1. Introduction
The Cambrian Period is Earth’s first interval with ecosystems dominated by eumetazoans, including diplo- and triploblastic animals, and metabionts (Valentine, Reference Valentine1994; Budd & Jensen, Reference Budd and Jensen2000). The replacement of the earlier Ediacaran biota by clades exhibiting most of life’s current disparity is commonly referred to as the ‘Cambrian Explosion’ (Cloud, Reference Cloud1948; Marshall, Reference Marshall2006). The recognition of this radiation in animals, including changes in their interaction with sedimentary substrates and the appearance of mid-water predation, biomineralization and modular coloniality (Landing et al. Reference Landing, Geyer, Brasier and Bowring2013, Reference Landing, Antcliffe, Geyer, Kouchinsky, Andreas and Bowser2018), had its roots in the 19th century (Darwin, Reference Darwin1859) and matured in the mid to late 20th century (see reviews by Droser et al. Reference Droser, Jensen and Gehling2002; Bengtson, Reference Bengtson, Lipps and Waggoner2004; Peterson et al. Reference Peterson, McPeek and Evans2005; Seilacher et al. Reference Seilacher, Buatois and Mángano2005; Marshall, Reference Marshall2006). However, it is only in the last three decades that refinement of Ediacaran–Cambrian chronology has led to dramatic changes in our understanding of the rates of taxonomic succession in stratigraphic sections, ecological turnover, geochemical excursions and eustatic and epeirogenic changes through this key period in the co-evolution of Earth and life (Bowring et al. Reference Bowring, Grotzinger, Isachsen, Knoll, Pelechaty and Kolosov1993; Bowring & Schmitz, Reference Bowring and Schmitz2005; Erwin, Reference Erwin2006).
An accurate chronostratigraphy for the Cambrian Period has only gradually emerged since the mid-1990s, mainly through the application of U-Pb zircon geochronology of tuffs (Bowring et al. Reference Bowring, Grotzinger, Isachsen, Knoll, Pelechaty and Kolosov1993; Davidek et al. Reference Davidek, Landing, Bowring, Westrop, Rushton and Adrain1998; Landing et al. Reference Landing, Davidek, Westrop, Geyer and Heldmaier1998, 2000) coupled to biostratigraphic and carbon isotope chemostratigraphic correlation schemes (Maloof et al. Reference Maloof, Schrag, Crowley and Bowring2005, Reference Maloof, Porter, More, Dudás, Bowring, Higgins, Fike and Eddy2010). A progressive ‘younging’ of the period has taken place as first noted by Landing et al. (Reference Landing, Davidek, Westrop, Geyer and Heldmaier1998), with an early estimated base at c. 570 Ma and top at c. 510 Ma (Harland et al. Reference Harland, Armstrong, Cox, Craig, Smith and Smith1989), gradually refined through radioisotopic dating to c. 538 Ma (Bowring et al. Reference Bowring, Grotzinger, Isachsen, Knoll, Pelechaty and Kolosov1993; Grotzinger et al. Reference Grotzinger, Bowring, Saylor and Kaufman1995; Amthor et al. Reference Amthor, Grotzinger, Schröder, Bowring, Ramezani, Martin and Matter2003; Bowring et al. Reference Bowring, Grotzinger, Condon, Ramezani, Newall and Allen2007; Linnemann et al. Reference Linnemann, Ovtcharova, Schaltegger, Gärtner, Hautmann, Geyer, Vickers-Rich, Rich, Plessen, Hofmann, Ziegler, Krause, Kriesfeld and Smith2019; Landing et al. Reference Landing, Geyer, Schmitz, Wotte and Kouchinsky2021). Early Cambrian (Terreneuvian and Series 2) chronostratigraphy has been constrained through a radioisotopically calibrated global carbon isotope chemostratigraphic age model (Landing et al. Reference Landing, Davidek, Westrop, Geyer and Heldmaier1998, Reference Landing, Geyer, Schmitz, Wotte and Kouchinsky2021; Maloof et al. Reference Maloof, Schrag, Crowley and Bowring2005, Reference Maloof, Porter, More, Dudás, Bowring, Higgins, Fike and Eddy2010). However, there remains a paucity of radioisotopic anchors for the Middle (Miaolingian) and Late (Furongian) Cambrian epochs (Peng et al. Reference Peng, Babcock, Ahlberg, Gradstein, Ogg, Schmitz and Ogg2020). Note that in this report, Lower/Early, Middle/Middle, Upper/Late Cambrian are precisely defined subsystems/subperiods proposed to replace the undefined, subsystem-level terms ‘lower’/‘early,’ ‘middle’/‘middle,’ and ‘upper’/‘late’ of many reports (Landing et al. Reference Landing, Geyer, Schmitz, Wotte and Kouchinsky2021).
Recently, novel constraints on the timing of the base of the Miaolingian and Furongian epochs have come from detrital zircon studies in western Laurentia (Karlstrom et al. Reference Karlstrom, Mohr, Schmitz, Sundberg, Rowland, Blakey, Foster, Crossey, Dehler and Hagadorn2020; Cothren et al. Reference Cothren, Farrell, Sundberg, Dehler and Schmitz2022). In this area, precise and accurate chemical abrasion isotope dilution thermal ionization mass spectrometry (CA-IDTIMS) provides U-Pb zircon maximum depositional ages in mixed siliciclastic-carbonate strata of the Tonto Group (Grand Canyon) and Nounan and St. Charles formations (northern Utah) with a west Laurentian trilobite succession (Palmer, Reference Palmer1965; Sundberg et al. Reference Sundberg, Geyer, Kruse, McCollum, Pegel’, Żylińska and Zhuravlev2016; Lin et al. Reference Lin, Sundberg, Jiang, Montañez and Wotte2019). These studies suggest a shortening of the Miaolingian and Furongian Epochs by ∼3 million years, which is at odds with the only other constraint on the Early–Middle Cambrian boundary from British Avalonia (Harvey et al. Reference Harvey, Williams, Condon, Wilby, Siveter, Rushton, Leng and Gabbott2011).
This report follows from terminal Ediacaran–Ordovician litho- and biostratigraphic work on the Avalonia microcontinent beginning in the 1980s (by EL), with documentation and sampling of many tuffs (Supplementary Appendix 1). Biostratigraphic and systematic studies of the trilobite faunas comprise a long-term project (e.g. Westrop & Landing, Reference Westrop and Landing2011, Kim et al. Reference Kim, Westrop and Landing2002; Landing & Westrop, Reference Landing and Westrop1998; Landing et al. Reference Landing, Johnson and Geyer2008; Westrop et al. Reference Westrop, Landing and Dengler2018) with relevant new work in Supplementary Appendix 2. The results of field sampling and laboratory analyses of tuffs are reviewed for SE Newfoundland (Fig. 1). Five new U-Pb zircon depositional ages across the Lower–Middle Cambrian boundary interval are the focus of this contribution. They are significant for being consistent with the younger estimate for the base of the Miaolingian Epoch proposed by Karlstrom et al. (Reference Karlstrom, Mohr, Schmitz, Sundberg, Rowland, Blakey, Foster, Crossey, Dehler and Hagadorn2020) on the basis of detrital zircon CA-IDTIMS maximum depositional ages from SW Laurentia and for assessing the diachrony of local occurrences of diagnostic Miaolingian trilobites linked to onlaps of dysoxic (likely OMZ) water masses onto several Cambrian palaeocontinent shelves. The new dates also have implications for the biostratigraphic utility of some trilobite assemblages and constraining very rapid rates of epeirogenic uplift and subsidence driven by action along the Avalonian transform fault.

Figure 1. Lower–Middle Cambrian localities (in red) sampled in this project in SE Newfoundland for U-Pb zircon dating of tuffs. Small-scale map shows Ediacaran–Lower Ordovician cover sequence inliers (in black) unconformable on upper Neoproterozoic; inset map shows Avalonian Newfoundland. Horizons at SP, Re and RBr provided datable zircons (this report Figs. 3–5); remaining localities in Supplementary Appendix 1. Abbreviations: Bn, Branch; BS, Brigus Point South; CD, Cape Dog; DP, Duck Point; FP, Fosters Point; HC, Highland Cove; Ho, Hopeall Head; MR, Manuels River; RBr, Red Bridge Road; SP, Smith Point. In black, LM, Langlade, France; FD is global standard for the base of Cambrian at Fortune Head. Map drafted in Adobe Illustrator using a Google Maps base as a template and modified with permission from Landing (Reference Landing, Nance and Thompson1996, Fig. 1) based on subsequent field work.
2. Geological setting
2.a. Avalonia as terminal Ediacaran–Ordovician microcontinent and terrane
Avalonia is a major tectonostratigraphic unit (i.e. terrane) extending from Rhode Island through Maritime Canada and (fragmented by subsequent opening of the Atlantic Ocean) southern Britain through Belgium. It forms the core of the Appalachian–Caledonian orogen, where its tectonically isolated fragments form inliers with characteristic uppermost Ediacaran–Ordovician sedimentary rock-dominated successions that are unconformable on Neoproterozoic and older basement (Fig. 2). The Avalon zone is bounded by fault-juxtaposed, coeval marine successions to the SE (Meguma zone, now brought to Avalonia) and what is regarded as a problematical ‘Gander Zone’ to the NW that corresponds to the traditional Appalachian ‘central mobile belt’ (e.g. review and references in Landing et al. Reference Landing, Keppie, Keppie, Geyer and Westrop2022; Landing et al. Reference Landing, Webster, Bowser and Nance2023; Landing & Geyer, Reference Landing and Geyer2023; Landing et al. Reference Landing, Webster, Bowser and Nance2023). Two differing syntheses of early Avalonian evolution exist. The ‘Perigondwana paradigm’ emphasizes the Avalonian basement in the definition of Avalonia and regards Avalonia as a fragment detached from Gondwana possibly in the Early Ordovician (e.g. Murphy et al. Reference Murphy, Nance, Keppie, Dostal, Wilson, Houseman, McCaffrey, Doré and Buiter2018, and references therein). The ‘Peribaltic synthesis’ uses the terminal Ediacaran–Cambrian cover sequence that unconformably overlies a collage of Proterozoic blocks to define the Avalonia terrane and microcontinent (Landing et al. Reference Landing, Keppie, Keppie, Geyer and Westrop2022; Landing et al. Reference Landing, Webster, Bowser and Nance2023). The Avalonian basement may have formed close to Baltica by accumulation of crustal and oceanic fragments along the Avalonian transform fault (Atf). By this model, a modern analog of Avalonia is the North Scotia Ridge (Landing et al. Reference Landing, Keppie, Keppie, Geyer and Westrop2022).
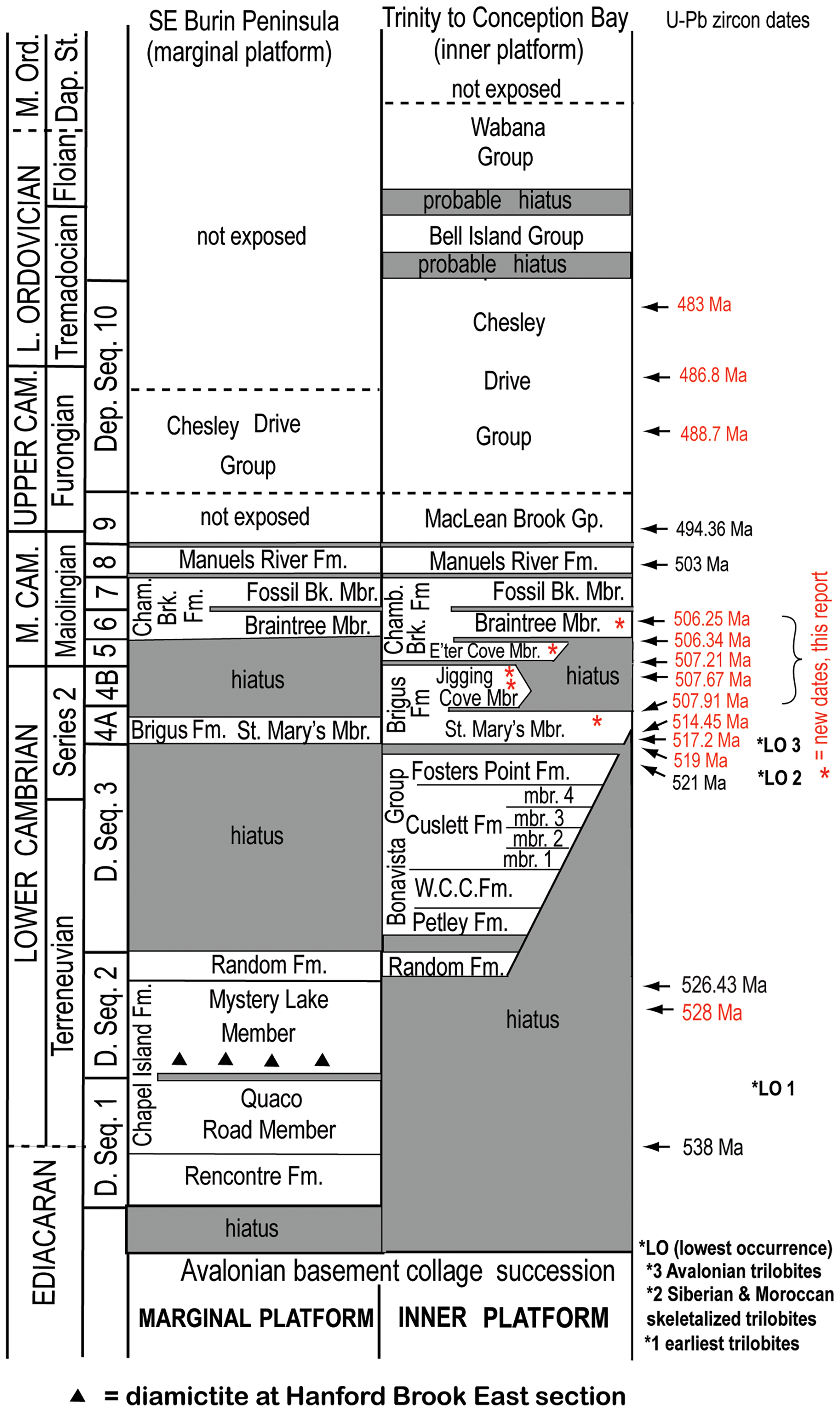
Figure 2. Geochronology and terminal Ediacaran–Lower Ordovician depositional sequence stratigraphy of Avalonian SE Newfoundland. Avalonian dates are in red. West–east cross-section of terminal Ediacaran–Lower Ordovician trans-Avalonian depositional sequences from Burin Peninsula (marginal platform) and east–west through Trinity–Conception bays (inner platform). Stratigraphy from Landing (Reference Landing, Nance and Thompson1996; also Landing et al. Reference Landing, Myrow, Narbonne, Geyer, Buatois, Mángano, Kaufman, Westrop, Kröger, Liang, Gougain and Landing2017, Reference Landing, Keppie, Keppie, Geyer and Westrop2022). Lowest occurrence (LO) of trilobites in Avalonia (Landing et al. Reference Landing, Geyer, Brasier and Bowring2013) and Siberia and Morocco (Landing et al. Reference Landing, Geyer, Schmitz, Wotte and Kouchinsky2021). Early phyletic origin of trilobites (Paterson et al. Reference Paterson, Edgecombe and Lee2019) preferred over c. 521 Ma date of Holmes and Budd (2022) who presumed heavy calcification in the definition of trilobites and left out the likelihood of weakly calcified Terreneuvian trilobites that may have produced Fortunian Cruziana and Rusophycus, as well as the collection artefact with the near absence of Terreneuvian soft-bodied fossil assemblages (Landing et al. Reference Landing, Geyer, Brasier and Bowring2013). U-Pb zircon dates: 483 Ma (Cape Breton Island, Landing et al. Reference Landing, Bowring, Fortey and Davidek1997); 486.8 Ma (North Wales, Landing et al. 2000); 488.7 Ma (North Wales, Davidek et al. Reference Davidek, Landing, Bowring, Westrop, Rushton and Adrain1998); 494.36 Ma (Arizona, U. S., Cothren et al. Reference Cothren, Farrell, Sundberg, Dehler and Schmitz2022, revises Peng et al. Reference Peng, Babcock, Cooper, Gradstein, Ogg, Schmitz and Ogg2012, of 497 Ma estimate); 503 (Germany, Landing et al. Reference Landing, Geyer, Buchwald and Bowring2014); 507.91, 507.67, 506.25 Ma (this report); 508.25 ± 2.75 Ma (New Brunswick, Landing et al. Reference Landing, Davidek, Westrop, Geyer and Heldmaier1998, recalculated by Schmitz, Reference Schmitz, Gradstein, Ogg, Schmitz and Ogg2020); 507.91, 514.45 Ma (England, Harvey et al. Reference Harvey, Williams, Condon, Wilby, Siveter, Rushton, Leng and Gabbott2011); 517.2 Ma (England, Williams et al. Reference Williams, Rushton, Cook, Zalasiewicz, Martin, Condon and Winrow2013); 526.43 (South China, Yang et al. In Press); 528 Ma (New Brunswick, Isachsen et al. Reference Isachsen, Bowring, Landing and Samson1994, recalculated by Schmitz, Reference Schmitz, Gradstein, Ogg, Schmitz and Ogg2020); 538 (Namibia, Linnemann et al. Reference Linnemann, Ovtcharova, Schaltegger, Gärtner, Hautmann, Geyer, Vickers-Rich, Rich, Plessen, Hofmann, Ziegler, Krause, Kriesfeld and Smith2019, reevaluated by Landing et al. Reference Landing, Geyer, Schmitz, Wotte and Kouchinsky2021). ‘Young’ Ediacaran–Cambrian boundary (c. 533 Ma; Nelson et al. Reference Nelson, Crowley, Smith, Schwartz, Hodgind and Schmitz2023) requires reevaluation (E. Landing & U. Linnemann, unpub. data). Abbreviations: Bk., Brook; Cham., Chamberlain’s; D., depositional; Dap. St., Dapingian Stage; Formation, Formation; Group, Group; *LO, lowest occurrences of trilobites; Mbr, Member; M., Middle; O., Ordovician; seq., sequence; W.C.C., West Centre Cove.
