Introduction
What lives is destined to die. Ageing is unavoidable for all life, but underlying mechanisms are still not fully understood. However, what all life forms have in common is the intertwining genetic and environmental elements that influence lifespan, and seeds are no exception. Seeds can be broadly categorized into two groups regarding their tolerance to desiccation, which predominantly influences seed longevity. Orthodox seeds, such as grains and those from most other crops, tolerate desiccation and suspend metabolism, thus have extended lifespans, whereas recalcitrant seeds, such as large-seeded temperate and tropical tree species, are desiccation intolerant and generally do not survive beyond 1 year (Ellis, Reference Ellis1991; Berjak and Pammenter, Reference Berjak and Pammenter2008). The extended longevity of orthodox seeds is critical for standard agricultural practices, but also enables the conservation of germplasm in seed banks. In the desiccated state, orthodox seed longevity is strictly influenced by temperature and seed moisture content (MC), with a reduction in either of these two abiotic components extending the seed life span (Ellis and Roberts, Reference Ellis and Roberts1980). Hence, to extend longevity over decades to centuries, seed banks store seeds equilibrated to low relative humidity (RH) at low temperatures. Inversely, for investigating ageing mechanisms in shorter-term research projects, seeds are aged much faster over days to months in protocols called ‘controlled deterioration’ or ‘accelerated ageing’ (i.e. 60–100% RH and 35–50°C).
At a sufficiently low temperature and MC (≤0.1 g H2O g−1 dry weight (DW) and 20°C), the cytoplasm in seeds solidifies into a non-crystalline matrix, described as ‘glassy’. The glassy cytoplasm restricts molecular diffusion, thus extending longevity via decelerating the rates of biochemical reactions implicated in seed deterioration (Walters et al., Reference Walters, Hill and Wheeler2005a; Buitink and Leprince, Reference Buitink and Leprince2008; Ballesteros and Walters, Reference Ballesteros and Walters2011). At the higher MC and temperature of rapid ageing protocols, the cytoplasm is no longer glassy, and long-distance molecular mobility is enabled (Ballesteros et al., Reference Ballesteros, Pritchard and Walters2020). Although the cytoplasm is still highly viscous at approximately <0.3 g H2O g−1 DW and 20°C, the enzyme-dependent xanthophyll cycle occurred in lichen at 0.17 g H2O g−1 DW and 20°C (Candotto Carniel et al., Reference Candotto Carniel, Fernandez-Marín, Arc, Craighero, Laza, Incerti, Tretiach and Kranner2020). Moreover, changes in primary metabolite profiles provided the evidence of aldo-keto reductase and glutamate decarboxylase activities in seeds aged at 80% RH and 45°C, in which cells had a viscous, but still fluid, cytoplasm (Gerna et al., Reference Gerna, Ballesteros, Stöggl, Arc, Seal, Marami-Zonouz, Na, Kranner and Roach2022). Therefore, rapid ageing protocols may not always lead to the same biochemical changes that occur under conditions that the majority of seeds used in agriculture or conservation are stored. For instance, the lipid phase remains stable and without signs of oxidation in seeds rapidly aged (Lehner et al., Reference Lehner, Mamadou, Poels, Côme, Bailly and Corbineau2008; Morscher et al., Reference Morscher, Kranner, Arc, Bailly and Roach2015; Roach et al., Reference Roach, Nagel, Börner, Eberle and Kranner2018; Schausberger et al., Reference Schausberger, Roach, Stoggl, Arc, Finch-Savage and Kranner2019; Gerna et al., Reference Gerna, Ballesteros, Stöggl, Arc, Seal, Marami-Zonouz, Na, Kranner and Roach2022), while lipid oxidation is clearly evident during storage at lower RH and/or temperatures (Seal et al., Reference Seal, Zammit, Scott, Flowers and Kranner2010a,Reference Seal, Zammit, Scott, Nyamongo, Daws and Krannerb; Roach et al., Reference Roach, Nagel, Börner, Eberle and Kranner2018; Wiebach et al., Reference Wiebach, Nagel, Börner, Altmann and Riewe2020; Gerna et al., Reference Gerna, Ballesteros, Stöggl, Arc, Seal, Marami-Zonouz, Na, Kranner and Roach2022; Groot et al., Reference Groot, van Litsenburg, Kodde, Hall, de Vos and Mumm2022).
