Introduction
Europa represents one of Jupiter's most fascinating satellite bodies due to the increased astrobiological interest that potentially lies beneath the icy surface. Geologists have indicated that there is a high probability of a saltwater ocean beneath the ice shell and potential lakes within the ice shell due to local melting (Soderlund et al. Reference Soderlund, Schmidt, Wicht and Blankenship2014). The dark red surface materials contain salts potentially from the ocean, that can promote chemical leaching through fissure/cracks in the ice shell (Brown and Hand Reference Brown and Hand2013). All of these factors suggest Europa has the chemical energy to potentially sustain life. Although NASA's Europa Clipper is not a life detection mission, it will conduct detailed reconnaissance of Europa to better discern through specific scientific investigations whether the icy moon could harbour conditions suitable for life.
One of the key factors in understanding any potential biology on Europa means that humans must not irrevocably contaminate it before studying it for the existence of possible life, a critical facet of planetary protection (PP). PP is the practice of protecting solar system bodies from forward contamination by Earth life and protecting Earth from potential backward contamination, such as possible harmful extraterrestrial contamination that may be returned from other solar system bodies. The Committee on Space Research (COSPAR), established by the International Council for Science in 1958, further outlines an international PP policy (Rummel Reference Rummel2015). The foundation of the COSPAR policy is established in the 1967 Outer Space Treaty, Article IX, which allows for the study of the celestial bodies such that exploration of those bodies ‘avoids their harmful contamination and also adverse changes in the environment of the Earth resulting from the introduction of extraterrestrial matter and where necessary shall adopt appropriate measures for this purpose’ (United Nations 1967).
COSPAR's PP policy categorizes space missions according to the type of encounter (i.e. flyby, orbiter, lander or sample return) and the target destination, including any bodies encountered en route (National Aeronautics and Space Administration 2011). As the target destination increases in relevance in providing clues to the process of chemical evolution and/or the origin of life and where the scientific community judges there is a risk that biological contamination would compromise future scientific enquiries (National Aeronautics and Space Administration 2011), the stringency of hardware cleanliness requirements for a mission also increases.
During the early years of PP, all forward contamination controls for missions were guided by a probabilistic approach as the framework for developing ‘quarantine standards’, which are now considered PP requirements (Werber Reference Werber1975).
Later, PP requirements were also defined in terms of a quantitative bioburden limit or spore allocation, for a target body similar to Mars. However, for ocean worlds, a quantitative probability of < 1 × 10−4 of contamination of a liquid water body, has been used since 1962 (Jaffe Reference Jaffe1963). Historically, Mars was also bound by a similar probability during the days the Viking landers were in development; however, post-Viking it became clear that Mars was much less hospitable to Earth life than previously hypothesized, so investigators proposed a ‘by exception’ mission categorization framework that eliminated probabilistic requirements for all objects unable to host Earth life in favour of bioburden based requirements (Devincenzi et al. Reference Devincenzi, Stabekis and Barengoltz1983).
The Europa Clipper Mission consists of an orbiter spacecraft on a trajectory that allows for approximately 40 relatively close (25–2700 km) encounters with the icy moon Europa to ensure global coverage of the icy body (Fig. 1). The Europa Clipper mission will explore Europa and investigate its habitability by utilizing a set of five remote-sensing instruments that cover the spectrum from thermal emission through the ultraviolet, four in-situ fields and particles instruments, a dual-frequency radar and a gravity science investigation. Key mission objectives will be to produce high-resolution images of Europa's surface, determine its composition, look for signs of recent or ongoing geyser activity, measure the thickness of the icy shell, search for subsurface lakes and determine the depth and salinity of Europa's ocean. NASA adopted requirements in NASA Procedural Requirements (NPR) 8020.12D consistent with COSPAR policy to enable scientific research.

Fig. 1. Europa Clipper's Science Tour groundtracks. The goal of Europa Clipper's groundtracks is to enable global coverage of regions of Europa from Jupiter orbit, acquire high-quality data despite the intense Europa radiation environment and ensure capability for obtaining synergistic data from all instruments simultaneously and during each flyby, in a simple and repeatable manner.