2.b. Avalonia of SE Newfoundland
SE Newfoundland is a standard of reference for Avalonian geological evolution, with ‘Avalonia’ named for the large Avalon Peninsula (Williams, Reference Williams1964, Reference Williams and Kay1969; Fig. 1). The cover sequence in SE Newfoundland and elsewhere in Avalonia is dominated by siliciclastic-dominated, limestone-poor, temperate latitude, shallow marine sedimentary rocks of the ‘Avalon Platform’ of Rast, O’Brien and Wardle (Reference Rast, O’Brien and Wardle1976; see Landing et al. Reference Landing, Keppie, Keppie, Geyer and Westrop2022; Landing et al. Reference Landing, Webster, Bowser and Nance2023). Cover sequence deposition was controlled by terminal Edicaran–Ordovician syndepositional faulting along the Avalon transform fault (Atf) (Landing, Reference Landing, Nance and Thompson1996; Landing et al. Reference Landing, Keppie, Keppie, Geyer and Westrop2022; Landing et al. Reference Landing, Webster, Bowser and Nance2023). Activity on the Atf produced NNE-striking faults (modern coordinates) and elongated NNE-striking basins and uplifts in a transtensional regime that was not a ‘rift environment’ (contra Álvaro, Reference Álvaro2021; Álvaro et al. Reference Álvaro, Johnson, Barr, Jensen, Palacios, van Rooyen and White2022). The SE migration of Avalonian depocenters through the Early Cambrian (Fig. 2) characterized this strike-slip regime (e.g. Noda, Reference Noda and Itoh2013). In addition, the production of coeval, minor, extensional and compressive igneous rocks including subaerial flows and tuffs in Avalonian sedimentary rocks (Figs. 3–6; Supplementary Appendix 1) has analogues in other transform fault settings as along the North Anatolian fault (Landing et al. Reference Landing, Keppie, Keppie, Geyer and Westrop2022, In press). Volcanogenic sandstones, tuffs, local volcanic edifices and intrusives common in the lower and upper parts of an Ads (Landing et al. Reference Landing, Keppie, Keppie, Geyer and Westrop2022). Landing et al. (Reference Landing, Keppie, Keppie, Geyer and Westrop2022, see section 16.5) discussed the seemingly peculiar association of coeval Na-alkalic tholeitic volcanics and intrusives with an extensional signature accompanying a silicic to mafic calc-alkaline suite with a collisional signature along the NW margin of Avalonia. This does not record different terranes (i.e. ‘Ganderia’ vs Avalonia) as concluded by some reports (e.g. Barr & White, Reference Barr, White, Nance and Thompson1996; Barr et al. Reference Barr, White and Miller2003; Barr et al. Reference Barr, White, Davis, McClelland and Van Staal2014a, Reference Barr, Bartsch, Miller and White2014b; vanh Rooyen et al. Reference van Rooyen, Barr, White and Hamilton2019). Rather, as noted above, it is interpreted to show coeval development of local collisional and extensional regimes along a transform fault.

Figure 3. Red Bridge Road section through quarries on south side of Red Bridge Road. Manuels River Formation and MacLean Brook Group truncated by Pleistocene ice-shove thrusts. Upper–Lower Cambrian Callavia broeggeri Zone and lower–Middle Cambrian Eccaparadoxides bennetti Zone ashes separated stratigraphically by 6.05 m and bracket Ads 4a–6 unconformity at 47° 28’ 58” N, 53° 00’ 34” W. Key: 1, cover; 2, siliceous mudstone, locally calcareous (with symbol); 3, quartz sand laminae; 4, interbedded mudstone and quartz sandstone; 5, quartz sandstone; 6, calcareous nodules; 7, methanogenic (sideritic) nodule; 8, bedded limestone; 9, manganese nodules (black), phosphatic clasts (red); 10, ooids and pisolites; 11, manganiferous (M), glauconite sand and clasts (G), pyritic (P); 12, lingulate brachiopods (left) and trilobites (centre and right); 13, burrows; 14, Coleoloides typicalis tubes, as bioclasts (left) and in situ and vertical (centre), hyoliths (right); 15, low SH-V stromatolites (right) and trans-Avalonian mud mound with in situ C. typicalis tubes is cap of Ads 3 (Landing et al. Reference Landing, Keppie, Keppie, Geyer and Westrop2022; Landing et al. Reference Landing, Keppie, Keppie, Geyer and Westrop2022); 16, unconformity; 17, tabular cross-sets; 18, hydrated Holyrood intrusive suite (Sparkes et al. Reference Sparkes, Beranek and Mills2021); 19, tuffs; 20, red to purplish red (r); 21, pink to brownish red (br); 22, green; 23; purple with/without greenish streaks. Abbreviations: Ads, Avalonian depositional sequence; B’tree, Braintree; C., Cavendish Formation (feather edge); Cham. Bk., Chamberlain’s Brook; E. e., Eccaparadoxides eteminicus; F., Fauna; F. Bk., Fossil Brook; Formation, Formation; Group, Group; M. hicksii, Mawddachites hicksii; Mbr, Member; M. C., Middle Cambrian; St., Stage; Subsys., Subsystem; Z., Zone. Laolingian Stage; Lenaldanian Series and Zhurinskyan Stage proposed by Landing et al. (Reference Landing, Geyer, Brasier and Bowring2013).

Figure 4. K-bentonite in upper St. Mary’s Member, Brigus Formation, east end of the second quarry on Red Bridge Road, 47° 28’ 58” N, 53° 00’ 34” W. Hammer for scale (30 cm). Picture courtesy of P. Parkhaev, Borissiak Paleontological Institute, Russian Academy of Sciences, Moscow.

Figure 5. Smith Point section: upper Lower–Middle Cambrian (upper Terreneuvian–lower Miaolingian) succession from Smith Point and west to cover; Lower–Middle Cambrian bracketed by dated ashes at 48° 11’ 59” N, 53° 52’ 15” W. Key and abbreviations in Fig. 3.
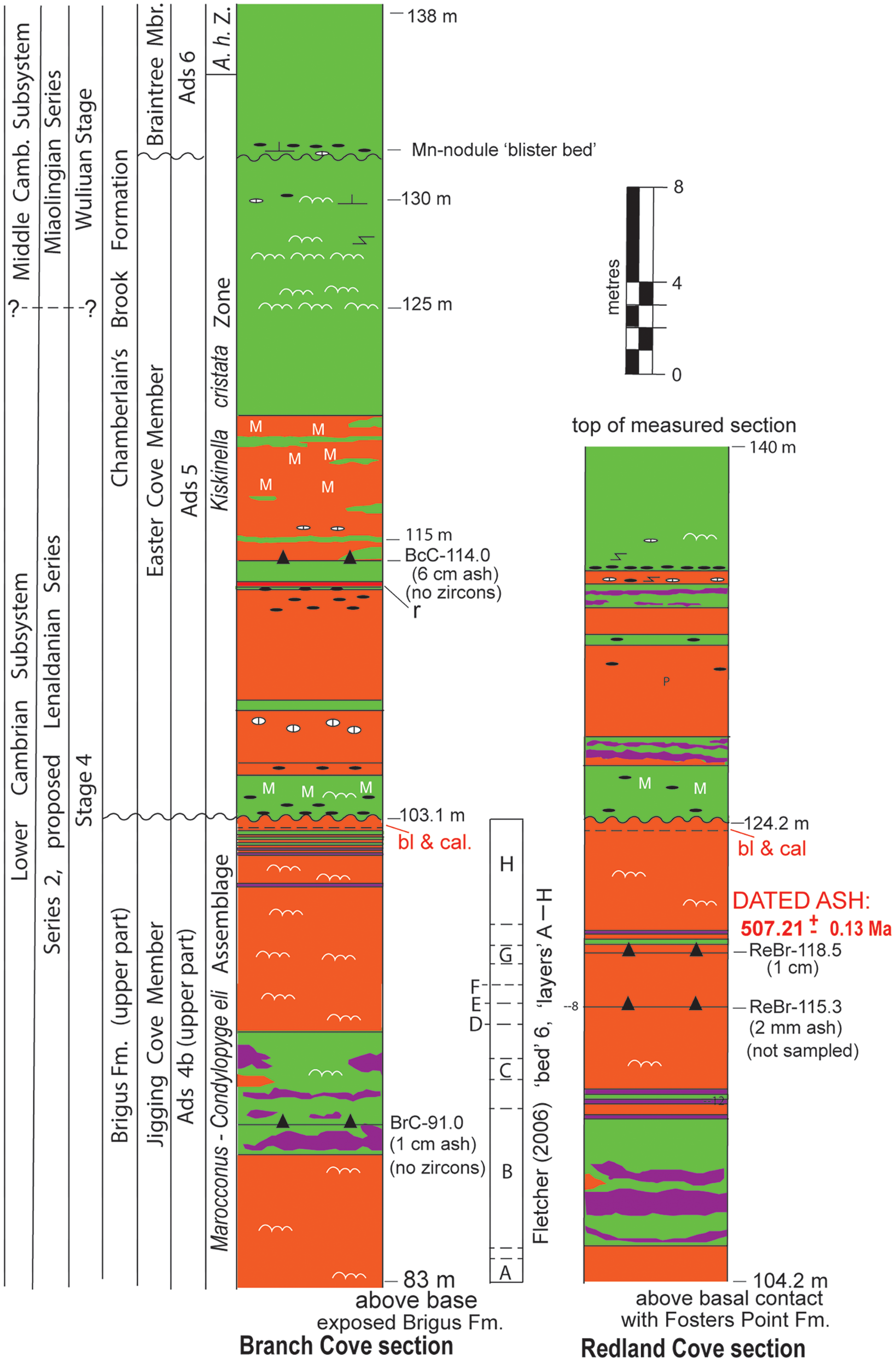
Figure 6. Lower–Middle Cambrian boundary interval at Branch and Redland coves, St. Mary’s Peninsula, SE Newfoundland (Fig. 1); dated uppermost Lower Cambrian ash at Redland Cove at 46° 48’ 45” N, 54° 07’ 23” W. No basis exists for precise correlation of Miaolingian Series’ base into Avalonia; the series’ base is questionably correlated into the Branch Cove section above Fletcher’s (2006) highest collected, ‘typically’ Lower Cambrian eodiscoid (Eoagnostus roddyi) in the Kiskinella cristata Zone (see Geyer, Reference Geyer2019). The K. cristata Zone is defined by the eponymous species’ range, which significantly overlaps the lower A. harlani range (Fletcher, Reference Fletcher2006). Fletcher (Reference Fletcher2006) referred the upper Jigging Cove Member of Landing and Westrop (Reference Landing and Westrop1998a, Reference Landing and Westrop1998b), without discussion, to a junior synonym he termed the ‘Branch Cove Member’. The uppermost Jigging Cove Member at Branch Cove was assigned to Fletcher’s (2006) ‘bed 6’ (i.e. an ‘interval 6’) with fossiliferous ‘layers’ (i.e. ‘units’) A–H. The report of Morocconus (i.e. Cephalopyge notabilis of Fletcher, Reference Fletcher2006) and Condylopyge eli from units B–H allows interval 6’s reference to a Morocconus-Condylopyge eli Assemblage (also Fig. 11). This assemblage name is applied as only one specimen of C. notabilis has been figured without discussion (Fletcher, Reference Fletcher2006, pl. 27, figs. 33, 34), and a cautious taxonomic approach is taken. Detailed correlation between Branch and Redland coves shows 5 m of differential erosion at the Ads 4b–5 (i.e. Brigus–Chamberlain’s Brook formation) contact. Thus, the dated tuff (ReBr-118.5) correlates with the top of Fletcher (Reference Fletcher2006) unit H. Subaerial exposure at the Ads 4a–5 contact is shown by bleaching (bl) and caliche (cal.) with a fine-grained crystic plasmic fabric (e.g. Allen, 1986) at the top of the Brigus. Symbols explained in Fig. 3 caption. Abbreviation: A. h. Z., Acadoparadoxides harlani Zone.
Eustatic changes accompanied development of terminal Ediacaran–Ordovician Ads alternations, but the dominant control was likely epeirogenic (e.g. Landing et al. Reference Landing, Keppie, Keppie, Geyer and Westrop2022, and references therein). This is seen by SE depocentre migration and narrow fault-defined NNE-trending depocentres, fracturing of the Avalonian basement with cover sequence onlap, and prominent volcanism at the top and base of some Ads alternations (i.e. base of Ads 8 and 9).
2.b. Ads succession and Lower–Middle Cambrian stratigraphy in SE Newfoundland
Transtensional faulting defined a marginal platform along NW Avalonia from Rhode Island to southern Britain, preserving the oldest (Ediacaran to early Terreneuvian/Lower Cambrian) sedimentary cover rocks (Fig. 2). By comparison, the Avalonian inner platform, the area that yielded the tuffs of this report, has a massive middle Terreneuvian white quartzite or younger units as the oldest Cambrian onlap deposit, with upper Lower Cambrian units such as the Brigus Formation locally overlying the Ediacaran basement (Landing, Reference Landing, Nance and Thompson1996; Landing et al. Reference Landing, Keppie, Keppie, Geyer and Westrop2022; Figs. 2, 3). Movement on the Atf led to coordinated faulting shown by regionally extensive Avalonian depositional sequences (Ads). Ten pre-Floian Ads alternations are recognized in North American (Landing, Reference Landing, Nance and Thompson1996; Landing et al. Reference Landing, Keppie, Keppie, Geyer and Westrop2022; Landing et al. Reference Landing, Webster, Bowser and Nance2023) and British Avalonia (Landing, Reference Landing, Nance and Thompson1996; Rees et al. Reference Rees, Thomas, Lewis, Hughes and Turner2014; Landing et al. Reference Landing, Keppie, Keppie, Geyer and Westrop2022; Landing et al. Reference Landing, Webster, Bowser and Nance2023).
Ads alternations are shoaling-up sequences (Fig. 2) that comprise member- or formation-level stratigraphic units. The base of an Ads is a diachronous unconformity that may show tens of metres or more of subaerial erosional cut-out and may be mantled by remané sediments that include fragments derived from any older cover sequence unit or the Proterozoic basement (Landing, Reference Landing, Nance and Thompson1996; Landing & Westrop, Reference Landing and Westrop1998a). Locally, the lowest part of an Ads unit is a carbonate clast conglomerate formed from thin-bedded nodular and bedded limestones cannibalized from initial deposits of the Ads itself. The base of the unconformably overlying Ads in SE Newfoundland is often a relatively thin onlap limestone, locally stromatolitic, that may overlie cyanobacterial build-ups of the underlying Ads as those of the Fosters Point Formation (Landing et al. Reference Landing, Keppie, Keppie, Geyer and Westrop2022; Landing et al. Reference Landing, Webster, Bowser and Nance2023; Fig. 2).
2.c. Lower–Middle Cambrian stratigraphy in SE Newfoundland
The trilobite-bearing, traditional Avalonian upper Lower–lower Middle Cambrian on the SE Newfoundland inner platform comprises the siliciclastic mudstone-dominated Brigus and Chamberlain’s Brook formations (Figs. 2, 3). The member-level subdivisions of these formations used herein (Landing, Reference Landing, Nance and Thompson1996; Landing & Westrop, Reference Landing and Westrop1998a) follow recommendations on nomenclatural in the international and North American stratigraphic codes (Salvador, Reference Salvador1994; North American Commission on Stratigraphic Nomenclature, 2005). The members are senior synonyms of those proposed by Fletcher (Reference Fletcher2006), who did not reference existing nomenclature and stratigraphic syntheses (e.g. Landing, Reference Landing, Nance and Thompson1996; Landing & Westrop, Reference Landing and Westrop1998a). Fletcher (Reference Fletcher2006) commonly relied on non-lithologic, supposed ‘separation planes’ to define the members (Westrop & Landing, Reference Westrop and Landing2011) and did not recognize trans-Avalonian depositional sequence unconformities that are geochronologically and epeirogenically significant (e.g. Landing, Reference Landing, Nance and Thompson1996; Rees et al. Reference Rees, Thomas, Lewis, Hughes and Turner2014).