The production of reactive oxygen species (ROS), such as superoxide and hydrogen peroxide, is unavoidable for all aerobic organisms (Halliwell, Reference Halliwell2006), including while desiccated (Kranner et al., Reference Kranner, Minibayeva, Beckett and Seal2010; Sano et al., Reference Sano, Rajjou, North, Debeaujon, Marion-Poll and Seo2016). ROS play major roles in seed physiology. On the one hand, they act in the cellular signalling pathways that promote germination, while on the other, they lead to oxidative damage and ageing, processes that hinder vigour, and contribute to viability loss (Bailly et al., Reference Bailly, El-Maarouf-Bouteau and Corbineau2008). Low-molecular-weight (LMW) antioxidants react with ROS, preventing oxidative damage to DNA, lipids and proteins. Crucially, in desiccated seeds, highly restricted enzyme activity results in LMW antioxidants as the only defence against ROS (Ballesteros et al., Reference Ballesteros, Pritchard and Walters2020; Gerna et al., Reference Gerna, Ballesteros, Stöggl, Arc, Seal, Marami-Zonouz, Na, Kranner and Roach2022). Water-soluble thiols, such as glutathione (GSH) and lipid-soluble tocochromanols, are considered important LMW antioxidants that maintain longevity in orthodox seeds (Kranner et al., Reference Kranner, Birtic, Anderson and Pritchard2006; Mène-Saffrané et al., Reference Mène-Saffrané, Jones and DellaPenna2010). Oxidative attack of unsaturated lipids leads to the production of peroxyl radicals, which can be scavenged by tocochromanols. These lipophilic antioxidants are divided into tocopherols (i.e. α-tocopherol or vitamin E for humans) and tocotrienols, unsaturated derivatives of tocopherols also found in seeds (Falk and Munne-Bosch, Reference Falk and Munne-Bosch2010). Glutathione is a tri-peptide, γ-glutamyl-cysteinyl-glycine, ubiquitous to all plants. In Leguminosae, the tri-peptide, γ-glutamyl-cysteinyl-β-alanine, called homo-glutathione (hGSH) is also synthesized (Klapheck, Reference Klapheck1988; Colville et al., Reference Colville, Saez, Lewis and Kranner2015). Synthesis of GSH and hGSH occurs via the precursor γ-glutamyl-cysteine (γ-GC), which also accumulates in seeds (Birtić et al., Reference Birtić, Colville, Pritchard, Pearce and Kranner2011; Colville et al., Reference Colville, Saez, Lewis and Kranner2015; Gerna et al., Reference Gerna, Roach, Stoggl, Wagner, Vaccino, Limonta and Kranner2017). As part of their antioxidant activity (e.g. electron donation to ROS), LMW thiols convert to their disulphide form such as glutathione disulphide (GSSG). When the reduction of GSSG by glutathione reductase is restricted, such as in desiccated seeds, the glutathione-based cellular redox state (E GSSG/2GSH) shifts towards more oxidized values (Kranner et al., Reference Kranner, Birtic, Anderson and Pritchard2006).
Due to time constraints on research projects, only few studies have investigated slower ageing processes that occur in ambient or cold-stored seed. Therefore, to improve understanding on seed deterioration under ambient conditions directly relevant to seed conservation and agriculture, we selected commercially important species with contrasting seed longevity and investigated relationships between seed viability, germination speed and changes in the LMW antioxidants such as glutathione and tocochromanols.
Materials and methods
Seeds
Seeds of Daucus carota (carrot var. Nantes 2, and var. Rotin), Helianthus cucumerifolius (sunflower), Phaseolus vulgaris (bean var. Brittle Wax), Cucumis sativus (gherkin var. Chinese Slangen), Raphanus sativus var. sativus (radish cv. Round – Half Red Half White), Raphanus sativus var. niger (black radish cv. Vienna Round Black), Lepidium sativum (cress) and Lactuca sativa (Lettuce var. crispa American Brown) were purchased from Austrosaat (Vienna, Austria), over 29 years since 2021, the latest harvest year available (Supplementary Table S1). Immediately after purchase, seeds were stored in original packets at an average room temperature of 21.9 ± 2.1°C and RH of 36.8 ± 6.6% (±SD), as recorded at hourly intervals for the whole of 2021 with a factory-calibrated Testo 175-H1 data logger (Testo, Belgium).
Germination tests and estimating time to 50% loss of total germination
For germination tests, C. sativus, R. sativus var. sativus, R. sativus var. niger, L. sativum and L. sativa were sown onto double thickness 85 mm Whatman number 1 filter papers, hydrated with 3 ml of deionized water in 90 mm Polystyrene Petri dishes, closed with Parafilm, and spaced out in five rows of ten seeds. For the bigger seeds of H. cucumerifolius and P. vulgaris, germination paper (grade 3644, Whatman) saturated with 40 ml of deionized water in closed transparent boxes was used. For each year of purchase, up to four replicas of 50 seeds were sown, each from different seed packets (Supplementary Table S2). Optimal stratification conditions and germination temperatures (Supplementary Table S1) were chosen according to the International Rules for Seed Testing (ISTA, 2010). Seeds were germinated in a growth chamber (Percival, PGC-6HO), with daytime light intensity for all temperatures of 50 μmol quanta m−2 s−1. Seed germination was scored for the first 5 days and then again on 8–12 days, 15–19 days, etc., for up to 6 weeks until no change in total germination (TG) occurred. For estimating the time to 50% loss of TG (P50), the TG of each species from all available years was fitted with Sigmoidal Logistic function (type 1) in OriginPro 2017G (IBM), without weighting and using the highest TG recorded as the upper limit.
