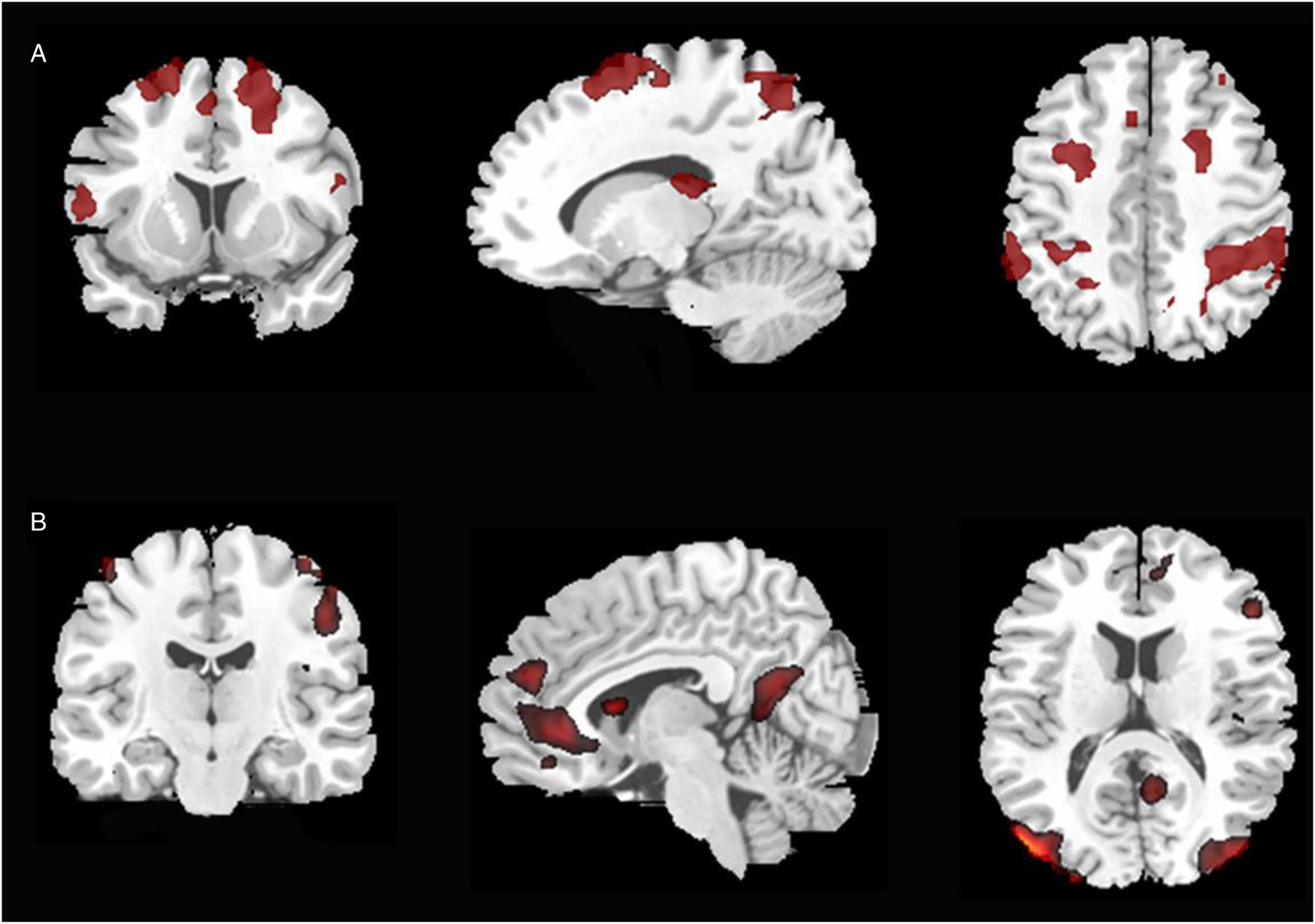
Figure 3. Patterns of brain activations as revealed by the conjunction analyses between (A) forced-switching and free-switching and (B) forced-switch and non-switch conditions.