2.c.1. Brigus Formation and St. Mary’s Member
The lower Brigus Formation is the St. Mary’s Member (Ads 4a), which has the lowest Avalonian trilobites. The trilobites commonly occur in a thin (up to 2 m) pinkish packstone (‘Clifton’ and later ‘Broad Cove’ members of Fletcher, Reference Fletcher2003, Reference Fletcher2006) that unconformably overlies the sub-trilobitic Foster’s Point Formation (e.g. Landing et al. Reference Landing, Keppie, Keppie, Geyer and Westrop2022; Figs. 2, 3). The St. Mary’s Member, with rare tuffs (Figs. 3, 4), corresponds to the Callavia Zone (e.g. Hutchinson, Reference Hutchinson1962) or C. broeggeri Zone (e.g. Landing & Westrop, Reference Landing and Westrop1998a; Fletcher, Reference Fletcher2006) and includes Fletcher’s (Reference Fletcher2006) ‘bed 1’ of his lower ‘Redland Cove Member’ (Figs. 3, 4). Callavia broeggeri is poorly known, not least because it is a large trilobite and, in our experience, specimens are often fragmentary and difficult to identify to the species level with confidence. Hutchinson (Reference Hutchinson1962) and Fletcher (Reference Fletcher2006) reported Callavia from the top of the traditional Smith Point Formation, which is now assigned to the base of the St. Mary’s Member (Landing & Benus, Reference Landing and Benus1988; Landing & Westrop, Reference Landing and Westrop1998b; Westrop & Landing, Reference Westrop and Landing2011), but no specimens were illustrated from this level. Only a few Callavia sclerites have been figured photographically to date from the Brigus Formation in SE Newfoundland (Hutchinson, Reference Hutchinson1962, pl. 24, Figs. 7–11, 14; Fletcher, Reference Fletcher2006, pl. 27, Fig. 3; Fletcher & Theokritoff, Reference Fletcher and Theokritoff2008, Fig. 5.3, 5.5, 5.6), and most of these are incompletely preserved. Revision of C. broeggeri will be presented elsewhere, but restudy of Hutchinson’s (Reference Hutchinson1962, pl. 24, Fig. 7–14) specimens and new material suggest there may be two species in the St. Mary’s Member (Westrop & Landing, unpublished data). The range of the genus in the St. Mary’s Member is reasonably well constrained, but species’ ranges should be used with caution. In this paper, the Callavia Zone refers to a unit essentially tied to the range of the genus, as in Avalonian Britain, whereas the C. broeggeri Zone is based on the first appearance of the eponymous species and may well represent only part of the Callavia Zone.

Figure 7. Strenuella strenua (Billings, Reference Billings1872), St. Mary’s Member, Brigus Formation, Brigus, Conception Bay, Newfoundland (Fig. 2, locality Br). Scale bars = 2 mm. (a–c) cranidium, GSC 269a (lectotype), dorsal, anterior, and lateral views, x7.5. (d–f) cranidium, GSC 269 (paralectotype), dorsal, lateral, and anterior views, x9. (g–i) cranidium, NBMG 23034, anterior, dorsal, and lateral views, collection BSBR 35.1, x9. (j) cranidium, NBMG 23035, dorsal view, collection BSBR-35.1, x12. (See Supplementary Appendix 2 for Systematic Palaeontology)

Figure 8. ‘Parasolenopleura’ cf. ‘P.’ ouangondiana (Hartt in Dawson, Reference Dawson1868), Braintree Member, Chamberlain’s Brook Formation, Red Bridge Road quarry, Kelligrews, Newfoundland, collection RBCB-38. Scale bars = 2 mm. (a–c) cranidium, NBMG 23036, dorsal, anterior and lateral views, x10. (d–f) cranidium, NBMG 23037, anterior, dorsal, and lateral views, x9.5. (See Supplementary Appendix 2 for Systematic Palaeontology)

Figure 9. Myopsostrenua cf. M. sabulosa Rushton (Reference Rushton1966), Jigging Cove Member, Brigus Formation, Smith Point, western Trinity Bay, Newfoundland, collection SPBr-24 (a, b) and St Mary’s Bay, Newfoundland, collection ReBr-61.7 (c–e). Scale bars = 2 mm. (a) cranidium, NBMG 23031, dorsal view, x3.75. (b) cranidium, NBMG 23032, dorsal view, x3.5. (c, d) cranidium, NBMG 23038, dorsal view, x6. (e) cranidium, NBMG 23039, dorsal view, x5. ‘Mallagnostus’ cf. ‘M.’ llarenai (Richter & Richter, Reference Richter and Richter1941) Jigging Cove Member, Brigus Formation, Smith Point, western Trinity Bay, Newfoundland, collection SPBr-40.95. (f) pygidium, NBMG 23033, dorsal view, x9. (See Supplementary Appendix 2 for Systematic Palaeontology)
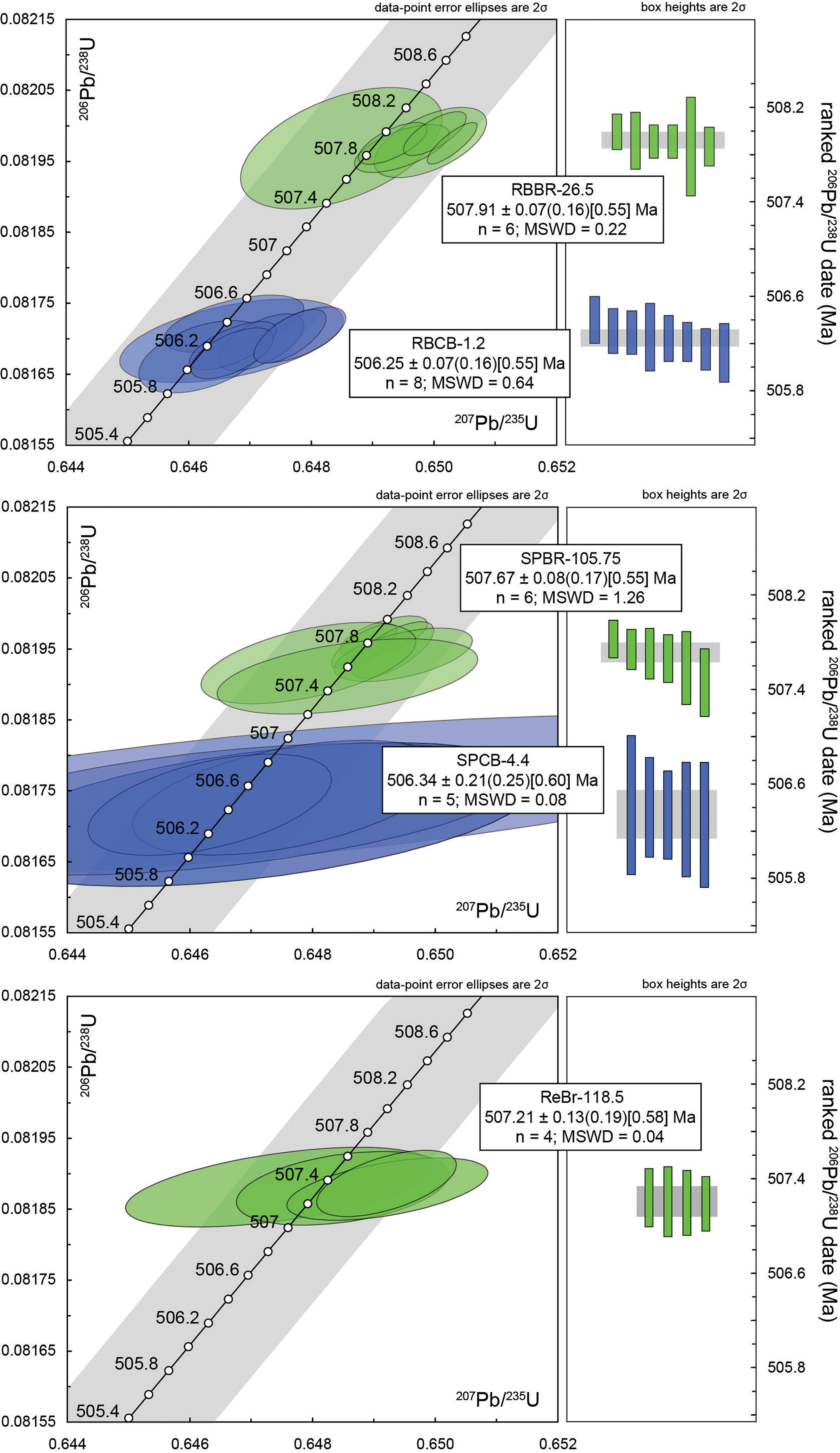
Figure 10. Concordia diagrams and ranked 206Pb–238U age plots for zircons from tuffs of the Brigus Formation and lower Chamberlain’s Brook Formation. Zircon ages were measured by CA-ID-TIMS.

Figure 11. Intercontinental correlation of Lower–Middle Cambrian boundary interval (e.g. Geyer, Reference Geyer2019) from Avalonian Newfoundland (e.g. Landing et al. Reference Landing, Keppie, Keppie, Geyer and Westrop2022; Landing et al. Reference Landing, Webster, Bowser and Nance2023; this report) to the Great Basin, SW Laurentia (e.g. Sundberg & McCollum, Reference Sundberg and McCollum2003; Webster, Reference Webster2011; Karlstrom et al. Reference Karlstrom, Hagadorn, Gehrel, Matthews, Schmitz, Madronich, Mulder, Pecha, Giesler and Crossey2018, Reference Karlstrom, Mohr, Schmitz, Sundberg, Rowland, Blakey, Foster, Crossey, Dehler and Hagadorn2020; Sundberg et al. Reference Sundberg, Karlstrom, Geyer, Foster, Hagadorn, Mohr, Schmitz, Dehler and Crossey2020); and North Greenland, NE Laurentia (e.g. Geyer & Peel, Reference Geyer and Peel2011).
Fletcher (Reference Fletcher2006) did not provide a complete taxonomic composition of his Brigus Formation trilobite zones. However, he equated the C. broeggeri Zone with what he called ‘Unit 1’ of his Redland Cove Member. Our field work (by EL) shows that the top of his Unit 1 in the type section of his Redland Cove Member (Fletcher, Reference Fletcher2006, Fig. 13) is a nodular limestone with a cap of calcareous sandstone. This is comparable to the cap of the C. broeggeri Zone in the Smith Point section and is thus interpreted to correlate with the basal Jigging Cove Member at the Smith Point section (Fig. 5, see section 4.2.3). In Fletcher’s (Reference Fletcher2006) sections, the base of the Jigging Cove Member (Ads 4b) corresponds to the first appearance of taxa of his ‘Strenuella’ sabulosa Zone (see Fig. 5; Myopsostrenua cf. M. sabulosa Fauna; Supplementary Appendix 2 has a brief discussion of the species).
2.c.2. Upper Brigus Formation and Jigging Cove Member
The Jigging Cove Member is equivalent to the ‘Jigging Cove’ and ‘Branch Cove’ members of Fletcher, Reference Fletcher2006; see Landing & Westrop, Reference Landing and Westrop1998b; Westrop & Landing, Reference Westrop and Landing2011, Fig. 2). This member is largely or completely absent in eastern Conception Bay (Fig. 1), unconformably overlies the St. Mary’s Member and comprises the upper Lower Cambrian of SE Newfoundland (Ads 4b). A distinctive conglomeratic limestone at the top of the St. Mary’s Member and ‘bed 1’ of Fletcher (Reference Fletcher2006) marks the appearance of the Myopsostrenua cf. M. sabulosa Assemblage at the unconformable base of the Jigging Cove Member (Ads 4b; Landing, Reference Landing, Nance and Thompson1996; Landing & Westrop, Reference Landing and Westrop1998b; Westrop & Landing, Reference Westrop and Landing2011, Figs. 2; 5; Supplementary Appendix 2). The upper bounding unconformity with the lower Chamberlain’s Brook Formation (Ads 5) shows subaerial exposure (i.e. bleaching and caliche) and erosional truncation of the Brigus Formation (Figs. 5, 6). With a Morocconus-Condylopyge eli Assemblage at its top (Fig. 6 and figure caption), the Jigging Cove has the highest known Avalonian Lower Cambrian faunas.
2.c.3. Chamberlain’s Brook Formation and Easter Cove Member
The lowest Chamberlain’s Brook Formation and base of the Avalonian Middle Cambrian is the Easter Cove Member (Fletcher, Reference Fletcher2006; i.e. ‘unnamed member’ of Landing, Reference Landing, Nance and Thompson1996, and Landing & Westrop, Reference Landing and Westrop1998a, Reference Landing and Westrop1998b). The Easter Cove Member comprises Ads 5 (Figs. 2, 5, 6) and is thickest on the St. Mary’s–west Trinity axis (Figs. 1, 5, 6). It lenses out and is locally absent in under a sequence boundary unconformity in eastern Conception Bay (Landing & Westrop, Reference Landing and Westrop1998a; Fig. 3). A manganese-nodule-rich bed unconformably overlying the Easter Cove Member is the base of the trans-North American Avalonian Braintree Member (Ads 6; Landing, Reference Landing, Nance and Thompson1996; Figs. 2, 5, 6). Kiskinella cristata Zone faunas with higher appearing specimens of Acadoparadoxides harlani persist through the Easter Cove into the lower Braintree Member, with the loss of K. cristata defining the base of the A. harlani Zone base (Fletcher, Reference Fletcher2006; Fig. 6, Supplementary Appendix 1).
2.c.3. Chamberlain’s Brook Formation – Braintree Member
The Braintree Member is an unconformity-bounded, siliciclastic mudstone-dominated interval (Ads 6) that comprises the majority of the Chamberlain’s Brook Formation (Figs. 2, 3). Whether in its type area in eastern Massachusetts (Geyer & Landing, Reference Geyer and Landing2001) or in SE Newfoundland, no local or regional basis exists for a consistent lithologic subdivision of the Braintree Member. Thus, the Braintree includes a succession of Fletcher’s (Reference Fletcher2006) members: ‘Wester Cove,’ ‘Waterfall Cove,’ ‘Big Gully,’ ‘Head Cove’ and most of the ‘Cape Shore’ (previously ‘Deep Cove’ Member of Fletcher, Reference Fletcher2003). This interval brackets the Acadoparadoxides harlani–Eccaparadoxides bennetti s.l. zones (Landing & Westrop, Reference Landing and Westrop1998a, Reference Landing and Westrop1998b; Landing et al. Reference Landing, Keppie, Keppie, Geyer and Westrop2022, Reference Landing, Webster, Bowser and Nance2023).
2.c.3. Chamberlain’s Brook Formation – Fossil Brook Member
Whether in SW New Brunswick; SE Newfoundland; or the Nuneaton area, England, the top of the Braintree Member and correlatives (i.e. upper Purley Shales) is capped by a thin (to 8 m) greenish mudstone-dominated interval (Ads 7) with basal trilobite packstone beds that unconformably overlie Ads 6. This is the top of the Chamberlains’ Brook Formation (Landing, Reference Landing, Nance and Thompson1996; Landing et al. Reference Landing, Webster, Bowser and Nance2023).
Ads 7 across North American Avalonia is the Fossil Brook Member with an Eccaparadoxides eteminicus Zone fauna (e.g. Kim et al. Reference Kim, Westrop and Landing2002). The basal limestone of Ads 7 was locally termed the ‘St. Mary’s Limestone’ within the ‘Deep Cove Member’ by Fletcher (Reference Fletcher2006). However, Fletcher’s (Reference Fletcher2006) ‘St. Mary’s (abandoned) is a junior homonym of the St. Mary’s Member of the Brigus Formation of Landing & Westrop, Reference Landing and Westrop1998a, Reference Landing and Westrop1998b). The greenish mudstones of the Fossil Brook Member are unconformably and diachronously overlain by the black Manuels River Formation (Ads 8; Landing, Reference Landing, Nance and Thompson1996; Landing et al. Reference Landing, Westrop and Geyer2023; Figs. 2, 3).
3. Lower–Middle Cambrian locales with zircon-bearing tuffs
3.a. Red Bridge Road
3.a.1. Location and stratigraphy
This unmetamorphosed, gently east-dipping Lower–Middle Cambrian succession is along the south side of Red Bridge Road (Fig. 1, loc. RBR) and south of Kelligrews village. It is exposed in a series of four small quarries used for road metal and fill (Landing & Benus, Reference Landing and Benus1988; Landing & Westrop, Reference Landing and Westrop1998a; Landing et al. Reference Landing, Myrow, Narbonne, Geyer, Buatois, Mángano, Kaufman, Westrop, Kröger, Liang, Gougain and Landing2017; Sparkes et al. Reference Sparkes, Beranek and Mills2021; Fig. 3). Red Bridge Road is a dirt track that intersects Rte. 60. The quarries are c. 7 km west of Rte. 60. The section extends for c. 300 m along Red Bridge Road. The Red Bridge Road succession is referable to the Avalonian inner platform because a relatively high Lower Cambrian unit, the Brigus Formation, nonconformably overlies Proterozoic basement (Figs. 2, 3, early Ediacaran Holyrood granite). The upper Red Bridge Road section includes the Middle Cambrian Chamberlain’s Brook Formation, Manuels River Formation and MacLean Brook Group.