Seed preparation for biochemical analyses
For biochemical analyses, seeds were weighed before and after freeze-drying for 5 days (−80°C and 0.043 mbar) to determine seed fresh weight, DW and MC. For grinding all seeds, except P. vulgaris, 2 ml reaction tubes (Eppendorf) were filled one-third with freeze-dried seeds, or for H. cucumerifolius isolated embryonic axes, and depending on seed size, with either one 7 mm agate bead (larger seeds) or two 3 mm glass beads and two 5 mm agate beads (smaller seeds). Samples were ground at −80°C for 2 min at a frequency of 30 Hz in a Tissuelyser II (Qiagen). For P. vulgaris, 20 seeds were ground in 25 ml steel grinding vessels with two 10 mm metal beads for 1 min and a frequency of 30 Hz in a Mikro-Dismembrator (Satorius). When available, individual seed packets were used as replicates (Supplementary Table S2), otherwise replicates (n = 4) of different seeds were taken from the same packet.
Analysis of LMW thiols and disulphides
Thiols and disulphides were extracted, depending upon species from between 50 and 200 mg DW in 1 ml of 0.1 M HCl, in the presence of one 5 mm and two 3 mm glass beads, for 2–4 min, as required, at a frequency of 30 Hz. Initial trials were conducted with all species to see if polyvinylpolypyrrolidone (PVPP) was necessary to measure GSH, resulting in its addition, in equal amount to seed DW, during the extraction of L. sativum and both Raphanus varieties. The ground seed extract was centrifuged for 10 min at 29,000 g at 4°C, after which 700 μl of the supernatant was transferred into a 1.5 ml reaction tube (Eppendorf), which was centrifuged for another 20 min, before analysis with an Agilent 1290 Infinity II according to the HPLC method of Bailly and Kranner (Reference Bailly and Kranner2011). Half-cell reduction potentials of thiol/disulphide redox couples (i.e. E GSSG/2GSH) were calculated according to the Nernst equation and standard half-cell redox potentials of Birtić et al. (Reference Birtić, Colville, Pritchard, Pearce and Kranner2011) and assuming pH of 7.3. Molar concentrations of thiols and disulphides were calculated from MC of the respective seeds.
Analysis of tocochromanols
For the analyses of tocochromanols, 50 mg of ground seed were placed into 2 ml reaction tubes (Eppendorf) with 800 μl of heptane and two 3 mm and one 5 mm glass bead, and shaken for 5 min at a frequency of 30 Hz. The samples were centrifuged for 20 min at 29,000 g at 4°C before injecting 20–50 μl, depending upon species, into an Agilent 1100 HPLC for separation and measurement by fluorescence (Ex: 295 nm; Ex: 325 nm) according to Schausberger et al. (Reference Schausberger, Roach, Stoggl, Arc, Finch-Savage and Kranner2019).
Results
Seed longevity under ambient storage conditions
Overall, the TG of seeds from different years of purchase, of all species, showed a sigmoidal relationship with time, agreeing with a normal distribution in seed longevity (Fig. 1). Using the function derived from the sigmoidal fit, the P50 was calculated for each species. Only D. carota var. Nantes 2 had a P50 <5 years, while L. sativa, R. sativus, L. sativum had P50 of 5–10 years, H. cucumerifolius and P. vulgaris had P50 of 5–15 years and C. sativus had P50 >15 years (Supplementary Fig. S1). In some species, such as L. sativa, C. sativus and R. sativus, TG decreased in seeds within the first years of storage, while for P. vulgaris and L. sativum, hardly any loss of TG occurred with the first 5–10 years of storage (Fig. 1). The gradient of slope of the sigmoidal fit, indicating the rate of viability loss, was also not uniform and much steeper for P. vulgaris and L. sativum, reflecting their initial resistance to loss of TG (Fig. 1). The most closely fitting sigmoidal relationship between TG and years of storage was found for L. sativa (Fig. 1E), whereas several years of deviation were observed for R. sativus var. sativus (i.e. lower than expected TG after 10 years and higher than expected after 17 years of storage) (Fig. 1C). Generally, germination speed (i.e. T20) after different durations of storage showed a positive linear relationship with TG (Fig. 1), with average R 2 values across species of 0.61 (Supplementary Table S3).