Specifically, the NPR 8020.12D requirements for flybys, orbiters and landers to icy satellites, including bioburden reduction, shall be applied to reduce the probability of inadvertent contamination of an ocean or other liquid water body to less than 1 × 10−4 per mission. Thus, the Europa Clipper Mission has been designated a PP Category III mission by the NASA Planetary Protection Officer in February of 2018. Contamination, as it relates to icy bodies, is further defined as ‘the introduction of a single viable terrestrial microorganism into a liquid-water environment’. The calculation of this probability included a conservative estimate of parameters, and addressed the following factors, at a minimum (National Aeronautics and Space Administration 2011):
• bioburden at launch
• cruise survival for contaminating organisms
• organism survival in the radiation environment adjacent to the target
• probability of encountering/landing on the target, including spacecraft reliability
• probability of surviving landing/impact on the target
• mechanisms and timescales of transport to the subsurface
• organism survival and proliferation before, during and after subsurface transfer.
On 13–15 November 2018, the Europa Clipper Planetary Protection Workshop was held. The workshop brought together external subject matter expert (SME) scientists and engineers in relevant fields of study, members of NASA Office of Planetary Protection, external stake holders, inter-agency partners, members of academia and members of the Europa Clipper project team. The goals of the workshop were to:
(i) validate the Probabilistic Risk Assessment modelling framework for Europa Clipper PP;
(ii) agree on model input values, or on a plan to derive/identify appropriate model inputs;
(iii) develop workshop concurrence regarding future PP research plans and their priority.
Pioneered by the nuclear industry, Probabilistic Risk Assessments (PRAs) have proved a useful tool for government agencies to assess safety (Stamatelatos et al. Reference Stamatelatos, Dezfuli, Apostolakis, Everline, Guarro, Mathias, Mosleh, Paulos, Riha, Smith, Vesely and Youngblood2011). This analytical method relies heavily on mathematics as a comprehensive, structured and logical analysis method to identify and assess risks in complex technological systems for cost-effectively improving their safety and performance. PRAs have historically been a preliminary design review and critical design review gate products for ‘Class A’ NASA missions, including robotic exploration. They have been applied to NASA's human flight systems, spacecraft reliability models and the approval of all missions carrying Multi-Mission Radioisotope Thermoelectric Generator (MMRTG) (e.g.Mars 2020 Perseverance Rover). The PP PRA will help inform acceptable trades regarding as-launched bioburden and demonstrate the ability to meet the probabilistic requirements for a Category III mission. Herein, we present and review the workshop proceedings as they relate to the workshop goals and the recommendations from the external SMEs and describe the Europa Clipper's Planetary Protection Probabilistic model, and the PP inputs necessary for the biological sub-model.
Europa Clipper mission and science overview
In order to understand the PP impact of this workshop in context with the PRA model, workshop participants received an overview of the Project science objectives, associated scientific hardware and mission plan/design. Europa Clipper project team members outlined the flight system components and scientific instruments, and most importantly, where critical hardware was located on the spacecraft. This overview is significant in the understanding of the many parameters used for the PRA model, especially the spacecraft reliability, how heavily the payload was shielded from radiation and other environmental characteristics (e.g. thermal, ventilation, etc.). Europa Clipper is scheduled to launch no later than 2024, and complete a Jupiter orbit insertion in 2030 after a 6-year Mars Earth Gravity Assist (MEGA) trajectory. The science mission will last 3.5 years with greater than 40 Europa flybys. Finally, the spacecraft is targeted for disposal into Jupiter's moon, Ganymede, based on updated 2021 science and mission design follow on conversations after the mission has concluded.
The Europa Clipper spacecraft will perform long, looping orbits with repeated close (~25 km in many cases) flybys of the icy moon. These close flybys will provide detailed imaging of Europa's surface and subsurface. Europa Clipper's payload will consist of nine scientific instruments including cameras and spectrometers to produce high-resolution images of Europa's surface and determine its composition (Fig. 2). An ice penetrating radar will determine the thickness of the moon's icy shell and search for subsurface lakes. The mission will also carry a magnetometer to measure the strength and direction of the moon's magnetic field and determine the depth and salinity of its ocean. Gravity measurements will also help confirm the existence of Europa's subsurface ocean. A thermal instrument will survey Europa's frozen surface in search of recent eruptions of warmer water at or near the surface, while additional instruments will search for evidence of water and tiny particles in the moon's thin atmosphere.