3.a.2. Brigus Formation at Red Bridge Road
The lowest Brigus Formation at Red Bridge Road is 25 cm of neomorphic, pink lime mudstone with planar cyanobacterial mats, oncoids and wave-oriented Coleoloides typicalis tubes in the easternmost quarry. As elsewhere in Avalonia, such shallow-water facies commonly lack trilobites (e.g. Landing & Westrop, Reference Landing, Westrop, Lipps and Waggoner2004). The lower 10.75 m of the Brigus in the easternmost quarry consists dominantly of red brown with purple and green siliciclastic mudstone with trace fossils but no body fossils. The lack of trilobites in these lower mudstones may reflect continued shallow-water, oxic mudstone deposition known through the Avalonian terminal Ediacaran–Cambrian succession with low abundance of trilobites in the shallowest facies (e.g. Myrow & Landing, Reference Myrow and Landing1992; Landing & Westrop, Reference Landing, Westrop, Lipps and Waggoner2004) and with dissolution of trilobite sclerites in non-calcareous mud. Trilobite fragments appear at 10.75 m. The 14.7–21 m interval in the second quarry features Callavia broeggeri Zone assemblages with a 4 cm thick K-bentonite (RBBR-26.5) at 26.5 m (Fig. 4). These assemblages include the eponymous species, Serrodiscus ‘bellimarginatus’ (Shaler & Foerste), Strenuella strenua (Billings; see Fig. 7 and Supplementary Appendix 2), Triangulaspis vigilans (Matthew) and Hebediscus planus (Hutchinson). These taxa enter the succession with appearance of red shales with bedded limestones and calcareous nodules and may reflect a somewhat deeper mudstone facies and non-dissolution of trilobite (including eodiscoid) sclerites in more calcareous muds.
The Brigus Formation above a massive limestone (18.45–19.5 m, trilobite sample at 19.0 m) is dominated by sparsely fossiliferous, light green to olive siliciclastic mudstone. Trilobite-bearing calcareous nodules with C. broeggeri cranidia occur in the lower 2.5 m of these green mudstones, with structureless (burrow-churned) mudstones characterizing the upper Brigus Formation and ranging up to an unconformity at 31.35 m with the Chamberlain’s Brook Formation. These lithologies and presence of a Callavia broeggeri Zone assemblage indicate that the uppermost Brigus Formation at Red Bridge Road comprises only the St. Mary’s Member (Ads 4a) on the Avalonian inner platform (Fig. 2).
At the nearby section at the Manuels River bridge (Landing & Westrop, Reference Landing and Westrop1998a, stop 2; Fig. 2, loc. MR), uppermost Brigus Formation mudstones feature a massive, phosphatized, intraclast pebble wackestone (15.5–16.0 m) bed (Dale, Reference Dale1915, p. 380). This bed forms a pavement in the Manuels River at its first easterly bed. It is only 1.2 m below the unconformable base of the Chamberlain’s Brook Formation (Ads 6), which is a manganiferous, stromatolitic, dolomitic green siltstone (Howell, Reference Howell1925, bed 1). This conglomeratic bed is the base of an erosionally thinned Jigging Cove Member (Ads 4b) and marks the Ads 4a–4b sequence boundary (Landing & Westrop, Reference Landing and Westrop1998a, p. 24).
These observations and correlations are thus taken as evidence that the Brigus Formation is truncated at Red Bridge Road under the Chamberlain’s Brook Formation because the Jigging Cove Member (Ads 4b) is absent. As a result, the 4 cm tuff (sample RBBR-26.5; Fig. 4) intercalated 3 m above the highest Callavia. broeggeri Zone fossils is assigned tentatively to that zone due to the absence of the Jigging Cove Member, and as the St. Mary’s Member does not extend above the C. broeggeri Zone in SE Newfoundland (discussed below). The U-Pb depositional age for RBBR-26.5 is thus high in the upper Lower Cambrian (within Ads 4a), but is not uppermost Lower Cambrian (Fig. 2).
3.a.3. Chamberlain’s Brook Formation at Red Bridge Road
The base of the Chamberlain’s Brook Formation at 31.35 m in the Red Bridge Road section is at the east end of the second quarry and lies within green siliciclastic mudstone. An unconformable base of the Chamberlain’s Brook on the Brigus Formation is marked by the appearance of manganiferous carbonate nodules and manganese-impregnated LLH (laterally linked hemispheroid) mats 65 cm higher in the section. As noted by Howell (Reference Howell1925) at Manuels River, the lower Chamberlain’s Brook is poorly fossiliferous, and body fossils are limited to inarticulate brachiopods and paradoxidid fragments that he and Fletcher (Reference Fletcher2006, p. 74) brought to Eccaparadoxides bennetti. Diverse trilobites occur higher in the Fossil Brook Member (Ads 7) that forms the uppermost Chamberlain’s Brook at the eastern end of the third quarry and comprise the Eccaparadoxides eteminicus Zone assemblage (Landing & Westrop, Reference Landing and Westrop1998a, p. 20; Kim et al. Reference Kim, Westrop and Landing2002).
Two important beds occur in the burrow-homogenized, green siliciclastic mudstone with scattered manganiferous and calcareous nodules that forms most of the Chamberlain’s Brook Formation on Red Bridge Road. The first is a 5 cm thick tuff 1.2 m above the base of the formation (sample RBCB-1.2). The second is a 25 cm thick trilobite packstone bed 38 m above the base of the Chamberlain’s Brook. This packstone forms the base of the third quarry west of the Precambrian-Brigus contact and yielded a small trilobite assemblage with ‘Parasolenopleura’ cf. ‘P.’ ouangondiana (Hartt in Dawson; Fig. 8, Supplementary Appendix 2). The species supplies limited biostratigraphic control other than it resembles topotype P. ouangondiana from the younger Fossil Brook Member (Ads 7) in New Brunswick (e.g. Fletcher, Reference Fletcher2005) and is similar to ‘P.’ gregaria (Billings) in the Braintree Member (Ads 6). As noted above, this interval is a senior synonym of Fletcher’s (Reference Fletcher2006) ‘Big Gully Member’ of the Chamberlain’s Brook in SE Newfoundland (Landing & Westrop, Reference Landing and Westrop1998b). The lowest beds of the Chamberlain’s Brook at Red Bridge Road are therefore assigned to the Braintree Member (Ads 6) and probably lie well below the fossiliferous Fossil Brook Member (Ads 7) at the top of the Chamberlain’s Brook (Landing & Westrop, Reference Landing and Westrop1998a, p. 20; Fig. 2).
3.a.4. Sub-Chamberlain’s Brook unconformity at Red Bridge Road
Additional data refine the bio- and lithostratigraphic correlation of the K-bentonite in the lowest Chamberlain’s Brook Formation at Red Bridge Road, specifically the absence of highly manganiferous, purple to bright red and green striped mudstones of the lowest Middle Cambrian Easter Cove Member (Ads 5; Figs. 2, 5, 6), which occurs further west and SW along the Placentia–Bonavista and St. Mary’s–East Trinity axes (Landing, Reference Landing, Nance and Thompson1996; Fletcher, Reference Fletcher2006; Landing et al. Reference Landing, Keppie, Keppie, Geyer and Westrop2022; Landing et al. Reference Landing, Webster, Bowser and Nance2023). Furthermore, the oldest trilobites from the lowest Chamberlain’s Brook Formation at the nearby Manuels River section (Fig. 1, locality MB) were referred to the traditional Eccaparadoxides bennetti Zone by Howell (Reference Howell1925). This conclusion was reaffirmed by Fletcher (Reference Fletcher2006, p. 74) who reported E. bennetti and Agraulos affinis in the lowest Chamberlain’s Brook at Manuels River and in sections along southern Trinity and Conception bays (e.g. also Hutchinson, Reference Hutchinson1962, section 3, bed 39; section 10, bed 71). Fletcher (Reference Fletcher2006) followed Landing (Reference Landing, Nance and Thompson1996) and Landing and Westrop (Reference Landing and Westrop1998a, Reference Landing and Westrop1998b) in concluding that the lower Chamberlain’s Brook, or ‘unnamed member’ of Landing (Reference Landing, Nance and Thompson1996; Landing & Westrop, Reference Landing and Westrop1998a, Reference Landing and Westrop1998b), and Easter Cove Member of Fletcher (Reference Fletcher2006) are present in St. Mary’s and western Trinity bays but absent in Conception Bay.
The available data suggest that a U-Pb zircon date on the RBCB-1.2 tuff of the lowest Chamberlain’s Brook Formation at Red Bridge Road is not earliest Middle Cambrian. The age is an upper bracket on the composite Ads 4a–6 hiatus. The best interpretation of this age is based in part on absence of the lithologically distinctive Easter Cove Member that ranges into the Acadoparadoxides harlani Zone of the basal Chamberlain’s Brook (see Fletcher et al. Reference Fletcher, Theokritoff, Lord and Zeoli2005; Fletcher, Reference Fletcher2006; Figs. 2, 3, 6). In addition, the presence of an unillustrated lower Eccaparadoxides bennetti Zone assemblage in the lowest Chamberlain’s Brook at the nearby Manuels River section (Howell Reference Howell1925; Fletcher Reference Fletcher2006) suggests that the best correlation of the RBCB-1.2 tuff is into the E. bennetti Zone.
3.b. Branch and Redland coves
3.b.1 Location and stratigraphy
Branch and Redland coves lie on the west side of St. Mary’s Bay at the southern point of the SE Avalon Peninsula (Fig. 1, loc. Bn and Re). Landing and Westrop (Reference Landing and Westrop1998b) described and figured a section exposed in sea cliffs on the north side of Branch Cove, two kilometres east of Branch Village (Figs. 1, 6; Supplementary Appendix 1, loc. Bn) The section extends for about a kilometre along the coast and includes the upper Jigging Cove Member (top Ads 4b) of the Brigus Formation, unconformably overlain by the East Cove Member of the Chamberlain’s Brook Formation (base Ads 5).
The strata of the upper Jigging Cove Member are assigned to the uppermost Lower Cambrian upper Morocconus-Condylopyge eli Assemblage, following the report of Fletcher (Reference Fletcher2006) on his ‘bed’ 6 and our own collections in the same interval. Important for global correlation, Fletcher (Reference Fletcher2006) reports rare Oryctocara granulata specimens (two pygidia, one cranidium) in his ‘layer H’ of ‘bed 6’ within 1 m of the unconformity with the overlying lower Easter Cove Member (Ads 5) of the Chamberlain’s Brook Formation, with an Avalonian lowest Middle Cambrian Kiskinella cristata Zone fauna (Fig. 6). These litho- and biostratigraphic controls suggest that the hiatus between uppermost Lower Cambrian (Brigus Formation) and lowermost Middle Cambrian (Chamberlain’s Brook Formation) strata is at a minimum in this section.
Although zircons were not recovered from tuffs sampled at Branch Cove, these strata can be matched in detail with those of nearby Redland Cove (Fig. 6; see Westrop & Landing, Reference Westrop and Landing2011, Appendix 4 for a detailed log). The Redland Cove section lies on the east side of Redland Point, between Point Lance village and the ecological reserve at Cape St. Mary’s. The Brigus Formation is exposed from the tip of Redland Point to the shore, with manganese-nodule-bearing green mudstones of the lowermost Chamberlain’s Brook Formation exposed to the east at the end of the highest shore cliffs. At Redland Cove, a tuff (ReBr-118.5) occurs in the uppermost Jigging Cove Member, six metres below the unconformity (Fig. 6), at a horizon correlative to upper layer ‘H’ of Fletcher (Reference Fletcher2006) at Branch Cove. The depositional age of this tuff (c. 507.21 Ma) thus constrains the highest Lower Cambrian strata (i.e. Ads 4b) in SE Newfoundland.
3.c. Smith point
3.c.1. Location and stratigraphy
The Smith Point succession on the north shore of Smith Sound is one of the earliest documented and most famous Cambrian outcrops in SE Newfoundland (e.g. Matthew, Reference Matthew1899; Walcott, Reference Walcott1900; Fig. 1, loc. SP). This weakly metamorphosed inner platform sequence overlies Random Formation quartzites further east along Smith Sound at Clifton village (Fig. 2). The gently west-dipping section (Fig. 5) is measured from just east of the wharf at Smith Point hamlet (Landing & Benus, Reference Landing and Benus1988; Landing & Westrop, Reference Landing and Westrop1998a; Landing et al. Reference Landing, Myrow, Narbonne, Geyer, Buatois, Mángano, Kaufman, Westrop, Kröger, Liang, Gougain and Landing2017). The Ads 3–4a unconformity and base of the Brigus shows 15 m of relief on the top of the sub-trilobitic Bonavista Group (top of Fosters Point Formation). The lowest trilobite-bearing, 1.3 m thick limestone bed of the Brigus Formation crops out under the wharf (Fig. 5).
Poorly preserved trilobites (Callavia, Strenuella strenua; collection SPBr-0.6) at the base of the St. Mary’s Member of the Brigus Formation (i.e. Ads 4a) are assigned to the Callavia broeggeri Zone, but the rest of the member is barren. A 60 cm thick green, glauconitic calcareous sandstone with limestone and phosphatic pebbles is the base of the Jigging Cove Member of the Brigus Formation and forms the Ads 4a–4b boundary (Landing & Westrop, Reference Landing and Westrop1998a). Poorly preserved cranidia of Myopsostrenua cf. M. sabulosa (Rushton) appear immediately above the sandstone (Landing & Westrop, Reference Landing and Westrop1998a), and ‘Mallagnostus’ cf. ‘M.’ llarenai (Richter & Richter) occurs at 40.45 m (Figs. 5, 9; Supplementary Appendix 2). Related species occur in Fletcher’s (Reference Fletcher2006) Strenuella sabulosa Zone, which characterizes the base of the Jigging Cove Member in his southern Brigus sections (see section 3.1.3).
The Smith Point succession is comparable to the Branch Cove and Redland Cove sections (discussed below; Fig. 6) in recording the Brigus–Chamberlain’s Brook unconformity. A tuff almost at the top of the Jigging Cove Member of the upper Brigus Formation (SPBr-105.75; c. 507.67 Ma; Supplementary Appendix 1) and a tuff in the lower Easter Cove Member (SPCB-4.4, c. 506.34 Ma) provide precise U-Pb zircon dates. These dates are particularly significant as they bracket the traditional Avalonian Lower–Middle Cambrian boundary and the epeirogenic activity that led to definition of the Ads 4b–5 unconformity specifically in west Trinity Bay (see section 5.c.2).
The SPBr-105.75 tuff admittedly has limited biostratigraphic control but is regarded as upper Lower Cambrian. It occurs in unfossiliferous strata of the Jigging Cove Member well above the upper Lower Cambrian Myopsostrenua cf. M. sabulosa fauna at the base of the Jigging Cove Member in the Smith Point succession (Fig. 3). Its age may range as high as the Morocconus-Condylopyge eli Assemblage, which is possible as the Smith Point and Branch and Redland cove sections have a comparable epeirogenic and depositional history as they lie close to the axis of the St. Mary’s–west Trinity depocentre (Fig. 2).
The overlying manganiferous, purple and bright red mudstones at the top of the section unconformably overlying the Jigging Cove Member (Ads 4b) are assigned to the lowest Middle Cambrian Easter Cove Member of the Chamberlain’s Brook Formation (Ads 5, see section 4.a.3). Tuff sample SPCB-4.4 is only 4.4 m above the base of the unfossiliferous Easter Cove Member. Its location in the Easter Cove Member means the tuff and its depositional age can be assigned to the Kiskinella cristata Zone of the lowest Chamberlain’s Brook and Easter Cove Member as at Branch and Redland coves further south on the St. Mary’s–west Trinity depocentre (Figs. 1, 2, 6). This correlation is consistent with Fletcher’s (2006, p. 64) comment that the lowest Chamberlain’s Brook strata in western Trinity Bay (e.g. McCartney, Reference McCartney1967) have large specimens of Acadoparadoxides harlani, which appears within the K. cristata Zone. This depositional age provides a geochronological bracket on the lowest Chamberlain’s Brook at Smith Point and elsewhere where the Easter Cove Member and its correlatives occur from North American to British Avalonia (i.e. Ads 5, see Landing et al. Reference Landing, Webster, Bowser and Nance2023).