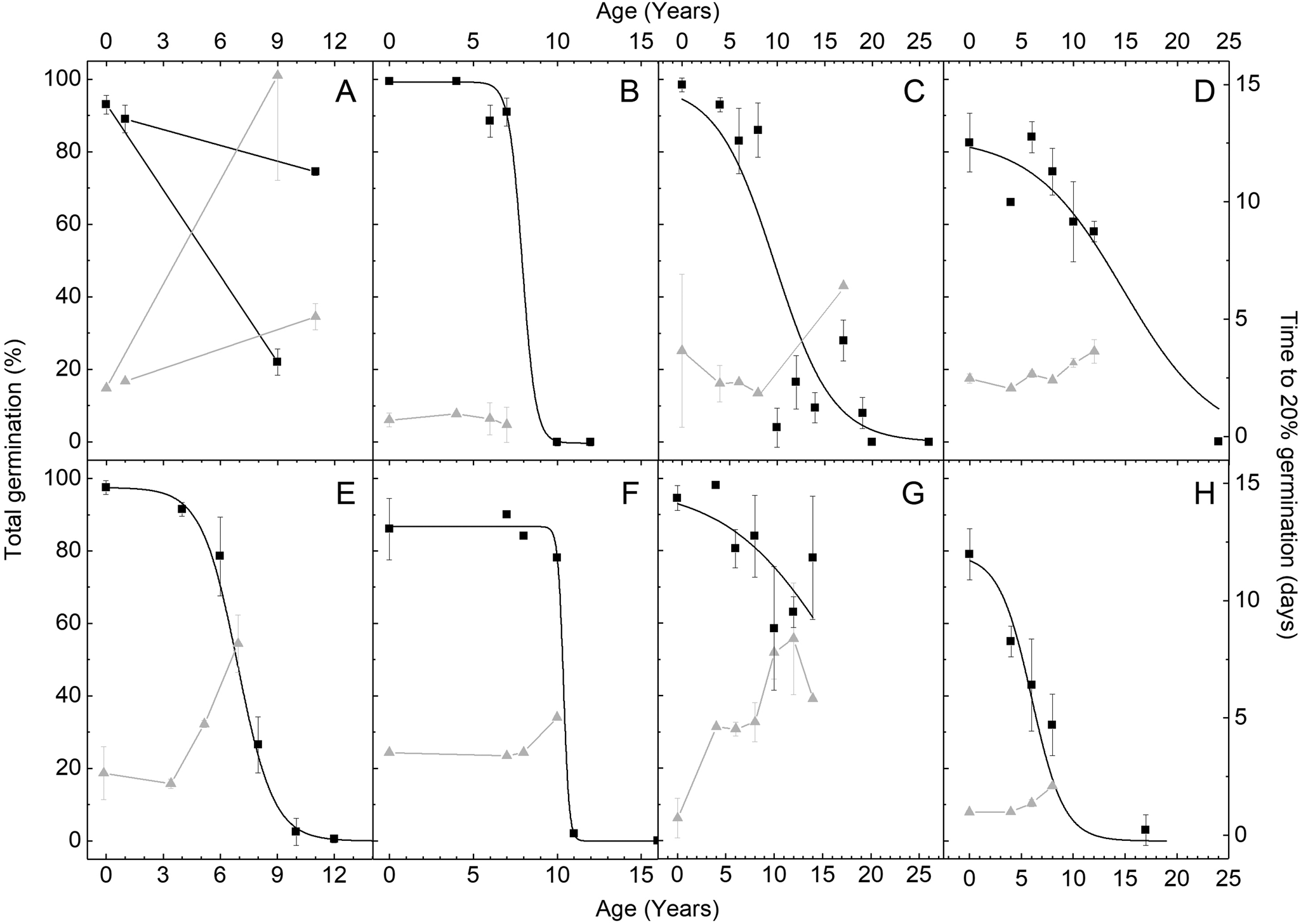
Fig. 1. Total germination and germination speed of seeds stored under ambient indoor conditions (22 ± 2°C; 37 ± 7% RH) since purchase. The left Y-axis shows average total germination (black squares) and the right Y-axis shows germination speed (grey triangles), as indicated by time it took to reach 20% germination, of (A) Daucus carota, (B) Lepidium sativum, (C) Raphanus sativus var. sativus, (D) Helianthus cucumerifolius, (E) Lactuca sativa, (F) Phaseolus vulgaris, (G) Cucumis sativus and (H) Raphanus sativus var. niger. Supplementary Tables S1 and S2 provide information on germination conditions and number of replicates. Error bars are ±SD.
Thiols and disulphides
Glutathione makes up >90% of total amount of LMW thiol and disulphides in all species, except for P. vulgaris in which 92% is γ-GC and an additional 3% is hGSH (Supplementary Fig. S2). The concentration of the most abundant LMW thiol/disulphide couple is different between seeds purchased from different years, but a consistent decline in total glutathione (GSH + GSSG) is not evident in D. carota, R. sativus var. sativus, C. sativus and H. cucumerifolius (Supplementary Fig. S3), as could be expected after storage and ageing. However, ageing effects are observable in seeds of all species regarding the relative concentrations of thiols and disulphides, indicative of oxidation during storage. Via the Nernst equation, molar concentrations of LMW thiols and disulphides enable the quantification of the potential of each redox couple (e.g. E GSSG/2GSH for glutathione), which provides an insight into cellular redox states. The range of E GSSG/2GSH values between seed lots with high TG and no TG was species-dependent (Fig. 2). For examples, E GSSG/2GSH values in non-stored seed of P. vulgaris are −155 mV, while in L. sativa are −245 mV, and in seeds with no TG are −105 and −208 mV, respectively (Fig. 2E, F). In seeds of all species, redox potentials of E γGCCGγ/2γGC parallel values of E GSSG/2GSH, although +30–50 mV higher (more oxidizing), except in P. vulgaris in which E γGCCGγ/2γGC are the most negative (Fig. 2F). Regardless, E Disulphide/2Thiol of both couples values are consistently less negative (oxidative-shifted) in seeds that had been stored for longer (Fig. 2). Correlating the E Disulphide/2Thiol values with TG revealed a negative linear relationship (Supplementary Table S3), which is the highest for L. sativa (R 2 = 0.955) and the lowest for H. cucumerifolius (R 2 = 0.321). Across species, the average R 2 value is 0.619 from all linear correlation coefficients between TG and E Disulphide/2Thiol values (Supplementary Table S3) when considering the most abundant LMW thiol/disulphide couple (glutathione and γ-GC in P. vulgaris; Supplementary Fig. S2).