The conjunction analysis between the forced-switch and non-switch conditions was performed to reveal neural mechanisms associated with forced language selection. The results showed left-lateralized activations within frontal gyrus regions (see Figure 3B and Table S3b), including the medial frontal gyrus (BA 9/11), inferior frontal gyrus (BA 47) and middle frontal gyri (BA 11). In addition, the left cingulate cortex (BA 31/32) and the bilateral middle occipital gyri (BA 19/37) were also found to be activated.
To determine the effect of voluntariness on the language control system, the free language selection context (i.e., the free-naming condition) was contrasted against the forced language selection condition (i.e., conjunction analysis between the forced-switch and non-switch conditions). The results revealed extensive activations in the bilateral frontal cortex, encompassing the medial (BA 6/8), middle (BA 9/10) and superior frontal gyrus (BA 6). The right inferior parietal lobule (BA 40) was also activated. Moreover, bilateral caudate was found to be activated, indicating that the fronto-parietal–caudate network is more engaged when in free as compared to forced language selection context (see Figure 4 and Table S4).
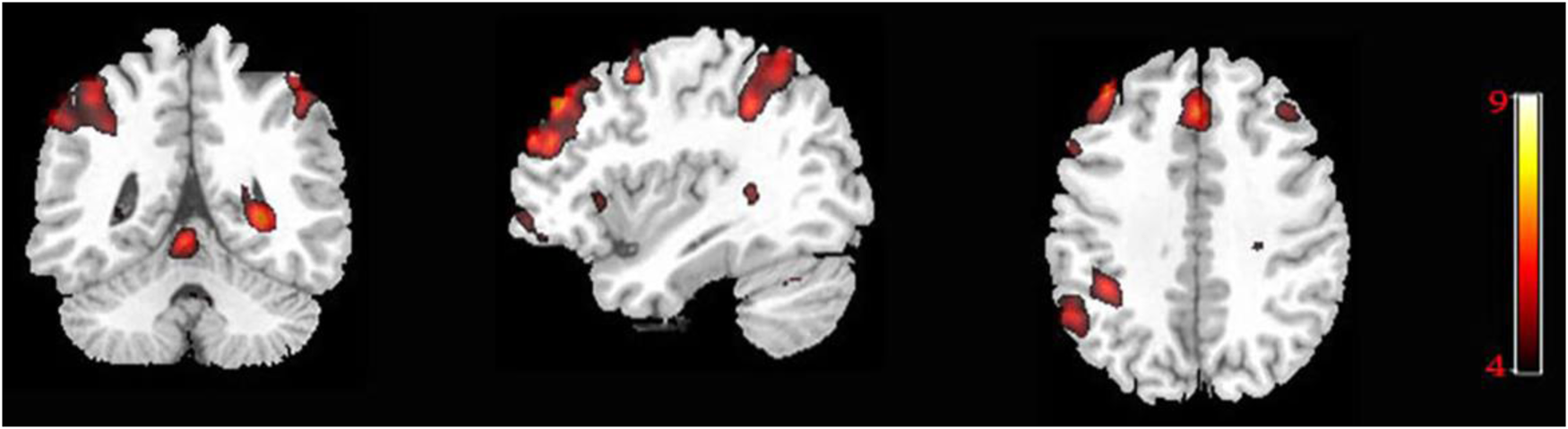
Figure 4. Brain activation maps as revealed by the contrast analysis between free and forced language selection contexts.