4. U-Pb zircon geochronology of SE Newfoundland tuffs
4.a. Analytical methods
Abundant populations of relatively small (c. 100–200 µm in long dimension), equant to elongate prismatic zircon crystals (Supplementary Fig. 1) were separated from bulk bentonite samples with an ultrasonic clay separator (Hoke et al. Reference Hoke, Schmitz and Bowring2014), followed by conventional density and magnetic methods. The entire zircon separate was placed in a muffle furnace at 900° C for 60 hours in quartz beakers to anneal minor radiation damage. Annealing enhances cathodoluminescence (CL) emission (Nasdala et al. Reference Nasdala, Lengauer, Hanchar, Kronz, Wirth, Blanc, Kennedy and Seydoux-Guillaume2002) and prepares the crystals for subsequent chemical abrasion (Mattinson, Reference Mattinson2005). Following annealing, individual sharply faceted, high aspect ratio grains with axial melt inclusions indicative of rapid subvolcanic growth were hand-picked for isotopic analysis.
U-Pb geochronology methods for chemical abrasion isotope dilution thermal ionization mass spectrometry (CA-IDTIMS) follow those published by Macdonald et al. (Reference MacDonald, Schmitz, Strauss, Halverson, Gibson, Eyster, Cox, Mamrol and Crowley2018). Zircon crystals are subjected to a modified version of the chemical abrasion method of Mattinson (Reference Mattinson2005), whereby single crystals are individually abraded in a single step with concentrated HF at 190° C for 12 hours. The remaining residual crystals were thoroughly rinsed before spiking with the ET2535 tracer, with complete dissolution at 220° C for 48 hours, followed by ion chromatographic purification of U and Pb and isotope ratio analysis by thermal ionization mass spectrometry. U-Pb dates and uncertainties for each analysis were calculated using the algorithms of Schmitz & Schoene (Reference Schmitz and Schoene2007) and the U decay constants of Jaffey et al. (Reference Jaffey, Flynn, Glendenin, Bentley and Essling1971; Supplementary Table 1). Uncertainties are based upon non-systematic analytical errors, including counting statistics, instrumental fractionation, tracer subtraction and blank subtraction. These error estimates should be considered when comparing our 206Pb/238U dates with those from other laboratories that used tracer solutions calibrated against the EARTHTIME gravimetric standards. When comparing our dates with those derived from other decay schemes (e.g. 40Ar/39Ar, 187Re-187Os), the uncertainties in tracer calibration (0.03%; Condon et al. Reference Condon, Schoene, McLean, Bowring and Parrish2015; McLean et al. Reference McLean, Condon, Schoene and Bowring2015) and U decay constants (0.108%; Jaffey et al. Reference Jaffey, Flynn, Glendenin, Bentley and Essling1971) should be added to the internal error in quadrature. Quoted errors for calculated weighted means are thus of the form ±X(Y)[Z], where X is solely analytical uncertainty; Y is the combined analytical and tracer uncertainty; and Z is the combined analytical, tracer and 238U decay constant uncertainty.
4.b. Results
4.b.1 sample RBBR-26.5
The heavy mineral separate for sample RBBR-26.5 from the St. Mary’s Member of the Brigus Formation at Red Bridge Road contains a dominant subpopulation of sharply facetted, equant to elongate prismatic crystals and subordinate subrounded crystals indicative of post-depositional reworking and holoclastic contamination (Supplementary Fig. 1). A total of eleven grains were selected for CA-IDTIMS analysis on the basis of sharply faceted morphology and high aspect. Five of these grains yielded a range in dates from 552 to 528 Ma and are interpreted as reworked detrital material. Six crystals that were distinguished by the presence of melt inclusions all produced concordant and equivalent isotope ratios, with a weighted mean 206Pb/238U date of 507.91 ± 0.07 (0.16) [0.55] Ma (2s; MSWD = 0.22). Given the reproducibility of these zircon crystals with morphologies and melt inclusions typical of rapid growth in the subvolcanic magma system, this date may be interpreted as closely approximating the volcanic eruption and depositional age in the late Early Cambrian (Fig. 10; Supplementary Table 1).
4.b.2. Sample RBCB-1.2
Sample RBCB-1.2 from the Chamberlain’s Brook Formation at Red Bridge Road produced a mineral separate dominated by sharply faceted morphologies, high aspect and the presence of melt inclusions (Supplementary Fig. 1). Eight single grains analysed by CA-IDTIMS produced concordant and equivalent isotope ratios, with a weighted mean 206Pb/238U date of 506.25 ± 0.07 (0.16) [0.55] Ma (2s; MSWD = 0.64). Given the reproducibility of these zircon crystals with morphologies typical of rapid growth in the subvolcanic magma system, this date (Fig. 10; Supplementary Table 1) may also be interpreted as closely approximating the volcanic eruption and depositional age in the early Middle Cambrian.
4.b.3. Sample SPBr-105.75
A total of nine zircon grains from sample SPBr-105.75 from the Jigging Cove Member of the Brigus Formation in the Smith Point succession were selected for CA-IDTIMS analysis from a mineral separate dominated by prismatic apatite with subordinate sharply faceted zircon crystals (Supplementary Fig. 1). Three of these grains yielded a range in dates from 530.3 to 528.9 Ma and are interpreted as reworked detrital material. Six crystals that were distinguished by a more equant habit and the presence of abundant melt and mineral inclusions all produced concordant and equivalent isotope ratios, with a weighted mean 206Pb/238U date of 507.67 ± 0.08 (0.17) [0.55] Ma (2s; MSWD = 1.26) (Supplementary Table 1). Given the reproducibility of these zircon crystals with morphologies and melt inclusions typical of rapid growth in the subvolcanic magma system, this date may be interpreted as closely approximating the volcanic eruption and depositional age in the latest Early Cambrian (Figs. 5, 10).
4.b.4. Sample SPCB-4.4
The mineral separate from sample SPCB-4.4 from the Easter Cove Member of the lowermost Chamberlain’s Brook Formation in the Smith Point succession (Fig. 5) yielded a minor amount of small, colourless, prismatic, equant zircon crystals. Of eight crystals prepared for chemical abrasion, two did not yield enough radiogenic Pb and U to produce reliable results, and a third grain yielded a Terreneuvian date attributed to inheritance. The remaining five crystals produced concordant and equivalent isotope ratios, with a weighted mean 206Pb/238U date of 506.34 ± 0.21 (0.25) [0.60] Ma (2s; MSWD = 0.08). Although the small size and low U content of these zircons produced less precise analyses (Supplementary Fig. 1; Supplementary Table 1), the resulting interpreted eruption and depositional age is slightly older than that of sample RBCB-1.2, consistent with its lower stratigraphic position within the lowest Easter Cove Member of the Chamberlain’s Brook Formation (Figs. 5, 10).
4.b.5. Sample ReBr-118.5
Only a few dozen small, prismatic, slightly elongate zircon grains were obtained from a very barite-rich mineral separate from sample ReBr-118.5 of the uppermost Jigging Cove Member of the upper Brigus Formation in Redland Cove (Fig. 6, Supplementary Fig. 1). Of seven crystals selected for chemical abrasion, one was completely dissolved and two other grains yielded latest Ediacaran or Terreneuvian dates. The remaining four crystals produced concordant and equivalent isotope ratios, with a weighted mean 206Pb/238U date of 507.21 ± 0.13 (0.19) [0.58] Ma (2s; MSWD = 0.04). This is the litho- and biostratigraphically highest dated horizon of the Brigus Formation and produced the youngest interpreted Early Cambrian eruption and depositional age (Figs. 6, 10; Supplementary Table 1).
5. Discussion
5.a. Radioisotopic insights into Avalonian Lower Cambrian trilobite zonal durations
The oldest identifiable British Avalonian trilobites are known in the Comley area in the English Midlands (see review in Rushton, Reference Rushton and Holland1974). Here, the Green Callavia Sandstone (traditionally termed Ac1; e.g. Raw, Reference Raw1936, p. 238) is the top of the Lower Comley Sandstones. This very thin sandstone has Callavia callavei (Lapworth) and Hebediscus ‘attleborensis’ (Shaler & Foerste) (which was restricted to the types from Massachusetts by Westrop & Landing, Reference Westrop and Landing2011). A tuff in the upper Green Callavia Sandstone is dated at 514.45 ± 0.36 Ma (Williams et al. Reference Williams, Rushton, Cook, Zalasiewicz, Martin, Condon and Winrow2013). Detailed correlation with SE Newfoundland is hindered because C. callavei is even more poorly known than C. broeggeri. More recent studies have referred the former to a different genus, Callavonia Lieberman (Lieberman, Reference Lieberman2001) or have treated the latter as the senior synonym of C. callavei (Fletcher & Theokritoff, Reference Fletcher and Theokritoff2008). The most comprehensive treatment of C. callavei is Raw’s (1936), but until his specimens are restudied, synonymy with C. broeggeri is, in our view, premature. As the Green Callavia Sandstone and St. Mary’s Member have the oldest unequivocal records of Callavia sensu lato (see Lieberman, Reference Lieberman2001, for an alternative classification), it is likely that these units are at least partly correlative.
Overlying strata at Comley are the Lower Comley Limestones which include a thin ‘Red Callavia Sandstone’ (0.75 m, Ac2) overlain by a metre of limestone (c. 1.0 m). The latter interval includes four beds (Ac3-5 and Ad) with distinctive fossil assemblages that form an exceptionally condensed interval with unconformities between each bed (Landing, Reference Landing, Nance and Thompson1996). The Red Callavia Sandstone with C. callavei (e.g. Raw, Reference Raw1936) may also be partly correlative with the St. Mary’s Member in SE Newfoundland. Rushton (Reference Rushton2011, Fig. 12; Harvey et al. Reference Harvey, Williams, Condon, Wilby, Siveter, Rushton, Leng and Gabbott2011) assigned the overlying limestone units (Ac3 and Ac4) to Fletcher’s (2006) Strenuella sabulosa Zone (see Myopsostrenua cf. sabulosa Zone, Fig. 5 and Supplementary Appendix 2 for a review of Myopsostrenua) and Geyerorodes (formerly Orodes) Zone, presumably because they lie above the Callavia-bearing interval. Bed Ac5, the Lapworthella Limestone at the top of the Comley Limestones, lacks biostratigraphically diagnostic fossils. Ac5 may be uppermost Lower Cambrian or lower Middle Cambrian (Rushton, Reference Rushton and Holland1974), with Harvey et al. (Reference Harvey, Williams, Condon, Wilby, Siveter, Rushton, Leng and Gabbott2011, Fig. 2) arbitrarily showing it straddling the Lower–Middle Cambrian boundary.
Although Callavia has been reported from Ac3 as C. (Cobboldus) cobboldi Raw, the holotype (Raw, Reference Raw1936, pl. 20, Fig. 1a–c) is so poorly preserved that Raw (Reference Raw1936, p. 264) noted that it is ‘too imperfect for satisfactory comparisons with other forms’. It does not offer a basis for biostratigraphic correlation, with the species treated as a synonym of Nevadella cartlandi Raw (in Walcott, Reference Walcott1910) by Lieberman (Reference Lieberman2001). Fletcher and Theokritoff (Reference Fletcher and Theokritoff2008) referred some specimens from the Strenuella Limestone (Ac4) to Strenuella strenua (Billings), but this species can be identified with confidence only in the Brigus Formation of SE Newfoundland (see Supplementary Appendix 2). Serrodiscus bellimarginatus (Shaler & Foerste) is reported from Ac3 but, as revised by Westrop and Landing (Reference Westrop and Landing2011), this species is also problematic, with variation in spinose axial nodes on the pygidium that might be intra-specific but could record a plexus of pseudocryptic species similar to Eodiscus (see Westrop et al. Reference Westrop, Landing and Dengler2018). In short, unresolved taxonomic issues limit the accuracy of correlation. If successions with unequivocal Callavia species are equated, then the St. Mary’s Member is broadly correlative with the Green and Red Callavia sandstones, with much of the Comley Limestone recording a post-Callavia interval. The correlation of Ac3 is equivocal and depends on the interpretation of S. ‘bellimarginatus’. It could correlate with the C. broeggeri Zone, but it could be younger because the species occurs with Myopsostrenua sabulosa in the Purley Shale in the Nuneaton district (Rushton, Reference Rushton1966, p. 5).
Trilobite-based correlations and the new U-Pb dates mean the c. 507.9 Ma age on what is probably the upper Callavia broeggeri Zone at Red Bridge Road is likely no younger than the Comley Ac2 limestone. In addition, the c. 507.7 Ma age on the Jigging Cove would be younger than the Myopsostrena sabulosa Zone. The c. 514.5 Ma date on the Green Callavia Sandstone means the traditional Callavia Zone has an extraordinary duration of at least c. 6.78 Ma as the Red Bridge Road tuff is from the top of this interval. However, the potential range of Callavia in Avalonian Britain could extend below the Green Callavia Sandstone. Further east in the English Midlands at Nuneaton, Williams et al. (Reference Williams, Rushton, Cook, Zalasiewicz, Martin, Condon and Winrow2013) determined a 517.2 ± 0.2 Ma zircon age on the lower Purley Shales Formation in association with Callavia sp. fragments. The sequence stratigraphy of the Nuneaton succession provides a better basis for correlation than trilobite fragments (Williams et al. Reference Williams, Rushton, Cook, Zalasiewicz, Martin, Condon and Winrow2013, Fig. 4) that cannot be identified with confidence. A sequence stratigraphic analysis shows the underlying sub-trilobitic Home Farm Limestone with vertically arranged, in situ Coleoloides is not only correlative biostratigraphically and by carbon isotope stratigraphy but is even lithologically comparable with the Fosters Point Formation (Ads 3) in SE Newfoundland (e.g. Landing et al. Reference Landing, Keppie, Keppie, Geyer and Westrop2022; Figs. 2, 5). Both the Home Farm and Fosters Point are correlative with the ca. 519–520 Ma interval in Siberia with the onset of the IV 13C excursion and within the range of the oldest Siberian trilobites (Brasier et al. Reference Brasier, Anderson and Corfield1992; Landing, Reference Landing, Nance and Thompson1996; Landing & Kouchinsky, Reference Landing and Kouchinsky2016; Landing et al. Reference Landing, Schmitz, Geyer, Trayler and Bowring2020, Reference Landing, Keppie, Keppie, Geyer and Westrop2022). This means the non-sequence with the Home Farm Member and overlying thin Woodlands Member sandstones (e.g. Williams et al. Reference Williams, Rushton, Cook, Zalasiewicz, Martin, Condon and Winrow2013, Fig. 1) is the Ads 3–4a boundary and that the lower Purley is correlative with the lower Brigus Formation.
With the c. 507.9 Ma date on Red Bridge Road sample RBBR-26.5 and the c. 517.2 Ma date at Nuneaton, the Callavia/Callavia broeggeri Zone is thus extraordinarily long by comparison with other metazoan-based biozones. It has a longer duration than even the entire Upper Cambrian (i.e. Cothren et al. Reference Cothren, Farrell, Sundberg, Dehler and Schmitz2022). Alternatively, this long duration could hint at potential problems in the correlation and dating of the base of the zone in Avalonian Britain. In either case, further study and differentiation of this late Series 2 chronostratigraphy is warranted.
5.b. Lower–Middle Cambrian boundary interval and evaluation of Miaolingian series
5.b.1. Radioisotopic calibration of Lower–Middle Cambrian boundary interval
A precise and correctly evaluated geochronology across the Lower–Middle Cambrian boundary interval brackets significant biotic and geological events. This interval is the twilight of the Cambrian Evolutionary Radiation as it includes the origination and diversification of the last high level and ecologically important metazoan groups – i.e. echinoderms and appearance of variety of colonial clades (e.g. Sprinkle, Reference Sprinkle, Lipps and Signor1992; Landing et al. Reference Landing, Antcliffe, Geyer, Kouchinsky, Andreas and Bowser2018). Profound changes in palaeoceanographic circulation and sea level are commonly said to be associated with the boundary interval (e.g. Nielsen & Schovsbo, Reference Nielsen and Schovsbo2015), although reinterpretation of Early–Middle Cambrian boundary sea-level changes does not indicate a eustatic regression (i.e. Hawke Bay event) as noted in many reports (Landing et al. Reference Landing, Webster, Bowser and Nance2023).
The International Commission on Stratigraphy (ICS) and International Union of Geological Scientists (IUGS) agreed to define the coterminous bases of the Miaolingian Series and Wuliuan Stage at the FAD (first appearance datum) of the trilobite Oryctocephalus indicus (Reed) in the Wuliu-Zengjiayan (W-Z) quarry in South China (Zhao et al. Reference Zhao, Jinliang, Babcock, Guo, Peng, Yin, Yang, Peng, Wang, Gaines, Esteve, Tai, Yang, Wang, Sun and Yang2019). The global Miaolingian was a way to resolve the problems of locally defined and temporally distinct Lower–Middle Cambrian series boundaries defined in highly provincial faunal successions on each Cambrian palaeocontinent. The Miaolingian base has also been used as the base of a proposed Middle Cambrian subsystem (Landing et al. Reference Landing, Geyer, Schmitz, Wotte and Kouchinsky2021; this report). The caveats to this ICS-accepted definition are that the series is defined at the FAD of O. Indicus, and secondly, this FAD is associated with, and possibly driven by, strong environmental change (section 5.b.4). This basal horizon is better designated as the ‘lowest occurrence’ (LO). A FAD is a phyletic origination event unlikely to be encountered in field work. In addition, reliance on the LO of a taxon for global correlation, as that of O. indicus, is compounded by collecting and taphonomic biases and local environmental features (as dysoxia) that preclude finding the earliest representatives of a taxon in any succession or using the LO for reliable chronostratigraphic correlations and definitions (e.g. Landing et al. Reference Landing, Geyer, Brasier and Bowring2013; Aubrey, Reference Aubrey, Rink, Thompson, Jull, Paces and Heaman2015). The strong environmental changes associated with the LO of O. indicus should have prohibited its use as a global standard (see Cowie et al. Reference Cowie, Ziegler, Boucot, Bassett and Remane1986, for recommended procedures for ICS and IUGS in establishing chronostratigraphic standards).