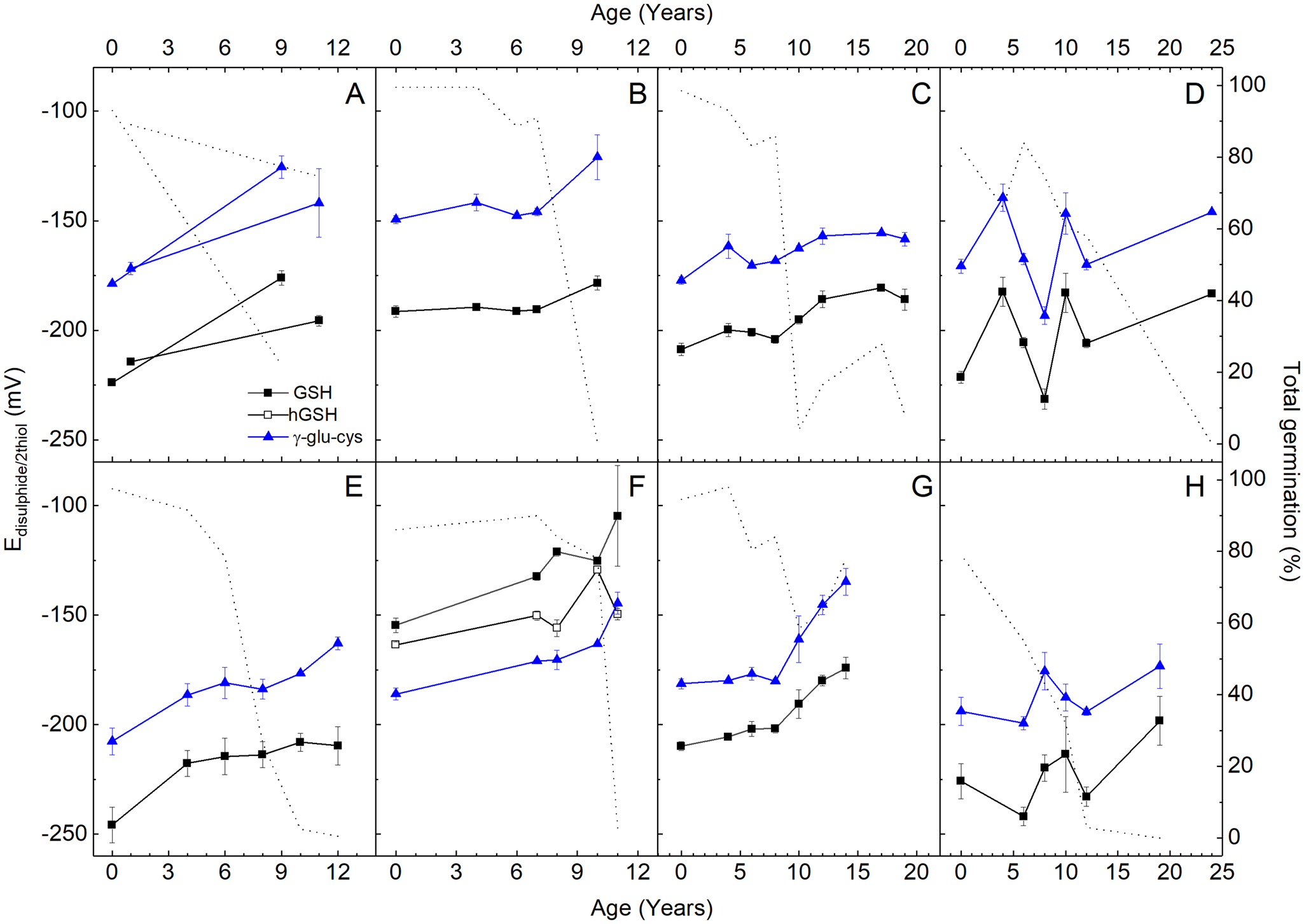
Fig. 2. Half-cell redox potentials of (homo)glutathione and γ-glutamyl-cysteine thiol/disulphide couples (E disulphide/2thiol) in dry seeds stored under ambient indoor conditions (22 ± 2°C; 37 ± 7% RH) since purchase. The left Y-axis shows redox potentials of glutathione (GSH, filled squares), homo-glutathione (hGSH, open squares) or γ-glutamyl-cysteine (γ-glut-cys, blue-filled triangles) of (A) Daucus carota, (B) Lepidium sativum, (C) Raphanus sativus var. sativus, (D) Helianthus cucumerifolius, (E) Lactuca sativa, (F) Phaseolus vulgaris, (G) Cucumis sativus and (H) Raphanus sativus var. niger, n = 4 replicates ± SD. Total germination is indicated by the dotted line (right Y-axis), as shown in Fig. 1.