4. Discussion
The present study investigates the effect of language selection contexts (e.g., free or forced) on the neural dynamics of language control during bilingual speech production. To prevent the potential artificial influences of using language cues, Chinese–English bilinguals were examined in a cue-void language switching task with a block, rather than event-related, design. The three blocks were non-switch, forced-switch and free-naming blocks, tapping respectively onto forced and free language selection contexts with both language switching and non-switch. Behavioural results showed that both free and forced language switching incurred a switching cost when compared to the non-switch condition. However, there were no significant differences in the switching cost magnitude between the free and forced language selection contexts. Costa and Santesteban (Reference Costa and Santesteban2004) argued that language switching requires higher inhibitory control in unbalanced as compared to balanced bilinguals. The proposal is supported by neuroimaging evidence showing that L2 proficiency modulates the activation levels of the language control network in bilinguals (e.g., Abutalebi et al., Reference Abutalebi, Della Rosa, Ding, Weekes, Costa and Green2013; Mouthon et al., Reference Mouthon, Khateb, Lazeyras, Pegna, Lee-Jahnke, Lehr and Annoni2020). Therefore, the fact that switch cost effects were found in both free- and forced-naming conditions in the present study could be due to unbalanced language proficiency in the bilingual participants (Zhang et al., Reference Zhang, Wang, Huang, Li, Qiu, Shen and Xie2015). Previous studies on balanced bilinguals showed an absence of switching effect in the free-naming condition (Blanco-Elorrieta & Pylkkänen, Reference Blanco-Elorrieta and Pylkkänen2017; Zhu et al., Reference Zhu, Blanco-Elorrieta, Sun, Szakay and Sowman2022).
In order to yield an approximate 50% switching rate in the free-naming condition, participants were instructed to “try to use two languages equally often and in a random order” (Arrington & Logan, Reference Arrington and Logan2004; Zhang et al., Reference Zhang, Wang, Huang, Li, Qiu, Shen and Xie2015). A switching rate of 46% was observed in the present study, similar to studies on unbalanced bilinguals (de Bruin & Xu, Reference de Bruin and Xu2023; Liu et al., Reference Liu, Timmer, Jiao, Yuan and Wang2019; Mooijman et al., Reference Mooijman, Schoonen, Ruiter and Roelofs2023; Sánchez et al., Reference Sánchez, Struys and Declerck2022) but higher as compared to studies examining free language switching in balanced bilinguals (Blanco-Elorrieta & Pylkkänen, Reference Blanco-Elorrieta and Pylkkänen2017; de Bruin et al., Reference de Bruin, Samuel and Duñabeitia2018, Reference de Bruin, Samuel and Duñabeitia2020; Grunden et al., Reference Grunden, Piazza, García-Sánchez and Calabria2020; Jevtović et al., Reference Jevtović, Duñabeitia and de Bruin2020). Interestingly, Gollan and Ferreira (Reference Gollan and Ferreira2009) showed that when the instructions of free picture-naming is to use “whatever language comes to mind”, bilinguals switch less often than when the instruction is “using each language about half the time” for the same task, indicating that the specific instruction plays a critical role in how frequent bilingual participants switch between languages in a free-naming task. According to Gollan and Ferreira (Reference Gollan and Ferreira2009), bilingual participants may develop a strategy to name relatively easy items in their non-dominant language and more difficult items in the dominate language, an effect referred to as the “item switching effects”. The stimuli used in the present study were high-frequency concrete words and they were repeated across conditions, in which the observed error rates were low (i.e., .3–3.6%), indicating that the influences of an experimental strategy (i.e., item switching effects) on language switching is highly unlikely in the present study.
However, it is important to note that, the instructions to use Chinese and English equally often and switch on a random basis might have reduced the extent to which the free-naming condition was voluntary (i.e., quasivoluntary language switching; Gollan & Ferreira, Reference Gollan and Ferreira2009), because it is conceivable that unbalanced bilinguals would use the dominant language more often if they were fully free to select the naming language. Moreover, the instruction might have increased the memory load of the task, as participants had to keep in mind the approximate number of trials named with each of the two languages. Therefore, it is possible that the requirement to use each language about half the time leads to the switching costs at the behavioural level in the free-naming condition, because the instruction reduced voluntariness and increased memory load.