By one interpretation, this boundary is a dramatic interval in trilobite successions with major changes synchronous between faunal provinces/realms, which would allow for easy correlation through the boundary interval (e.g. Babcock et al. Reference Babcock, Peng and Ahlberg2017; Zhao et al. Reference Zhao, Jinliang, Babcock, Guo, Peng, Yin, Yang, Peng, Wang, Gaines, Esteve, Tai, Yang, Wang, Sun and Yang2019). However, these syntheses have been challenged by studies that conclude the last occurrences of the redlichioid and olenelloid trilobites, and the earliest occurrences of paradoxidids are diachronous and overlap between several palaeocontinents in the Lower–Middle Cambrian boundary interval, which confounds interregional correlation (e.g. Geyer, Reference Geyer2019; Fletcher, Reference Fletcher2003; Sundberg & McCollum, Reference Sundberg and McCollum2003; 2005; Sundberg et al. Reference Sundberg, Geyer, Kruse, McCollum, Pegel’, Żylińska and Zhuravlev2016, Reference Sundberg, Karlstrom, Geyer, Foster, Hagadorn, Mohr, Schmitz, Dehler and Crossey2020).
Specifically, Sundberg et al. (Reference Sundberg, Karlstrom, Geyer, Foster, Hagadorn, Mohr, Schmitz, Dehler and Crossey2020) summarize work that shows the LO of Oryctocephalus indicus and base of their O. indicus Zone and the Miaolingian Series is two Laurentian trilobite zones above the highest olenellids in SW Laurentia and combined this biostratigraphy with a zircon maximum depositional age (MDA) of ≤ 506.6 ± 0.3 Ma on the highest olenellids in the Grand Canyon region (Karlstrom et al. Reference Karlstrom, Mohr, Schmitz, Sundberg, Rowland, Blakey, Foster, Crossey, Dehler and Hagadorn2020). Sundberg et al. (Reference Sundberg, Karlstrom, Geyer, Foster, Hagadorn, Mohr, Schmitz, Dehler and Crossey2020) thus conclude that the Miaolingian Series base is ≤ 506.6 ± 0.3 Ma and that the highest occurrence of East Gondwanan (i.e. South China) redlichioids is close to but higher than that of the highest Laurentian olenellids and assign a c. 506 Ma age to the highest redlichioids. These temporal markers are significantly younger than the aforementioned tuff date of 509.1 ± 0.2 Ma just below the earliest local occurrences of paradoxidids and the interpreted Lower–Middle Cambrian boundary in British Avalonia (Harvey et al. Reference Harvey, Williams, Condon, Wilby, Siveter, Rushton, Leng and Gabbott2011), although paradoxidids actually occur lower in the upper Lower Cambrian Marocconus-Cephalopyge eli Assemblage at Branch Cove, SE Newfoundland (Fletcher, Reference Fletcher2006). The presumption in this analysis (Sundberg & McCollum, Reference Sundberg and McCollum2003) is that the LO of O. indicus is a precise, synchronous geochronologic marker between the SW US and South China.
5.b.2. Biostratigraphic correlations through Avalonian Lower–Middle Cambrian boundary interval
That the Oryctocephalus indicus LO is a precise marker for global correlation is testable by a presumed correlation of the Miaolongian Series’ base into the Avalonian lower Acadoparadoxides harlani Zone (Geyer, Reference Geyer2005, Reference Geyer2019; Sundberg et al. Reference Sundberg, Geyer, Kruse, McCollum, Pegel’, Żylińska and Zhuravlev2016, Reference Sundberg, Karlstrom, Geyer, Foster, Hagadorn, Mohr, Schmitz, Dehler and Crossey2020; Zhao et al. Reference Zhao, Jinliang, Babcock, Guo, Peng, Yin, Yang, Peng, Wang, Gaines, Esteve, Tai, Yang, Wang, Sun and Yang2019). This intercontinental correlation, which is modified below, was based upon correlating the lowest Miaolingian into an interval above three key taxa known in SE Newfoundland. At Branch Cove, Fletcher (Reference Fletcher2006) reported Ovatoryctocara granulata, which appears below Oryctocephalus indicus at the GSSP, at the top of the Morocconus-Condylopyge eli Assemblage in the upper Jigging Cove Member (the senior synonym of the ‘Branch Cove Member’ of Fletcher, Reference Fletcher2006; Figs. 6, 11). The unconformably overlying Kiskinella cristata Zone of the Easter Cove Member (lowest Chamberlain’s Brook Formation, Ads 5; Figs. 6, 11) has Eoagnostus roddyi and Condylopyge eli, known from the traditional uppermost Lower Cambrian in Laurentia and Siberia, respectively. The K. cristata Zone corresponds to the range of K. cristata, with A. harlani appearing in the middle of the zone and overlapping the upper ranges of E. roddyi and C. eli in the upper Easter Cove Member (Fletcher, Reference Fletcher2006) and persisting through the supra-Easter Cove unconformity into the Braintree Member. Consequently, a proposed correlation of the lower Miaolingian should include the A. harlani and upper K. cristata zones.
These correlations must be qualified. They are based on a simplistic presence-absence approach to biostratigraphy. Thus, Ovatoryctocara granulata at Branch Cove has limited significance for interregional correlation. Its three known sclerites (Fletcher, Reference Fletcher2003) presumably came from a calcareous nodule horizon at the top of the Jigging Cove Member (Fletcher, Reference Fletcher2006, Fig. 15). Thus, its reported ‘range’ (one horizon) is a taphonomic artefact with subsequent work not recording the nodules or additional O. granulata specimens over three field seasons (by EL, EL with GG, El with SRW) and during a 1997 visit by the Cambrian Subcommission (Landing & Westrop, Reference Landing and Westrop1998b, pp. 42–44).
The known range of fossil taxa underestimates their true stratigraphic longevities (e.g. Marshall, Reference Marshall2006). For purposes of discussion, the ‘typical’ upper Lower Cambrian Eoagnostus roddyi has a 22 m range (including its appearance below the Jigging Cove–Easter Cove member unconformity) at Branch Cove where it has three reported fossil horizons. (Fletcher, Reference Fletcher2006, pp. 57, 65). At 50% and 95% levels of confidence (e.g. Marshall, Reference Marshall1990), respectively, the confidence interval (CI) of its upper stratigraphic range is 9 m higher in the upper Kiskinella cristata Zone of the lower Braintree Member and 74 m higher in the lower Eccaparadoxides bennetti Zone. The 74 m upper CI brings the traditional Avalonian Middle Cambrian E. bennetti Zone, surprisingly, into correlation with the upper Lower Cambrian of Laurentia and Siberia and below the Miaolingian following Sundberg et al.’s (Reference Sundberg, Geyer, Kruse, McCollum, Pegel’, Żylińska and Zhuravlev2016, Reference Sundberg, Karlstrom, Geyer, Foster, Hagadorn, Mohr, Schmitz, Dehler and Crossey2020) correlation procedures. A modest upper CI for Condylopyge eli (eight horizons, 49 m known range; Fletcher, Reference Fletcher2006) at a 95% level of confidence is c. 26 m, which is in the middle Acadoparadoxides harlani Zone of the Braintree Member (‘Wester Cove Member’ of Fletcher, Reference Fletcher2006).
The Ovatoryctocara granulata spot sample (Fletcher, Reference Fletcher2006; in ‘layer H’ at Branch Cove, Fig. 6) contributes nothing to an evaluation of CIs. But, the CIs of E. roddyi and C. eli bracket the Miaolingian base in the upper K. cristata–lower E. bennetti zonal interval. There is no method to rule out the higher correlation as severe provincial differences in biotas only allow tentative correlations of the upper E. bennetti Zone (upper Ads 6), with a more robust correlation of the higher E. eteminicus Zone (Ads 7) into Iberian and Baltic middle Middle Cambrian successions (Kim et al. Reference Kim, Westrop and Landing2002; Fletcher, Reference Fletcher2006; Landing et al. Reference Landing, Webster, Bowser and Nance2023).
In the context of this qualified biostratigraphy, the U-Pb zircon geochronology in this report clearly bears upon the current conundrum: does the chronostratigraphy of this Lower–Middle Cambrian boundary interval in SE Newfoundland support the inference from SW Laurentia for a base of the Miaolingian Series ≤ 506.6 ± 0.03 Ma, or the c. 509.1 ± 0.2 Ma constraint from British Avalonia
5.b.3. Miaolingian basal date of c. 506.3 Ma
It is now apparent that in SE Newfoundland, younger U-Pb zircon depositional ages bracket the Lower–Middle Cambrian boundary interval (Fig. 10). Our 507.91 ± 0.08 Ma tuff is significantly below the boundary and just above the highest occurrence of Callavia broeggeri in the lower Brigus Formation (Fig. 3). In addition, two tuffs dated at 507.67 ± 0.08 Ma and 507.21 ± 0.13 Ma in overlying upper Brigus Formation strata bracket a reported occurrence of the latest Early Cambrian Ovatoryctocara granulata (Figs. 5, 6), although Fletcher’s (2006) report of this species has not been later confirmed (discussed above). Above the regional, composite unconformities that bracket the Easter Cove and Braintree members of the Chamberlain’s Brook Formation (Figs. 2–6), two tuffs with depositional ages of 506.34 ± 0.21 and 506.25 ± 0.07 Ma in the Kiskinella cristata and Eccaparadoxides bennetti Zones bracket the Acadoparadoxides harlani Zone. The U-Pb dates of this report thus do show that an Oryctocephalus indicus LO/Zone age at the W-Z quarry, if correlated into the lowest A. harlani Zone (Sundberg et al. Reference Sundberg, Geyer, Kruse, McCollum, Pegel’, Żylińska and Zhuravlev2016, Reference Sundberg, Karlstrom, Geyer, Foster, Hagadorn, Mohr, Schmitz, Dehler and Crossey2020; Geyer, Reference Geyer2019; Zhao et al. Reference Zhao, Jinliang, Babcock, Guo, Peng, Yin, Yang, Peng, Wang, Gaines, Esteve, Tai, Yang, Wang, Sun and Yang2019), must be younger than 507.2 ± 0.1 Ma and may be even more closely bracketed to 506.3 ± 0.1 Ma (based upon Monte Carlo sampling of the two bracketing ages). This bracket is remarkably consistent with the recently published maximum depositional age constraint of ≤ 506.6 ± 0.3 Ma for the base of the Miaolingian as locally proposed in southwestern Laurentia (Grand Canyon area) (see sections 5.b.3. and 5.b.4.).
Given the suggested geochronologic agreement between the results from SE Newfoundland and SW Laurentia, the basal Miaolingian Series age estimate of 509.1 ± 0.2 Ma (Zhao et al. Reference Zhao, Jinliang, Babcock, Guo, Peng, Yin, Yang, Peng, Wang, Gaines, Esteve, Tai, Yang, Wang, Sun and Yang2019; Cohen et al. Reference Cohen, Finney, Gibbard and Fan2013) based upon the U-Pb zircon geochronology of the ‘Comley ub’ tuff bed in Nuneaton, England (Harvey et al. Reference Harvey, Williams, Condon, Wilby, Siveter, Rushton, Leng and Gabbott2011) requires some reconsideration. Harvey et al.’s (Reference Harvey, Williams, Condon, Wilby, Siveter, Rushton, Leng and Gabbott2011) ‘Comley ub’ tuff bed lies right above the erosional Ads 4b–6 sequence boundary on the Avalonian uppermost Lower Cambrian or lowest Middle Cambrian Lapworthella Limestone (unit ‘ad'), and immediately under the lower Middle Cambrian Quarry Ridge Grits (Landing, Reference Landing, Nance and Thompson1996; Rees et al. Reference Rees, Thomas, Lewis, Hughes and Turner2014; Landing et al. Reference Landing, Keppie, Keppie, Geyer and Westrop2022; Landing et al. Reference Landing, Webster, Bowser and Nance2023; see Rushton, 1972, Fig. 12, on basal unconformity of Quarry Ridge Grits).
It is well known that the boundary interval is highly condensed at Comley, with significant disconformities and unconformities separating the constituent beds of the underlying Lower Comley Limestones. One possibility is that of an unrecognized hiatus at the base or top of the ‘Comley ub’ bentonite, separating it temporally from the Quarry Ridge Grits and their very poorly preserved Middle Cambrian fossil assemblages. A second possibility is that the protoliths to the altered bentonite, which is described as discontinuous and of variable thickness, are older reworked rip-up clasts that cap the basal transgressive conglomerates of the Quarry Ridge Grits. A third possibility is that the zircons in the ‘Comley ub’ bed are epiclastic volcanic zircons from an older tuff that were redeposited in younger sediments. Thin tuffs are common in the Avalonian uppermost Lower and Middle Cambrian (e.g. Supplementary Appendix 1), and it follows that reworking and redeposition of relatively juvenile epiclastic zircons in this epeirogenically active microcontinent may be common (e.g. Landing et al. Reference Landing, Keppie, Keppie, Geyer and Westrop2022; Landing, Westrop & Geyer, Reference Landing, Westrop and Geyer2023). In all three cases, the ‘Comley ub’ date of 509.10 ± 0.22 Ma would be interpreted as a maximum depositional age, removing any conflict with but not corroborating the younger base of the Miaolingian as interpreted in SW Laurentia and correlated with uncertainty into SE Newfoundland.
A number of reports (Harvey et al. Reference Harvey, Williams, Condon, Wilby, Siveter, Rushton, Leng and Gabbott2011; Sundberg et al. Reference Sundberg, Geyer, Kruse, McCollum, Pegel’, Żylińska and Zhuravlev2016; Geyer, Reference Geyer2019; Zhao et al. Reference Zhao, Jinliang, Babcock, Guo, Peng, Yin, Yang, Peng, Wang, Gaines, Esteve, Tai, Yang, Wang, Sun and Yang2019) presume the Comley ub’ age comes from the Acadoparadoxides harlani Zone. However, the age is likely even higher in the lower Middle Cambrian – perhaps above the A. harlani Zone, with Landing (Reference Landing, Nance and Thompson1996) correlating the unit into Ads 6. The lowest paradoxidid in Harvey et al.’s (Reference Harvey, Williams, Condon, Wilby, Siveter, Rushton, Leng and Gabbott2011) section was described as Paradoxides groomi (Lapworth) from poorly preserved, large fragments in a conglomerate (Fletcher, Reference Fletcher2006, p. 65) with ‘characters [….] very imperfect’ (Lake, Reference Lake1935, p. 209). Harvey et al. (Reference Harvey, Williams, Condon, Wilby, Siveter, Rushton, Leng and Gabbott2011) accepted Fletcher’s (Reference Fletcher2006, p. 65) synonymy of P. groomi with A. harlani, though this synonymy is a remark without discussion by Fletcher (Reference Fletcher2006), The specimens identified as ‘P. groomi’ by Lake (Reference Lake1935) are inadequate to characterize a species. Indeed, P. groomi was not part of later discussions of A. harlani (i.e. Fletcher et al. Reference Fletcher, Theokritoff, Lord and Zeoli2005; Fletcher & Theokritoff, Reference Fletcher and Theokritoff2008), and some of Lake’s (Reference Lake1935) figured specimens certainly cannot be assigned to A. harlani. We regard P. groomi as a nomen dubium and base a more defensible correlation of Harvey et al.’s (Reference Harvey, Williams, Condon, Wilby, Siveter, Rushton, Leng and Gabbott2011) ash by recognizing the Ads 4b–6 boundary under the Quarry Ridge Grits (Landing, Reference Landing, Nance and Thompson1996; Landing et al. Reference Landing, Keppie, Keppie, Geyer and Westrop2022; Landing et al. Reference Landing, Webster, Bowser and Nance2023). This means a tuff with un-reworked zircons in the Quarry Ridge Grits should be more similar in age to the c. 506.25 ash from the Eccaparadoxides benneti zonal interval at Red Bridge Road (Figs. 3, 11).