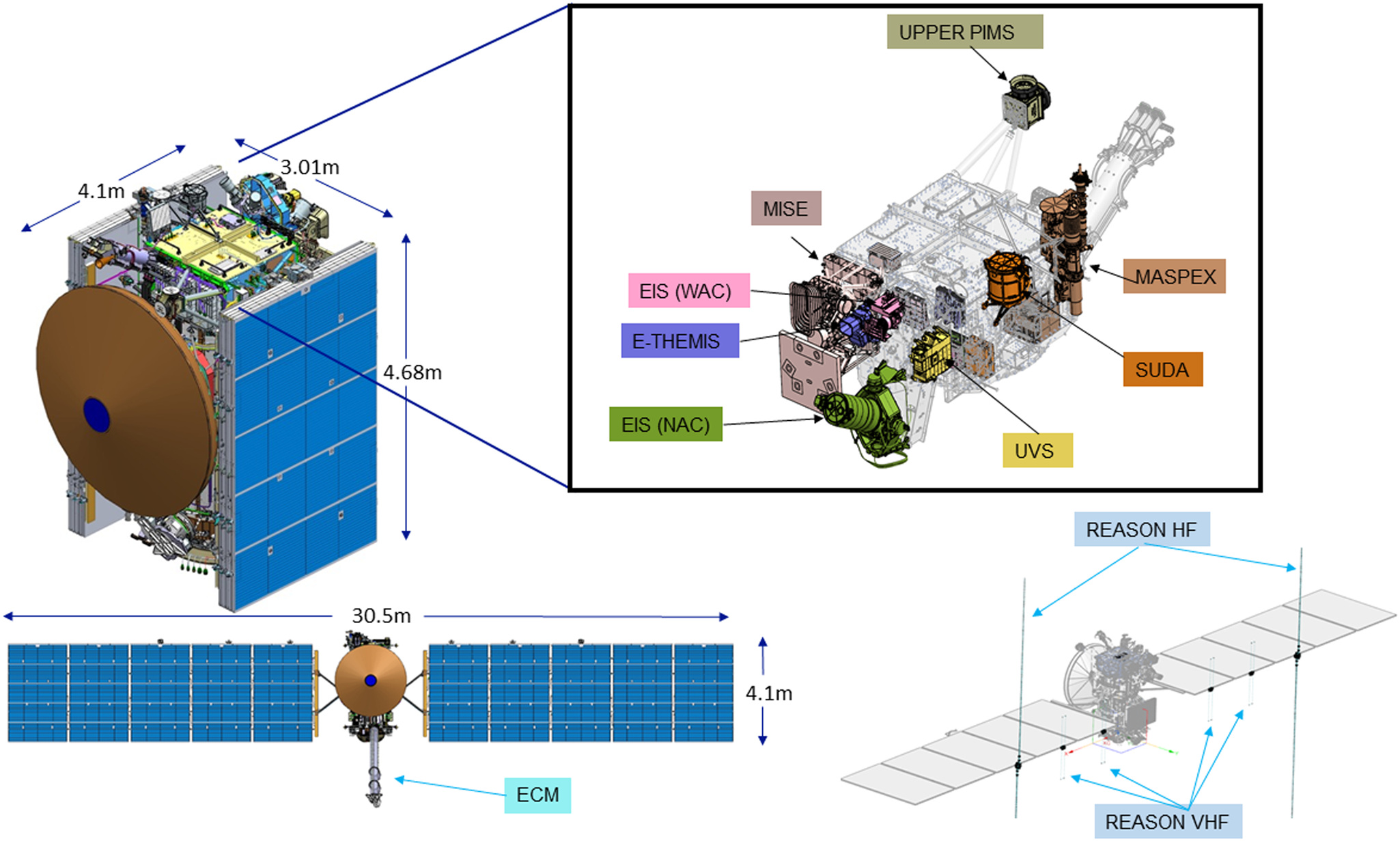
Fig. 2. Europa Clipper's payload. PIMS, plasma instrument for magnetic sounding; ECM, Europa Clipper magnetometer; MISE, mapping imaging spectrometer for Europa; EIS, Europa imaging system; WAC, wide-angle camera; NAC, narrow-angle camera; REASON, radar for Europa assessment and sounding: ocean to near-surface; E-THEMIS, Europa thermal emission imaging system; MASPEX, mass spectrometer for planetary exploration/Europa; UVS, ultraviolet spectrograph/Europa; SUDA, surface dust mass analyser.