Analysis of fMRI results dissociated the neural mechanisms underlying bilingual language control in free as compared to forced language selection contexts. This was achieved by first contrasting both forced-switching (see Table S1a) and contrasting free-naming against non-switch condition (see Table S1b), and then contrasting the outcome of these analysis. The results showed activations in the bilateral middle frontal gyri, the left medial frontal gyrus, the left temporal gyrus and the right insula. Previous studies comparing internally with externally guided linguistic tasks have shown robust activations in the dorsolateral and ventrolateral prefrontal cortex, and medial frontal cortex (MFC) in monolinguals (Crosson et al., Reference Crosson, Sadek, Maron, Gökçay, Mohr, Auerbach, Freeman, Leonard and Briggs2001) and bilinguals (Zhang et al., Reference Zhang, Wang, Huang, Li, Qiu, Shen and Xie2015). For instance, using multivariate pattern analyses, Reverberi et al. (Reference Reverberi, Kuhlen, Seyed-Allaei, Greulich, Costa, Abutalebi and Haynes2018) have found increased activation in the medial prefrontal cortex when participants could freely choose which language to use for picture naming. Critically, evidence from non-linguistic tasks have shown that the middle frontal cortex was involved in the voluntary selection between different response alternatives (e.g., Brass et al., Reference Brass, Lynn, Demanet and Rigoni2013; Demanet et al., Reference Demanet, De Baene, Arrington and Brass2013; Orr & Banich, Reference Orr and Banich2014; Walton et al., Reference Walton, Devlin and Rushworth2004; Wisniewski et al., Reference Wisniewski, Goschke and Haynes2016). The engagement of the MFC in voluntary linguistic and non-linguistic tasks suggests that these regions might be associated with cognitive control underlying voluntary acts that are non-specific to language.
To minimize influences of the discrepancy in switching frequency between free-naming (i.e., ≈50% switch rate) and forced-naming conditions (i.e., forced-switch: 100% switch rate; non-switch: 0% switch rate), we also analysed the fMRI data in another approach by first carrying out a conjunction analysis of forced-switch and non-switch conditions. The result of this analysis revealed neural mechanism associated with language control in forced-language selection contexts without the influence of language switching (see Table S3b), because in both conditions the naming language was predetermined, rather than selected at participants' own will, whether there the naming language was switched or not. Then, we contrasted results of the conjunction analysis with the free-naming condition to reveal how free language selection contexts affect language control in bilinguals independent of language switching. Results of this analysis replicated most results found previously featured by increased activations in middle-frontal gyrus in free-naming as compared to forced-naming conditions (see Table S4). However, the new analysis also revealed activations in the right inferior parietal lobule, superior frontal gyrus/SMA and caudate, which were not reported in the previous analysis.
To further specify the neural mechanisms associated with language control, we examined switching effects derived from (a) a change in language and (b) a change in meaning, respectively. The conjunction analysis involving free- and forced-language switching showed activations in bilateral fronto-parietal regions including the dorsolateral prefrontal cortex, premotor cortex, supramarginal gyrus and caudate (see Table S3a). These brain regions have been implicated in cognitive control of both linguistic (for reviews, see Abutalebi & Green, Reference Abutalebi and Green2007, Reference Abutalebi and Green2008, Reference Abutalebi and Green2016; Green & Abutalebi, Reference Green and Abutalebi2013) and non-linguistic tasks that involve code-switching (e.g., Dreher et al., Reference Dreher, Koechlin, Ali and Grafman2002; Rodriguez-Fornells et al., Reference Rodriguez-Fornells, Lugt, Rotte, Britti, Heinze and Münte2005). Given that in both domain-general (e.g., Dosenbach et al., Reference Dosenbach, Visscher, Palmer, Miezin, Wenger, Kang, Burgund, Grimes, Schlaggar and Petersen2006) and domain-specific (e.g., language; Green, Reference Green1998) theories of task switching, it is believed that switching from one task (or language) to another involves inhibition of the previously relevant task-set. Therefore, our results seem to suggest that free language switching might engage the neural mechanism of inhibitory control to a similar extent as forced language switching.