Tocochromanols
Tocochromanol compositions and amounts, on a DW basis, are different between species (Fig. 3). For example, in seed lots with >50% TG, H. cucumerifolius have α-tocopherol concentrations >0.6 μmol g−1 DW (Fig. 3D), while in L. sativa they are 0.25–0.03 μmol g−1 DW (Fig. 3E). These two species are the only to contain β-tocopherol, which is at <10-fold lower concentration than α-tocopherol. In D. carota, only tocotrienols were detected, and γ-tocotrienol is the dominant form (Fig. 3A). Notably, P. vulgaris possesses very low total tocochromanol levels (<0.05 μmol g−1 DW; Fig. 3F), but like other species γ-tocopherol is the most abundant form. In R. sativus, C. sativus and L. sativum, δ-tocopherol also makes up a minor fraction of the total tocochromanol pool (Fig. 3B, C, G, H). Generally, less abundant tocochromonals weakly correlate with TG (Supplementary Table S3), whereas lower amounts of the most abundant tocochromanol are found in seeds stored for more time with low TG (Fig. 3). In R. sativus, C. sativus and L. sativum, this relationship is weakened by the low γ-tocopherol levels in seeds from 2021 before storage (Fig. 3B, C, G, H). Across species, the average R 2 value is 0.50 from all linear correlation coefficients between TG and amounts of the most abundant tocochromanol (Supplementary Table S3).
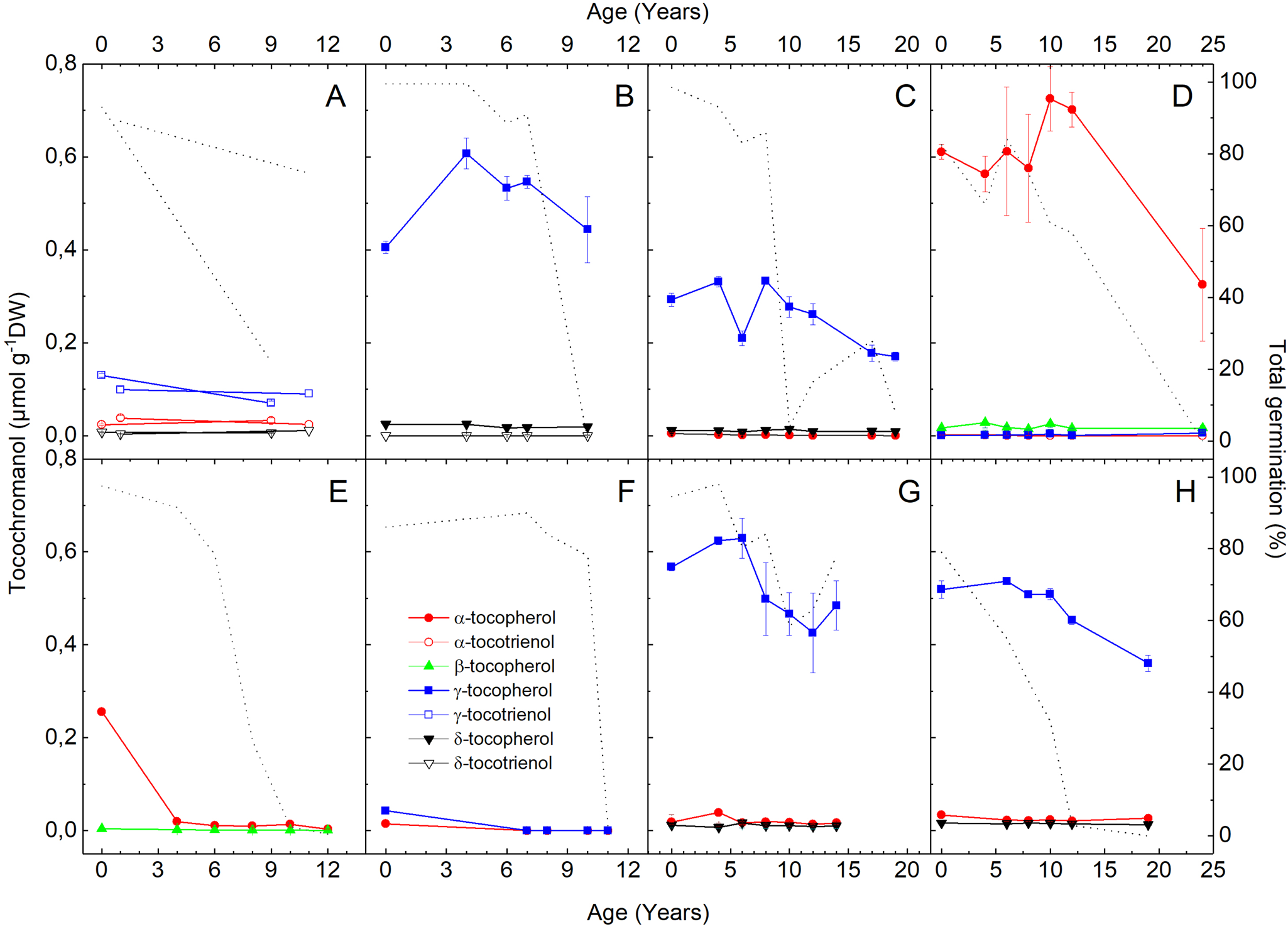
Fig. 3. Tocochromanol concentrations in dry seeds stored under ambient indoor conditions (22 ± 2°C; 37 ± 7% RH) since purchase. The left Y-axis shows concentrations of α-tocopherol (filled red circle), α-tocotrienol (open red circle), β-tocopherol (upward green triangle), γ-tocopherol (filled blue square), γ-tocotrienol (open blue square), δ-tocopherol (downward filled black triangle) and δ-tocotrienol (downward open black triangle) in (A) Daucus carota, (B) Lepidium sativum, (C) Raphanus sativus var. sativus, (D) Helianthus cucumerifolius, (E) Lactuca sativa, (F) Phaseolus vulgaris, (G) Cucumis sativus and (H) Raphanus sativus var. niger, n = 4 replicates ± SD. Total germination is indicated by the dotted line (right Y-axis), as shown in Fig. 1.
Discussion