For PP purposes, it is critical to understand where hardware is located on the spacecraft, its reliability, how heavily it is shielded from radiation, and its other environmental characteristics (e.g. thermal, ventilation, etc.). Instruments and their components are located across the spacecraft, with electronics and other radiation sensitive optics requiring heavy shielding, while other less sensitive booms and structures are fully exposed to the harsh elements of space as well as the Jovian environment (Fig. 2). Each of these individual features must ultimately be assessed when formulating a probability of contamination. The highly sensitive imaging instruments must be shielded from the Jovian radiation using highly resistant environmental shielding. In addition to the exterior flight system instruments, the main spacecraft electronics and other sensitive hardware are stored in a ‘vault’ that is composed of a thick titanium wall that is designed to protect sensitive hardware.
The Europa Clipper PP probabilistic model
A primary motive for the Europa Clipper PP probabilistic model was to show compliance with NASA's probabilistic PP requirement (McCoy et al., Reference McCoy, Dinicola, Everline, Burgoyne and Reinholtz2020) and use a risk-based approach to ensure that the flight system underwent balanced cleaning and microbial reduction procedures prior to launch to satisfy biological contamination risk requirements. This was of particular importance because some hardware and materials used by Clipper can be detrimentally affected by some microbial reduction techniques, like high heat microbial reduction. Some microbial reduction protocols can also have impact to schedule, cost and performance of the flight project. Alternatively, if hardware is not cleaned sufficiently, future scientific investigations could be impacted, which is the critical aspect of PP. As described in McCoy et al., the PP PRA equation for Europa Clipper is composed of three sub-models, impact, resurfacing and biological. The impact model includes the spacecraft reliability analysis, trajectory analysis and calculation of impact probability. The resurfacing model introduces a model of Europa resurfacing activity for purposes of quantifying PP risk. It considers Europa geophysics and conservatively models any resurfacing event as a contact with the Europa subsurface. Lastly, the biological model assesses microorganism survival relative to the estimated at-launch bioburden, including organism lethality en route to Europa and on its surface, in the event of an off-nominal impact. If hardware was not cleaned sufficiently, there is a risk of contaminating Europa with terrestrial organisms during an unplanned impact. The Planetary Protection Equipment List (PPEL) provides an estimated initial bioburden at launch associated with the Clipper flight system that is inputted into the model to calculate the final probability of contamination.
Additionally, a critical feature affecting the overall probability of contamination (P C) is a defined period of biological exploration, or finite period into the future by which the search for extraterrestrial life would be completed – later simplified to the period of exploration to accommodate robotic missions. Since the first robotic space probes were launched approximately 50 years ago, 100 years would be too short given the multi-decade pace of outer planet missions. Alternatively, there was no solid justification for 10 000 years after considering the pace of technological change and the length of human civilization (National Research Council 2012). Therefore, an order of magnitude period of biological exploration was proposed that is would not.
After combining the three models for each manoeuver and summing over all manoeuvers, the current best estimate (CBE) of the probability of contamination, P C, is calculated. Prior to the workshop, and input from the SMEs, the initial inputs and parameters of the PRA equated to a probability of P C = 1.5 × 10−4, which did not meet the PP requirement of < 1 × 10−4 (Fig. 4). Three key conclusions were drawn for each model discussed during this workshop and its effect on the total P C: (1) impact model – most sensitive to trajectory but not a viable option. Trajectory biasing is not viable leverage for meeting requirements without drastic mission and science changes. (2) Resurfacing model – most sensitive to a defined period of biological exploration; and (3) biological model – needs more than 11-order reduction in microbial bioburden to affect P C. The discussions during this workshop, highlighted below, focused on dissecting these conclusions. The impact, resurfacing and biological model and described in detail in McCoy et al. (Reference McCoy, Dinicola, Everline, Burgoyne and Reinholtz2020). Here we discuss the biological model in more detail.