5.b.4. Evaluating diachrony of basal Miaolingian correlation: lithofacies and palaeoenvironmental changes
Unanswered conceptual and practical problems are associated with the Miaolingian that question its utility as a global chronostratigraphic and correlation standard. In particular, the base of the Miaolingian Series at the Global Stratotype Section and Point (GSSP) in South China is defined at the ‘first appearance datum’ (FAD) of Oryctocephalus indicus (Zhao et al. Reference Zhao, Jinliang, Babcock, Guo, Peng, Yin, Yang, Peng, Wang, Gaines, Esteve, Tai, Yang, Wang, Sun and Yang2019, pp. 168, 173; discussed in 5.b.1 as a Lowest Occurrence). However, the GSSP level is further described (Zhao et al. Reference Zhao, Jinliang, Babcock, Guo, Peng, Yin, Yang, Peng, Wang, Gaines, Esteve, Tai, Yang, Wang, Sun and Yang2019, pp. 168, 173, 177) as linked to significant environmental changes including ‘peak negative values’ in δ 13C (and thus dysoxia) and a ‘major eustatic transgression’ in South China. These environmental changes are accompanied by abrupt biotic changes shown by trilobites and agnostids (17 species disappear, 9 persist, 7 LOs) and acritarchs (5 species disappear, 9 persist, 18 LOs) at the O. indicus LO. The significance of these environmental and ecological changes versus evolutionary emergence and turnover for controlling the taxonomic composition across the GSSP may be debated but are certainly associated with the GSSP section.
The base of the Oryctocephalus indicus Zone and O. indicus’ LO has been considered to be lithofacies-linked and associated with low oxygen facies (Landing, Reference Landing2012, section 4.3.1). This association is clear in SW Laurentia (SE California and SW Nevada) with the highest olenellids in carbonate platform facies (Mule Springs Formation) disappearing and being replaced by new taxa in overlying siliceous mudstone and carbonate (lower Monola Formation), and with 85% of lower Monola taxa disappearing with the LO of O. indicus Zone taxa in dark grey shale (e.g. Sundberg & McCollum, Reference Sundberg and McCollum2003; Sundberg, Reference Sundberg2018, Figs. 2, 4; Fig. 11). The Lower–Middle Cambrian boundary grey shales with O. indicus faunas also likely mark onlap in the inner-detrital belt further east in Nevada (e.g. Webster et al. Reference Webster, Gaines and Hughes2008; Landing, Reference Landing2012). Another example of the O. indicus biofacies tracking lithofacies is in Spiti, India, where the biofacies appears with transgression of dysoxic facies over an unconformity, extends through 6 m of dark mudstone and disappears in overlying more oxic facies (Singh et al. Reference Singh, Singla, Bhargava, Kaur and Stopden2020).
A lithofacies-biofacies association is apparently even shown at the GSSP of the Miaolingian Series and Wuliuan Stage. A uniform lithofacies through the GSSP is claimed (e.g. Gaines et al. Reference Gaines, Mering, Zhao and Peng2011; Zhao et al. Reference Zhao, Jinliang, Babcock, Guo, Peng, Yin, Yang, Peng, Wang, Gaines, Esteve, Tai, Yang, Wang, Sun and Yang2019), but variability is shown by bundles of dark and light mudstone laminae and vertical and lateral soft-sediment deformation and syndepositional thrusting (Sundberg et al. Reference Sundberg, Zhao, Yuan and Lin2011; Sundberg, Reference Sundberg2018, Fig. 3a). Even more significant lithofacies variability through the GSSP interval is shown by Zhao et al.’s (Reference Zhao, Jinliang, Babcock, Guo, Peng, Yin, Yang, Peng, Wang, Gaines, Esteve, Tai, Yang, Wang, Sun and Yang2019, Fig. 4c) picture of unweathered mudstone in the Wuliu-Zengjiayan (W-Z) quarry that can be matched with Sundberg et al.’s (Reference Sundberg, Zhao, Yuan and Lin2011, Fig. 4) graphic section. This comparison suggests the GSSP is in mudstones referable to ‘Logan cycles’ – asymmetrical, mesoscale (1–5 m) redox-calcareous alternations described on the NE Laurentian Ordovician slope and interpreted as Milankovitch alternations (e.g. Landing et al. Reference Landing, Webster, Bowser and Nance2023, with references). A complete Logan cycle is an upward succession of anoxic black mudstone (A-unit), grading into laminated greenish mudstone with sparse burrows (B-unit), and an upper C-unit of brownish, dolomitic mudstone with abundant burrows (Landing et al. Reference Landing, Benus and Whitney1992; Landing Reference Landing2007, Reference Landing2012; Landing & Webster, Reference Landing, Webster, Grover and Mango2018). The GSSP interval (Zhao et al. Reference Zhao, Jinliang, Babcock, Guo, Peng, Yin, Yang, Peng, Wang, Gaines, Esteve, Tai, Yang, Wang, Sun and Yang2019, Fig. 4c; Sundberg et al. Reference Sundberg, Zhao, Yuan and Lin2011, Fig. 3a) has green-grey mudstone (0–1.55 m) comparable to a B-unit, overlying brownish mudstone (1.55–1.9 m, C-unit), and then interbedded black and green-grey mudstone (1.9–3.1 m) comparable to a transitional A–lower B-unit. The Oryctocephalus indicus LO and Miaolingian GSSP are low in the interbedded black and green-grey and mudstone.
Thus, the local range of faunas characterized by an Oryctocephalus indicus-aspect species (see next section) comprises a traditional biostratigraphic zone, but may also represent a biofacies (see Ludvigsen et al. Reference Ludvigsen, Westrop, Pratt, Tuffnell and Young1986) that locally appears with onlap of a probable dysoxic slope water mass (Landing, Reference Landing2012). The seven ‘new’ trilobite species at the GSSP horizon, including O. indicus, have to be considered to be pre-existing (i.e. pre-GSSP) species that locally appeared with shelf onlap of a deeper-water O. indicus assemblage – thus, the local presence and lowest occurrence of an O. indicus biofacies may not have any decisive geochronologic significance if driven by local onlap (i.e. epeirogenic subsidence).
5.b.5. Evaluating diachrony of the basal Miaolingian correlation: taxonomy and species distribution
The use of the Oryctocephalus indicus’ LO to define a Cambrian series’ base and relying on it for global correlation require geochronologic and chemostratigraphic confirmation. Obviously, the correct identification of the species is required. Arguably, the diagnosis of O. indicus is problematical. It was first defined from Himalayan India from distorted specimens, while most of its cranidia (and other sclerites) from the GSSP section are visibly taphonomically distorted (e.g. Sundberg et al. Reference Sundberg, Zhao, Yuan and Lin2011, Fig. 12; Esteve et al. Reference Esteve, Zhao and Peng2017; Esteve et al. Reference Esteve, Zhao, Maté-González, Gómez-Heras and Peng2018, Fig. 1; Zhao et al. Fig. 6). This distortion likely took place with dissolution in a mudstone that underwent slumping and folding on a palaeoslope and may also be tectonic (e.g. Sundberg et al. Reference Sundberg, Zhao, Yuan and Lin2011).
Multiple species of an Oryctocephalus indicus-plexus may occur in the GSSP succession based on varying numbers of transglabellar furrows (1–3) in adult (holaspid) specimens (e.g., Zhao et al. Reference Zhao, Jinliang, Babcock, Guo, Peng, Yin, Yang, Peng, Wang, Gaines, Esteve, Tai, Yang, Wang, Sun and Yang2019). The nearly 100% presence of three furrows in Laurentian collections (Sundberg et al. Reference Sundberg, Zhao, Yuan and Lin2011; Zhao et al. Reference Zhao, Jinliang, Babcock, Guo, Peng, Yin, Yang, Peng, Wang, Gaines, Esteve, Tai, Yang, Wang, Sun and Yang2019) and other reported occurrences of O. indicus suggests a different species. The Siberian O. reticulatus was synonymized with O. indicus (Zhao et al. Reference Zhao, Yuan, Peng, Yang, Peng, Lin and Guo2006; Esteve et al. Reference Esteve, Zhao and Peng2017). This synonymy has been questioned by Geyer and Peel (Reference Geyer and Peel2011) who note it has had the result of making O. indicus a seemingly very widespread taxon useful in a GSSP definition.
In addition to distortion and varying transglabellar furrow number is a claim that lower GSSP collections show a ‘primitive’ morphotype with two pairs of pygidial spines that is succeeded in higher collections by an ‘advanced’ morphotype with three spines (Zhao et al. Reference Zhao, Jinliang, Babcock, Guo, Peng, Yin, Yang, Peng, Wang, Gaines, Esteve, Tai, Yang, Wang, Sun and Yang2019, p. 171). This ‘advanced’ morphotype is coeval with SW Laurentian (California and Nevada) collections according to Zhao et al. (Reference Zhao, Jinliang, Babcock, Guo, Peng, Yin, Yang, Peng, Wang, Gaines, Esteve, Tai, Yang, Wang, Sun and Yang2019). However, this argument and resultant correlation is based on a process-based approach to species recognition that relies on a constructed evolutionary narrative. Such approaches have no place in modern biosystematics (e.g. Westrop & Adrain, Reference Westrop and Adrain2016, p. 397, with a critique of process-based approaches). A sceptical viewpoint is that the SW Laurentian ‘O. indicus’ is not conspecific with and may differ significantly in age from the form termed ‘O. indicus’ at the W-Z GSSP horizon.
Even the purported great palaeogeographic extent of reported Oryctocephalus indicus is problematical if compared with modern marine invertebrates. The great circle distance of O indicus’ reports along the Cambrian tropics (South China–North Greenland) is c. 120° (see Landing et al. Reference Landing, Geyer, Brasier and Bowring2013, Fig. 3), a distribution known in only 0.035% of marine invertebrates (Foote & Miller, Reference Foote and Miller2013). Thus, the distribution of a single Oryctocephalus species across a third of the tropical marine realm was unlikely, even if correctly identified. This argument can be countered by proposing that O. indicus could have had a wide distribution if it had a nektic or pelagic habit – which is unlikely. The overall form of Oryctocephalus with very dorsoventrally compressed body and a wide, plate-like anterior margin had very high drag coefficients. These features differ from the more laterally compressed body shape with a small, sometimes cylindrical frontal area (‘head’) present in actively swimming invertebrate and vertebrate taxa that may be geographically widespread and nektopelagic (e.g. Vogel, Reference Vogel1981, chapters 5, 7).
5.b.6. Evaluating diachrony of basal Miaolingian correlation: chemostratigraphy
Given apparent problems with taxonomy and lithofacies control of Oryctocephalus indicus Zone associations, non-biostratigraphic (i.e. radioisotope or chemostratigraphic) correlation could provide a more robust basis for highly resolved interprovincial correlation of successions where O. indicus is reported. This approach is taken by Lin et al. (Reference Lin, Sundberg, Jiang, Montañez and Wotte2019) who provide a chemostratigraphic (carbon isotope) basis for the correlation of the LO of O. indicus in Nevada and South China sections, including the Miaolingian GSSP. Lin et al.’s (Reference Lin, Sundberg, Jiang, Montañez and Wotte2019) synthesis is puzzling because the South China GSSP has a ‘stepwise decline [in δ 13Ccarb values] …. marked by peak negative values’ at the LO of O. indicus (Zhao et al. Reference Zhao, Jinliang, Babcock, Guo, Peng, Yin, Yang, Peng, Wang, Gaines, Esteve, Tai, Yang, Wang, Sun and Yang2019, p. 177, and references therein, italics added). However, in contrast, Lin et al. (Reference Lin, Sundberg, Jiang, Montañez and Wotte2019) show peak positive values at the LO of the purported O. indicus Zone in Nevada.
Lin et al. (Reference Lin, Sundberg, Jiang, Montañez and Wotte2019) divided the Miaolingian boundary interval into successive chemostratigraphic intervals (N1–N5) defined by δ 13Ccarb trends (i.e. N1 and lower N4, rising values; N2 and N3, falling and then rising values; N5, stable). The five intervals are correlated by their chemostratigraphic signatures between Nevada and South China (Lin et al. Reference Lin, Sundberg, Jiang, Montañez and Wotte2019, Figs. 7, 8). Significantly, the top of the N2 excursion interval in South China and Nevada seems to be synchronous in both regions as it marks comparable developments in trilobite biotas with disappearance of the ‘characteristic’ upper Lower Cambrian taxa Bathynotus in both regions and Olenellus in Nevada and Redlichia in South China.
However, Lin et al. (Reference Lin, Sundberg, Jiang, Montañez and Wotte2019) rely on the O. indicus’ LO as the decisive tie-line for precise Nevada–South China correlation rather than the chemostratigraphic correlation. The consequence of biostratigraphic correlation is that they (p. 11, 15) show that the O. indicus’ LO ‘occurs much higher’ [i.e. base of N4 in Nevada] but lower just above ‘the major N2 excursion’ in South China. They explain this supposed diachroneity by proposing that it reflects the absence of N3 and N4 in South China and that it ‘impl[ies] stratigraphic condensation, a hiatus and/or environmental changes in the South China sections.’ However, they do not note physical evidence of any of these implied factors in sections lying c. 30 km along the South China palaeoslope.
The simplest explanation based on comparable chemostratigrapic signatures and the disappearance of ‘characteristic’ upper Lower Cambrian trilobite genera in N2 (noted above) is that the LO of ‘O. indicus’ simply occurs higher in N4 in Nevada and that the N3 and N4 signatures are present, albeit subdued, in South China (e.g. Lin et al. Reference Lin, Sundberg, Jiang, Montañez and Wotte2019, Fig. 8). As an aside, a ‘hiatus’ should rule out continued use of the Miaolingian GSSP as a global standard by the ICS and IUGS (Cowie et al. Reference Cowie, Ziegler, Boucot, Bassett and Remane1986). We emphasize that reliance on presumed synchroneity of a taxon’s LO in intercontinental correlation is an obvious assumption (discussed above), particularly when the correlation can be tested by non-biostratigraphic methods. The interpretation, that the ‘high’ and ‘low’ O. indicus’ LO in Nevada and South China, respectively, shows diachrony, was not considered by Lin et al. (Reference Lin, Sundberg, Jiang, Montañez and Wotte2019) although it seems to be demonstrated by chemostratigraphy. Diachrony as the best interpretation of Nevada–South China chemostratigraphic correlation further accords with Zhao et al.’s (Reference Zhao, Jinliang, Babcock, Guo, Peng, Yin, Yang, Peng, Wang, Gaines, Esteve, Tai, Yang, Wang, Sun and Yang2019, p. 177) conclusion that an ‘advanced’ O. indicus morphotype is the lowest form of the ‘species’ in Nevada. Thus, an alternative palaeobiological interpretation is that a different cryptospecies of the O. indicus-plexus appears with onlap of a dysoxic water mass in Nevada that is younger than the appearance of similarly dysoxic bottom water at the Miaolingian GSSP in South China.
Diachrony of the South China GSSP and the Nevada LO of purported O. indicus is further corroborated by work in the Stansbury Basin, South Australia. Here, Birksmith et al. (Reference Birksmith, Brock, Betts, Holmes and Zhang2023) use the strongly negative ROECE δ 13C excursion as one basis for correlation of the LO of I. indicus at the GSSP (see Zhao et al. Reference Zhao, Jinliang, Babcock, Guo, Peng, Yin, Yang, Peng, Wang, Gaines, Esteve, Tai, Yang, Wang, Sun and Yang2019) into South Australia. This report further emphasizes that the strongly positive δ 13C at the base of the purported ‘O. indicus’ assemblage in Nevada (Lin et al. Reference Lin, Sundberg, Jiang, Montañez and Wotte2019) shows the SW Laurentian assemblage is younger than the GSSP horizon.
Diachrony of the LO of the Oryctocephala indicus Zone/biofacies in Nevada compared with South China and South Australia seems consistent with epeirogenic, not eustatic, control of the onlaps of dysoxic water. Such epeirogenic control in Nevada may reflect brachyseismic activity with rift-related faulting and volcanism through much of the Early Cambrian of SW Laurentia (e.g. Smith et al. Reference Smith, Nelson, O’Connell, Eyster and Lonsdale2023). Eustratic control in the Early–Middle Cambrian boundary interval on the onlap of dysoxic OMZ water cannot be invoked as this time interval seems to show modest eustatic rise (e.g. Haq & Schutter, Reference Haq and Schutter2008). More recent work suggests the eustatic fall–rise couplet termed the ‘Hawke Bay event’ through the Lower–Middle Cambrian boundary must be reevaluated as showing continued, albeit slow, rise that began as early as the Avalonian Callavia broeggeri Chron (Landing & Webster, Reference Landing, Webster, Grover and Mango2018; Landing et al. Reference Landing, Webster, Bowser and Nance2023).
5.b.6. Interregional correlation of Miaolingian
In short, precise radioisotopic age or robust chemozone correlation offers hope for quantifying the possible diachrony of Oryctocephalus indicus Zone/biofacies assemblages. The aforementioned caveats to interregional correlation introduce uncertainty into this exercise. Nonetheless, our new robust radioisotopic calibration of Avalonian strata of SE Newfoundland provides a numerical age for a level correlated to the LO of reported O. indicus in Nevada of 506.3 ± 0.1 Ma. This age is fully consistent with a detrital zircon maximum depositional age constraint of ≤506.6 ± 0.3 Ma in the basal sheet sandstones of the Sauk II transgression below the LO of O. indicus in the overlying Pioche Shale. This first tie point between independently dated successions on two palaeocontinents appears to constrain the potential diachrony of O. indicus Zone/biofacies assemblages to 0.3 +0.6/−0.3 Ma, within the current limitations of biostratigraphic correlation. However, much more taxonomic, chemostratigraphic and radioisotopic work is needed to confirm and refine this conclusion.