The aim of this study was to investigate relationships between TG and antioxidants in seeds stored under ambient conditions from species with contrasting seed longevity. For all species, except C. sativus, seed lots ranged from maximum to no TG, enabling the accurate calculation of P50. Relative seed longevity between species in this study generally conform to other ageing studies (Priestley et al., Reference Priestley, Cullinan and Wolfe1985; Walters et al., Reference Walters, Wheeler and Grotenhuis2005b; Nagel and Börner, Reference Nagel and Börner2010). One explanation for differing longevity is the composition of seed storage compounds, with lipid-rich seeds tending to possess shortened longevity (Nagel and Börner, Reference Nagel and Börner2010). However, all seeds contain lipids in the form of membranes, and membrane integrity is essential to organelle and cell compartmentalization, and thus viability. Seeds of Arabidopsis and tomato mutants deficient in tocochromanols show reduced longevity and suffer more lipid peroxidation than wild-type seeds (Mène-Saffrané et al., Reference Mène-Saffrané, Jones and DellaPenna2010; Chen et al., Reference Chen, Li, Fang, Shi and Chen2016), supporting a role for tocochromanols in enabling seed longevity. A positive linear correlation was found for all species between TG and amounts of the most abundant tocochromanol, indicating the consumption of tocochromanols occurred during seed storage, although this correlation is weak for L. sativum (R 2 = 0.17) and R. sativus var. sativus (R 2 = 0.30). In these two species, at least, low levels of γ-tocopherol were measured in seeds from 2021 without storage, contributing to this weak correlation. Temperature and soil moisture during seed development, and particularly during seed filling, affect tocochromanol concentrations (Oomah et al., Reference Oomah, Kenaschuk and Mazza1997; Siger et al., Reference Siger, Michalak, Cegielska-Taras, Szala, Lembicz and Nogala-Kalucka2015; Carrera and Seguin, Reference Carrera and Seguin2016; Haro et al., Reference Haro, Dardanelli and Martinez2020). Since seeds used in this study were harvested from different years, seasonal climatic differences during seed production would have influenced seed development and tocochromanol contents before storage. Without storage, it is not possible to say if seeds from 2021 with lowered tocochromanol amounts would have shortened longevity, but there are indications that seeds from other seasons with relatively low tocochromanol levels have shortened longevity, for example 6-year-stored R. sativus var. sativus.