The external SMEs agreed the Clipper PRA was a valid approach to assess contamination, but with the several unverified assumptions throughout the assessment combined with limited data regarding the geological resurfacing yielded a conservative (higher) probability of contamination. With additional refinement in the factors of the model, which relied on historical and current data points, this PRA model could successfully meet the probability of contamination requirement.
Planetary protection parameter inputs and future research plans
Biological parameter overview
The proper calibration of any PRA model relies on critical parameters or input data that are needed to be refined based on sound modelling assumptions. In a notional PP PRA, several parameters, particularly in a biological model, should be based on NASA guidance, experiential data, industry standards or historical data points from early space flight missions. The meeting discussion focused on biological inputs for microbial reduction using radiation, spore values and spore to viable organism calculations. The mathematical framework to support PP inputs to the assessment includes the initial bioburden for the spacecraft. The NASA Standard Assay (NSA), a culture-based assay specifically selecting for spore-forming microorganisms approved in the 1970s, remains the approved verification test for assessing bioburden counts. While no additional method is approved for this estimation, there have been significant advances in quantitative polymerase chain reaction and metabolic assays that have been recently used to supplement cultivation assays and determine a spore to culturable and culturable to viable factors. For Clipper, bioburden counts were estimated for each region of the spacecraft and tracked in the Clipper PPEL, a tool that the JPL PP team routinely uses for bioburden tracking, specifically for the Europa Clipper mission. The Europa Clipper PPEL, based on the Clipper Mass Equipment List (MEL), provides greater resolution of mated surfaces and encapsulated volumes (non-metallic and vented volumes) than typical Mars missions and differs from typical Mars missions by not having a bioburden allocation requirement. Initial bioburden estimates are calculated using worst case specification values (National Aeronautics and Space Administration 2011) and the dimensions of the hardware/components to determine total bioburden.
Because the probability of contamination requirement for Category III icy satellite requirement is not defined in spore bioburden allocations but a probability, many of the biological inputs are based on spore to viable organism conversions. Moreover, the NPR probability of contamination is bound by a ‘single organism’; hence, the acceptable allocation of organisms on the Europan surface is essentially zero. Based on our current understanding of biology, it cannot be confidently stated that zero viable organisms exist on the spacecraft. The NASA Planetary Protection Office (PPO) encouraged the SMEs to focus on the intent of the requirement, which is a low probability, not zero probability.
Microbial reduction and ionizing sterilization dose
The shielded areas of the Europa Clipper spacecraft pose a significant PP challenge. The environmental shielding of the instruments and spacecraft vault limit the radiation to the interior hardware surfaces thus reducing the microbial reduction potential from radiation in-flight. Given the environmental protection of the ‘vault’ in particular (e.g. thermal control and lower radiation) there is an increased potential for microbes to refuge within this hardware location thus posing a PP concern. To meet PP requirements, Europa Clipper spacecraft must undergo microbial reduction using various modalities that may include precision cleaning, heat microbial and irradiation to reduce the microbial bioburden to sufficient levels. Gamma radiation and electron beams have been considered as methods of sterilization for pre-flight, as they are used extensively in the medical and food industry to increase the shelf life of many food products (Diehl Reference Diehl2002). For example, the International Atomic Energy Agency (IAEA) recommends 0.001 Megarad (Mrad) – 0.1 Mrad of irradiation for sprouting inhibition of potatoes, onions and garlic; whereas, the World Health Organization (WHO) recommends 1–5 Mrad for commercial sterilization and the elimination viruses.
To understand the sterilization effect of radiation on spacecraft isolated microbes, JPL PP engineers reviewed 60 publications, 60 years of research, 130 radiation survival curves across 70 species in laboratory conditions (Hendrickson Park, 2019, unpublished). Using an agglomerative clustering algorithm to group survival curves, curves were grouped based on the Euclidian distance between their average slopes. The algorithm identified four vegetative clusters, a separate cluster for all spore-formers and identified similarities across the large dataset. Datasets were categorized by (1) dose rate (Mrad min−1), (2) growth phase, (3) irradiation medium and (4) nutrient-rich or poor. Analysing the survival curves revealed that most vegetative cells died between 1 and 3 Mrad; however, extremely tolerant organisms, isolated from spacecraft, such as Deinococcus radiodurans or spore formers like Bacillus megaterium could not be accurately extrapolated. PP plans for European Space Agency's (ESA) 2022 mission JUICE (JUpiter ICy moons Explorer) indicate that the hardware will be considered sterilized at 2.5 Mrad. This value is commonly associated with gamma irradiation in the biomedical industry, and it was suggested by SMEs to use this parameter for the Europa Clipper PRA. It was noted, that for future missions further scientific vetting should be conducted on this parameter once additional data became available.