5.c. Geochronologic brackets on Avalonian epeirogeny, deposition, and volcanism
An increasing number of U-Pb dates from Avalonia begin to allow for precise determinations of the timing of epeirogenic activity and volcanism and the rates of deposition on the Avalonian platform. Several results from this study may be discussed.
5.c.1. Age of onlap of Ads 4a
Ads 4a, which includes the Callavia broeggeri Zone, had a strongly diachronous transgression across Avalonia, and the depth of sub-Ads 4a erosion varies greatly across the Avalonian platform. As detailed elsewhere (Landing, Reference Landing, Nance and Thompson1996; Landing et al. 2015, Reference Landing, Keppie, Keppie, Geyer and Westrop2022, and references therein), the oldest part of the Avalonian trilobite-bearing Cambrian is significantly younger than an unconformably underlying lower Lower Cambrian (Fortunian) massive quartzite on the marginal platform (i.e. St. Non’s Formation, South Wales; Random Formation, Burin Peninsula, SE Newfoundland). The age of the overlying trilobite-bearing Ads 4a on the massive quartzite may be as great as c. 519 Ma (Caerfai Bay) in North Wales (Landing et al. Reference Landing, Geyer, Brasier and Bowring2013) or as young as c. 507.91 with onlap of the upper St. Mary’s Member across the Random Formation in the Burin Peninsula (e.g. Landing & Westrop, Reference Landing and Westrop1998a).
On the inner platform, a relatively thick (locally 12 m) sub-trilobitic limestone unit is locally the cap of Ads 3 (Fosters Point Formation in Avalonian North America; Home Farm Limestone, Nuneaton, English Midlands). This unit has a maximum age of c. 520 Ma given its biostratigraphic (small shelly fossil) and carbon isotope correlation into the lower but not lowermost trilobite-bearing strata in Siberia (lower Atdabanian Stage, carbon isotope excursion IV) (e.g. Landing & Kouchinsky, Reference Landing and Kouchinsky2016; Landing et al. Reference Landing, Geyer, Schmitz, Wotte and Kouchinsky2021). The age of transgression of Ads 4a across Ads 3 is as great as c. 517 Ma at Nuneaton (Woodlands Member and Purley Shales Formation) while Ads 3–4a contacts on western parts of the inner platform in SE Newfoundland, the northern Antigonish Highlands, and Cape Breton Island would be c. 507.7 Ma (e.g. Landing et al. Reference Landing, Keppie, Keppie, Geyer and Westrop2022, and references therein). By this interpretation, the oldest date (c. 517 Ma) would correspond to onlap on the marginal platform shortly after deposition and erosion of Ads 3.
5.c.2. Epeirogenic rates of Ads 4b–6
By comparison with a seemingly languid rate of epeirogenic activity in Ads 4a (the c. 9.5 Ma duration of the Callavia broeggeri/Callavia Zone), terminal Early–early Middle Cambrian epeirogenic activity, and consequently activity on the Avalonian transform fault, was rapid. A much shorter time interval of c. 700 ka spanned offlap, onlap and deposition of Ads 4b (Jigging Cove Member) (depositional ages of 507.9 Ma in the upper St. Mary’s Member and 507.2 Ma in the upper Jigging Cove Member).
The Jigging Cove–Easter Cove Member and Avalonian Lower–Middle Cambrian boundary unconformity in the stratigraphically ‘most complete’ sections at Branch and Redland coves shows a modest amount of differential erosion (5 m; Fig. 6) on the southern St. Mary’s-east Trinity axis. However, the depositional ages of 507.2 on the upper Jigging Cove Member (Ads 4b) and 506.3 Ma in the lower Easter Cove Member (Ads 5) show an unexpectedly long, c. 900 ka, hiatus. As might be expected, a far longer duration of the composite Lower–Middle Cambrian boundary is present in eastern Conception Bay at Red Bridge Road with the St. Mary’s Member–Braintree Member hiatus bracketing c. 1.65 Ma (depositional ages of c. 507.9 on RBBR-26.5 and c. 506.25 on RBCB-1.2).
A rapid rate of epeirogeny and biotic overturn is shown in the Ads 5–lower 6 interval. This included Ads 4b–5 offlap and Ads 5 deposition (end of Moroconus-Condylopyge eli Assemblage interval) to Kisknella and lower Acadoparadoxides harlani zones, i.e. Appendix 1, loc. Bn) followed by Ads 5–6 onlap and the onset of Ads 6 deposition (A. harlani and lower Eccaparadoxides bennetti zones). This dynamic history occurred within the c. 900 ka resolution of tuff ages through this interval (Fig. 11). In the future, we anticipate that the determination of dates on tuffs from the Manuels River Formation in SW New Brunswick is a way to estimate rates of epeirogenic activity into Ads 8 (Fig. 2).
5.c.3. Late Early Cambrian volcanic pulse and unity of Avalonia microcontinent
Successions bracketing the Lower–Middle Cambrian boundary interval (i.e. Ads 4b–6, Figs. 2–4) are present on the marginal and inner platform from Rhode Island–SW New Brunswick–SE Newfoundland, North and South Wales, and English Midlands and extend into central Belgium (e.g. Landing, Reference Landing, Nance and Thompson1996; Landing et al. Reference Landing, Webster, Bowser and Nance2023, Fig. 2). Unfortunately, the boundary interval is accessible in relatively few sections. This reflects limited exposures in densely vegetated areas away from coastal outcrops, erosional non-sequences that truncate the uppermost Lower Cambrian (Ads 4b), and the restriction of the uppermost Lower Cambrian to elongate, fault-bounded syndepositional basins as in SE Newfoundland (Figs. 1, 2, see Placentia–Bonavista and St. Mary’s–East Trinity axes). Despite limitation to several coastal sections, the uppermost Jigging Cove Member in SE Newfoundland shows one or several successive tuffs, whereas they have not been noted in the lower Jigging Cove. These upper Jigging Cove Member and lower Chamberlain’s Brook Formation tuffs are present at Smith Point and Branch and Redland coves (Supplementary Appendix 1).
Well to the SW, the Bourinot Belt in Cape Breton Island is brought to Avalonia, not a ‘Gander terrane’ (e.g. contra van Rooyen et al. Reference van Rooyen, Barr, White and Hamilton2019), based on a typical Avalonian Middle Cambrian–Tremadocian cover sequence (e.g. Landing, Reference Landing, Nance and Thompson1996; Landing et al, Reference Landing, Keppie, Keppie, Geyer and Westrop2022; Landing et al. Reference Landing, Webster, Bowser and Nance2023). The extensional Eskasoni volcanics in Cape Breton underlie a typical Avalonian cover sequence and have been regarded as a volcanic edifice on the NW edge (in modern coordinates) of the Avalonian marginal platform (e.g. Landing et al. Reference Landing, Johnson and Geyer2008; Landing et al. Reference Landing, Keppie, Keppie, Geyer and Westrop2022, section 8.7, with references). The 505 ± 3 Ma late Early–middle Middle Cambrian age on the Eskasoni volcanics (compare Landing et al. 2019) makes them possibly coeval with the latest Early Cambrian tuffs documented in this report in SE Newfoundland (Ads 4b). This correlation is strengthened by upper Lower Cambrian acritarchs in the Eskasoni Formation that show the unit lies in the oldest part of this age bracket (Palacios et al. Reference Palacios, Jensen, White and Barr2012). Thus, the age of Eskasoni volcanism may be compatible with the epeirogenic activity that led to the c. 507.91 Ma volcanism of the upper Jigging Cove Member in SE Newfoundland (Landing et al. Reference Landing, Webster, Bowser and Nance2023, see ‘Marginal Platform, Cape Breton Island,’ and references).
Another link in late Early Cambrian volcanism on the Avalonian marginal platform is at Beaver Harbour, SW New Brunswick, where the Waites Lane volcanic edifice shows extensional late Early Cambrian volcanism (Landing et al. Reference Landing, Johnson and Geyer2008, and references therein). The Waites Lane volcanics unconformably underlie the fossiliferous middle Middle Cambrian Fossil Brook Member (Ads 7) and are laterally equivalent to upper Lower Cambrian tuffs in the upper Hanford Brook Formation (Ads 4b) dated at 508 Ma ± 1.0 Ma 60 km to the NE in Saint John, New Brunswick (Isachsen et al. Reference Isachsen, Bowring, Landing and Samson1994; Schmitz, Reference Schmitz, Gradstein, Ogg, Schmitz and Ogg2012, age recalculation; Landing et al. Reference Landing, Keppie, Keppie, Geyer and Westrop2022; Landing et al. Reference Landing, Webster, Bowser and Nance2023).
This review of known late Early Cambrian volcanics and associated extensional activity further emphasizes coeval volcanics as part of a regionally extensive cover sequence along the Avalonian marginal platform. This shared epeirogenic and consequent volcanic activity accords with a pulse of extensional activity on the Avalonian transform fault and along a unified Avalonia microcontinent (e.g. Landing et al. Reference Landing, Keppie, Keppie, Geyer and Westrop2022; Landing et al. Reference Landing, Webster, Bowser and Nance2023, and references therein). While we favour this tectonic scenario for regional patterns of Avalonian marine off- and onlap, we cannot rule out accompanying changes in eustasy across the Early–Middle Cambrian boundary, given the apparently coeval and rapid transgression of terminal Lower Cambrian sheet sands across southwest Laurentia (Karlstrom et al. Reference Karlstrom, Mohr, Schmitz, Sundberg, Rowland, Blakey, Foster, Crossey, Dehler and Hagadorn2020). However, this same time interval in NE Laurentia (eastern New York–western Newfoundland) is interpreted as showing a very slow rate of epeirogenic rise (Landing & Webster, Reference Landing, Webster, Grover and Mango2018; Landing et al. Reference Landing, Webster, Bowser and Nance2023).
5.c.4. Avalonian Lower–Middle Cambrian boundary interval and incompleteness of stratigraphic and fossil record
The succession of regionally extensive Avalonian depositional sequences (Ads) is separated by unconformities, each representing a hiatus of undetermined length (Landing, Reference Landing, Nance and Thompson1996; Landing et al. Reference Landing, Keppie, Keppie, Geyer and Westrop2022; Figs. 2, 3, 5, 6). This report provides the first evidence on the duration of individual and composite unconformities and the relative completeness of the Avalonian stratigraphic record (Figs. 10, 11).
As might be expected, the composite Ads 4a–6 unconformity at Red Bridge Road and other localities on the eastern part of the inner platform (Figs. 1, 2) shows the longest hiatus. U-Pb volcanic zircon dates a few metres above and below the Ads 4a–6 unconformity (Figs. 3, 11; ash beds RBBr-26.5 and RBCB-1.2) indicate a c. 1.66 Ma hiatus.
An associated (and surprising) result is that the cryptic Ads 4b–5 unconformity (i.e. siliciclastic mudstone on siliciclastic mudstone, Fig. 5) that more closely brackets the Avalonian Lower–Middle Cambrian boundary corresponds to an unexpectedly long (c. 1.33 Ma) hiatus at the Smith Point section (Figs. 5, 10, 11; SPBr-105.75 and SPCB-4.4 ashes). However, the top of the preserved Avalonian uppermost Lower Cambrian (Ads 4b) is quite diachronous, apparently reflecting post-depositional erosion, with the upper Brigus Formation on the southern St. Mary’s western Trinity axis (Fig. 1) c. 0.46 Ma younger in age (Figs. 10, 11; ash horizons SPBr-105.75 and ReBr-118.5). In short, the most completely preserved and most fossiliferous Avalonian Lower–Middle Cambrian boundary sections are in SE Newfoundland (e.g. Fletcher, Reference Fletcher2006), but these sections (i.e. samples ReBr-118.5 and SPCB-4.4) show a boundary hiatus of at least 0.87 Ma. Between relatively unfossiliferous facies and a hiatus just short of a million years in length, Avalonia does not preserve insights into the global Lower–Middle Cambrian turnover in trilobite faunas. Indeed, the common statements in literature reports of a ‘continuous’ sedimentary record though various stratigraphic intervals with key biotic changes should be regarded as indicating an incomplete understanding of local sedimentary successions. Indeed, evidence suggests that more time may actually be represented by hiatuses at sequence boundaries and other unconformities and diastems than is actually recorded by the rock succession (e.g. Landing, Reference Landing2012, p. 269 and references therein).
6. Conclusions
Regional sampling of the Lower–Middle Cambrian boundary succession in SE Newfoundland led to the recovery of five dated zircon-bearing tuffs. When combined with biostratigraphy, sequence stratigraphy and other U-Pb dates from Avalonian North America and Britain, the following determinations can be made:
-
1) the fundamental accuracy of the U-Pb zircon geochronology in this report is supported by the fact that progressively younger dates are recorded on successively higher tuffs as bracketed by biostratigraphic and sequence stratigraphic criteria;
-
2) the Callavia Zone s.l. had a very long duration of c. 9.5 Ma, e.g. from c. 517.2 to 507.7 Ma, which limits its biostratigraphic utility; by contrast the post-Callavia Zone Lower Cambrian (Ads 4b) lasted ≤ 1.4 Ma, e.g. from ≤ c. 507.7 to ≥ c. 506.3 Ma;
-
3) the Miaolingian base, within the limitations of its biostratigraphic correlation into Avalonia (SE Newfoundland), is constrained to ≤ 507.2 to ≥ 506.3 Ma, which is remarkably consistent with the maximum depositional age (mda) of ≤ 506.6 ± 0.3 Ma for the base of the Miaolingian as interpreted in southwestern Laurentia (Grand Canyon area).
-
4) the 509.1 ± 0.2 Ma date from the English Midlands formerly used for the age of the base of the Miaolingian is problematical with respect to the results from SE Newfoundland and SW Laurentia, and a recalculated c. 508 Ma date on the upper Lower Cambrian of New Brunswick, and may be biased by sedimentary and/or epiclastic reworking;
-
5) the geochronologic significance of the LO of faunas assigned to the Oryctocephalus indicus Zone/biofacies and definition of the base of the Miaolingian Series should be reevaluated given concerns that shelf occurrences of the eponymous taxon may represent a species-plexus linked to diachronous local onlaps of dysoxic water. This study is among the first to apply radioisotope correlation to constrain the potential diachrony of O. indicus Zone/biofacies assemblages to 0.3 +0.6/−0.3 Ma, within the current limitations of biostratigraphic correlation.
-
6) An alternative view (see Acknowledgments) is that the confidence interval of the few ‘typical,’ Laurentian upper Lower Cambrian trilobites known in SE Newfoundland extends as high as the traditional Avalonian lower Middle Cambrian Eccaparadoxides bennetti Zone, which yielded a 506.25 ± 0.2 Ma mda date indistinguishable from the ≤ 506.6 ± 0.3 Ma age proposed for the base of the Miaolingian proposed in SW Laurentia.
-
7) rates of epeirogenic activity along the Avalonian transform fault, constrained by marine offlap and onlap, were slow during much of the Early Cambrian, whereas subsequent terminal Early Cambrian–early Middle Cambrian activity was exceptionally rapid, suggesting fundamental tectonic reorganization of Avalonia that obscures evidence of coeval eustatic changes.
Supplementary material
The supplementary material for this article can be found at https://doi.org/10.1017/S0016756823000729
Acknowledgements
The development of scientific explanations commonly involves divergent models (Kuhn, Reference Kuhn1962). In providing the first precise U-Pb zircon geochronology through the Avalonian Lower–Middle Cambrian boundary interval, we note that the controversy over Oryctocephalus indicus’ systematics extends to the authors of this paper. MDS favours an interpretation in which a single species defines an essentially isochronous base of the Miaolingian Series in SW Laurentia and South China. EL, GG and SRW consider ‘O. indicus’ represents at least two species, and only the younger of these is represented in SW Laurentia, as shown, in particular, by chemostratigraphic correlation. Funding for the analytical infrastructure of the Boise State Isotope Geology Laboratory was provided by NSF grants EAR-0521221 EAR-1337887 and EAR-0824974 to MS. Support for Cambrian chronostratigraphy was provided by the New York State Museum and by NSF grant EAR-1954583 to M.S. Much of E.L.’s field and laboratory work was done under National Science Foundation grant support while at the New York State Museum, in collaboration with S. A. Bowring (1953–2019) of the Massachusetts Institute of Technology. G.G.’s field work was supported by research grant GE 549/13-1 of the Deutsche Forschungsgemeinschaft; the preparation of the manuscript was made possible by research grant GE 549/22-1. S. Jensen and an anonymous reviewer are thanked for their comments. This report is dedicated to Dr. David B. Nash (1949–2023, University of Cincinnati, ret.)