However, the argument that inhibitory mechanism is engaged in free language switching is inconsistent with findings of previous MEG studies, which have failed to find switching costs in free-naming contexts (Blanco-Elorrieta & Pylkkänen, Reference Blanco-Elorrieta and Pylkkänen2017; Zhu et al., Reference Zhu, Blanco-Elorrieta, Sun, Szakay and Sowman2022). Moreover, Blanco-Elorrieta and Pylkkänen (Reference Blanco-Elorrieta and Pylkkänen2017) found that natural, voluntary language switching did not recruit prefrontal regions whereas such brain activity was obtained in forced language switching (Blanco-Elorrieta & Pylkkänen, Reference Blanco-Elorrieta and Pylkkänen2017). Zhu et al. (Reference Zhu, Blanco-Elorrieta, Sun, Szakay and Sowman2022) also observed the engagement of the right inferior frontal gyrus only in the bivalent context, in which bilingual speakers were forced to name pictures in the cue-directed language. This observation disappeared in the natural switching context, in which bilinguals were free to choose the naming language.
Notably, the neural mechanisms underlying bilingual language control is multifaceted and highly adaptive. In the present study, unbalanced bilingual participants were instructed to use both languages equally often and switch on a random basis. This instruction undoubtedly compromised the freedom of language selection in the free-naming condition, because in a truly free-naming context, participants would use any language at any given time without having to keep track of the number and direction of language switching (e.g., Blanco-Elorrieta & Pylkkänen, Reference Blanco-Elorrieta and Pylkkänen2017; de Bruin et al., Reference de Bruin, Samuel and Duñabeitia2018; Jiao et al., Reference Jiao, Gao, Schwieter, Li, Zhu and Liu2022; Zhu et al., Reference Zhu, Blanco-Elorrieta, Sun, Szakay and Sowman2022). Furthermore, the dual-stimuli structure of the naming task also complicated the language selection process, because participants had to distinguish the prime from the target picture in each trial, as there was no visual mark that sets the trials apart. Although there was a 2000-ms break between each trial, it is still possible that the target picture of trial 1 was taken as the prime picture of trial 2 and vice versa. Therefore, the “quasivoluntary” nature of the present study and the specificity of the experimental task combined might explain the behavioural switching costs and associated neural activations observed in the free-naming context, whereas no such observation was made previously. As compared to language switching, meaning switching activated rather restricted areas in the brain (see Table S2), where the observation of bilateral middle occipital gyri/fusiform gyri was specific to meaning switching relative to language switching. Little evidence on bilingual language control has shown the involvement of the occipital gyri/fusiform gyri, but it is well-established that visual attention enhances the processing efficiency of information in the occipital gyrus (e.g., Murray & Wojciulik, Reference Murray and Wojciulik2004). One possibility is that the activations in the occipital gyri/fusiform gyri were associated with visual updates (i.e., picture-switch versus picture-switch) independent of language selection and speech production.
5. Summary
While the language control system has been well studied in bilingual research, how it functions in different language selection contexts (e.g., free versus forced) remains poorly understood. We examined the behavioural and neural correlates of picture-naming in forced-switch, non-switch and free-naming conditions. The observation of switching costs in behavioural performance across different conditions suggests the involvement of inhibitory control in both forced and free language selection. However, forced language selection was associated with left-lateralized frontal activations whereas free language selection was associated with bilateral activations in fronto-parietal regions. This dissociation was confirmed with two, rather than one, approaches to fMRI data analysis, suggesting that forced and free language selection engages separable neural mechanisms. Our speculation is that the neural correlates of forced language selection are responsible for inhibitory control, whereas neural correlates of free language naming are responsible for intention and regulation of self-initiated behaviour. The present study also demonstrated that the specific instruction used in the experiment is critical to the results. Future studies would have determined to what extent the requirement of using both languages equally often in the free-naming task compromise the voluntariness of language selection and affect the functioning of the control system.
Supplementary material
The supplementary material for this article can be found at https://doi.org/10.1017/S1366728924000324.
Data availability statement
The processed data and analysis scripts are available at https://osf.io/kg3tr/?view_only=cc9b903e1cee4468aa24fff734058af3. The raw data can be made available by request to the correspondent author.
Acknowledgements
This study was supported with the funding provided to Y. Z. (21XYY001) and to Y. J. W. (21AYY014) by the National Social Science Foundation of China.
Author contributions
The manuscript has been read and approved by all named authors.
Competing interests
None