Bioburden limits for Europa Clipper
NPR 8020.12D guidance does not offer a standardized process for determining the acceptable bioburden for an Icy Moon mission. As a slightly different approach than Martian missions, the Europa Clipper project in coordination with the NASA PPO settled on a spore bioburden density instead of a spore allocation requirement. For Clipper, the hardware would be categorized into two groups, PP-limited and non-PP-limited based on several factors. PP-limited surfaces are defined as hardware meeting one or more of the following conditions: (i) surface is part of an element that cannot meet reliability requirements after 3-log heat microbial reduction and cannot be cleaned, (ii) the surface reaches a radiation dose of at least 2.5 Mrad prior to first possible Europa impact and (iii) surface is sterilized by impact heating. Spores are defined as organisms cultivated by the NASA Standard Assay when used with a heat shock step (NASA-HDBK 6022). Any hardware that is classified as PP-limited would have a bioburden limit of < 1000 spores m−2 while all other hardware that is classified as non-PP-limited will have a bioburden limit < 300 spores m−2. These values were determined using heritage-based bioburden limits seen on Mars spacecraft. The 1000 spores m−2 limit on PP-limited also matches with heritage launch vehicle cleanliness requirements. With the adoption of these bioburden based requirements, this reduced the need for many pieces of Clipper to undergo heat-microbial reduction (HMR) or irradiation reduction. Instead, favouring a more risk-balanced approach to PP implementation such as assembly in clean environments, recontamination prevention, precision cleaning and NSA verification sampling.
PPEL development and conversion factors
The PPEL was used to help provide an initial bioburden estimate for the PRA model. Planetary protection engineers on previous mission used scientific literature, historical engineering judgement, NPR 8020.12D and coordination with the NASA PPO to determine this initial bioburden estimates for hardware. The PPEL, which provides detailed bioburden counts for Europa Clipper spacecraft hardware, was driven by the following conversion factors (1) spores to culturable organisms factor, (2) culturable organisms to viable organisms factor, (3) recontamination from environment and (4) specification values from NPR 8020.12D. Based on historical Viking lander capsule and orbiter data, a correction factor of 60 : 1 (Puleo et al. Reference Puleo, Fields, Bergstrom, Oxborrow, Stabekis and Koukol1977) was applied to every spore count to obtain the total culturable (organisms that are able to be cultivated on a given growth medium) organisms. Then based on the SSB recommendations (Space Studies Board, National Research Council 2000), an additional factor of 1000 was applied to obtain the number of viable organisms (VO), for a total of 60 000 viable organisms for every spore detected on the spacecraft. However, based on current JPL data points (Hendrickson et al. Reference Hendrickson, Lundgren, Mohan, Urbaniak, Benardini and Venkateswaran2017), this parameter may be overly conservative and should be closer to 1200. Based on requirement language, at delivery to System Integration and Test (SI&T), the number of viable microorganisms in each Flight System region shall be less than the allocation thresholds specified in the PPEL. Using these PPEL estimates, the total viable organism allocations are further determined based on hardware surface area and associated lethality factors. The initial model further assumes that each region PPEL allocation receives a 3-log order microbial reduction, following standard PP protocols (e.g. cleaning, recontamination prevention, cleanroom assembly and test, monitoring). Note that as mentioned above some components may not be able to undergo such extensive microbial reduction procedures but the cumulative hardware microbial reduction credit for a given grouping should be 3 orders. Recontamination of spacecraft remains a major concern for hardware and PP engineers. Based on heritage values, an upper bound of 1000 spores m−2 for Clipper with the assumption that the launch vehicle fairing will be clean enough to meet this requirement, and the spacecraft exposed surfaces will be mitigated by Jovian radiation exposure.