A decrease in tocochromanol concentrations does not always occur during seed ageing, for example when rapid ageing protocols are used that lead to fluid cytoplasm (Seal et al., Reference Seal, Zammit, Scott, Nyamongo, Daws and Kranner2010b; Groot et al., Reference Groot, Surki, de Vos and Kodde2012, Reference Groot, van Litsenburg, Kodde, Hall, de Vos and Mumm2022; Morscher et al., Reference Morscher, Kranner, Arc, Bailly and Roach2015; Lee et al., Reference Lee, Kwak, Yoon, Lee and Hay2017; Roach et al., Reference Roach, Nagel, Börner, Eberle and Kranner2018; Schausberger et al., Reference Schausberger, Roach, Stoggl, Arc, Finch-Savage and Kranner2019). Recently, Gerna et al. (Reference Gerna, Ballesteros, Stöggl, Arc, Seal, Marami-Zonouz, Na, Kranner and Roach2022) showed that lipid peroxidation and the consumption of tocochromanols only occur during seed ageing when the cytoplasm is glassy. Therefore, the ambient ageing conditions in the current study, leading to seeds with MC <0.05 g H2O g−1 DW and the cytoplasm being glassy-in-state (Buitink and Leprince, Reference Buitink and Leprince2008), rendered the seeds vulnerable to lipid peroxidation, which may have contributed to viability loss.
Redox poising by LMW thiol/disulphide couples is at the basis of cellular redox homeostasis (Schafer and Buettner, Reference Schafer and Buettner2001; Foyer and Noctor, Reference Foyer and Noctor2011), especially in seeds (Kranner et al., Reference Kranner, Birtic, Anderson and Pritchard2006; Colville and Kranner, Reference Colville and Kranner2010). Oxidative shifts of the cellular redox environment, as viewed through E GSSG/2GSH, have been closely related to losses of seed viability in seeds that age rapidly when the cytoplasm is fluid (Kranner et al., Reference Kranner, Birtic, Anderson and Pritchard2006; Roach et al., Reference Roach, Beckett, Minibayeva, Colville, Whitaker, Chen, Bailly and Kranner2010; Birtić et al., Reference Birtić, Colville, Pritchard, Pearce and Kranner2011; Morscher et al., Reference Morscher, Kranner, Arc, Bailly and Roach2015; Nagel et al., Reference Nagel, Kranner, Neumann, Rolletschek, Seal, Colville, Fernández-Marín and Börner2015; Schausberger et al., Reference Schausberger, Roach, Stoggl, Arc, Finch-Savage and Kranner2019). In those studies, P50 associates with E GSSG/2GSH values between −180 and −160 mV, while in this study P50 associates with E GSSG/2GSH values between −180 and −200 mV (Fig. 2), which is a value range found in other seeds aged slower with glassy cytoplasm (Seal et al., Reference Seal, Zammit, Scott, Nyamongo, Daws and Kranner2010b; Gerna et al., Reference Gerna, Ballesteros, Stöggl, Arc, Seal, Marami-Zonouz, Na, Kranner and Roach2022). An exception is P. vulgaris, in which E GSSG/2GSH values were much higher due to very low GSH concentrations. Although P. vulgaris seeds are known to possess more hGSH than GSH (Klapheck, Reference Klapheck1988), the main LMW thiol/disulphide couple in this Brittle Wax var. of P. vulgaris was γ-GC. P. vulgaris is known to produce γ-glutamyl dipeptides (Giada et al., Reference Giada, Miranda and Lanfer Marquez1998), but γ-GC was, so far, never found as the dominant LMW thiol/disulphide couple in seeds of 73 investigated species of Leguminosae (Colville et al., Reference Colville, Saez, Lewis and Kranner2015). Although considered more as a GSH precursor than antioxidant, γ-GC has a γ-glutamyl moiety coupled to cysteine, enabling this dipeptide to be a substrate to glutathione peroxidase and glutathione transferase (Quintana-Cabrera et al., Reference Quintana-Cabrera, Fernandez-Fernandez, Bobo-Jimenez, Escobar, Sastre, Almeida and Bolaños2012; Muraoka et al., Reference Muraoka, Yoshida, Ohno, Matsuura, Nagano, Hirata, Arai and Hirata2021). Evidently in all species, E γGCCGγ/2γGC parallels E GSSG/2GSH, indicating that both are equally responding to the given seed redox environment and buffering ROS production. Indeed, the average R 2 values between TG and E GSSG/2GSH or E γGCCGγ/2γGC for all species are 0.59 and 0.58, respectively (Supplementary Table S1). Thus, the main difference leading to the lower redox potential of these two couples is not a different redox activity, but the concentration of thiol form, for which GSH is much higher than γ-GC in all species except P. vulgaris.
In conclusion, viability loss under the ambient conditions of this study, leading to a glassy cytoplasm, associated with oxidative damage to the lipid and aqueous parts of the seed, regardless of species-specific seed longevity. This is in contrast to rapid seed ageing tests often used in research projects, which places the cytoplasm into a fluid state and leaves the lipid phase mostly undamaged during ageing. Furthermore, a lesser thiol/disulphide oxidative shift occurred in seeds aged with glassy cytoplasm, relative to when the cytoplasm is fluid, also highlighting that different ageing mechanisms occur when seeds are aged with glassy or fluid cytoplasm.
Supplementary material
To view supplementary material for this article, please visit: https://doi.org/10.1017/S0960258522000101.
Financial support
This work was supported by the Austrian Science Foundation (FWF P 32599).
Conflicts of interest
The author(s) declare none.