The historical value for surface microbial density for microorganisms on free surfaces is outlined in the ‘surface microbial density (d s(0)) parameters, based on environmental cleanliness of the cleanroom (i.e. ISO 8) (National Aeronautics and Space Administration 2011). However, when JPL PP engineers re-examined 861 Interior Exploration using Seismic Investigations, Geodesy and Heat Transport (InSight) mission closeout samples from ISO 8 cleanrooms or better, using the NSA, the results showed a mean spore count of 0.1444 (standard deviation = 0.816). Comparing these numbers to actual historical data from InSight, Mars Science Laboratory (MSL) and Mars 2020, they found that the final bioburden for these missions was only 30% of the starting allocation of 5 × 105 spores. Overall, the spacecraft surfaces for those missions were far cleaner than NPR specification values by 2-orders of magnitude and Clipper's recontamination values should be re-assessed given these historical observations. As an input to the biological model, the PP engineers suggest using a new lower estimate (with margin), rather than an absolute number. The external SMEs agreed that the NPR specification values need updating, but it would be better to understand the genesis of historical spacecraft datasets in combination with compiling mission heritage numbers. New values need to be based on a data-driven peer-reviewed approach.
Sampling devices and recovery
Sampling is an essential component to determining the microbial bioburden present on spacecraft and assembly cleanrooms (Duc et al. Reference Duc, Kern and Venkateswaran2004; Vaishampayan et al. Reference Vaishampayan, Probst, Duc, Bargoma, Benardini, Andersen and Venkateswaran2013). Currently, PP engineers sample an average of approximately 10% of representative spacecraft surfaces as an imposed project specific requirement or heritage-based best practice. The sampling efficiencies of various materials (polyester, nylon-flocked or cotton) range from 11 to 49%, depending on the standard procedure used. These efficiencies, or average recoveries, have had a drastic effect on assessing bioburden allocations as well as the correction factors that are applied to the data. New sampling devices are needed to maximize the limited surface area sampled (< 25 cm2 for swabs and < 1.0 m2 for wipes) and improve overall efficiency and recovery of microbial cells or potentially DNA isolation. Investment in this area could improve cell and DNA extraction, which is the trajectory of future project requirements. By performing additional efficiency studies or exploring new sampling devices, it could provide PP engineers with a higher statistical confidence in the data from the NSA assay, or may increase the number of samples collected within a given area on the spacecraft and reduce the risk of missing critical data points. JPL PP engineers have worked with the NASA PPO to determine recovery efficiencies of Polyester Texwipes TX3211 and TX3224, two materials in use. The SMEs suggest using alternatives to the NSA by leveraging commercial-off-the-shelf products or an Internal Research & Development Program (IRAD) process to identity novel sampling technologies.
NASA standards for planetary protection
Along with sampling devices, better statistical approaches are needed to estimate bioburden numbers from the NSA and generate higher confidence. For example, when ‘zero’ is recorded, indicating no colony forming units (CFU) during the NSA, does this truly represent the absence of spores on the spacecraft given the low sampling efficiency and small area sampled (< 25 cm2). From previous missions, the respective projects, in some cases, conservatively applied a historical value of ‘one’ CFU count for each zero CFU detected. Additional experiments were conducted using Mars 2020 swabs where the JPL PP team demonstrated that out of 373 swab samples, 99.46% were true negatives (zero) by additional culture methods. Because of the stringent less than ‘one viable microorganism’ NPR requirement for the Clipper mission, a ‘zero to one’ for CFU counts for a given sample conversion would not be feasible. This ‘zero to one’ conversion is tied to surface area of spacecraft, and since the Europa Clipper spacecraft is so large, approximately 7500 m2, it takes a large hit from a zero to one conversion value. It was noted that NASA – in conjunction with Idaho National Lab (INL) – is evaluating the statistical approaches for bioburden accounting to provide a viable approach for all mission applications including Clipper.
When developing the PPEL, an upper bound bioburden estimate for worst-case specifications are applied based on historical NPR requirements, which impacts all bioburden estimates, including encapsulated bioburden allocations for spacecraft with large surface areas. Leveraging these parameters to develop a worst-case scenario does not account for improved environmental cleanliness of current ISO cleanrooms when setting these high spore allocations for determining bioburden densities. JPL PP engineers propose creating a gradient specification for use and updating historical data points, to update the specification to become a better representation of most probable values for cleanrooms in which historical data exist. The SMEs commented that manufacturing processes are also much cleaner and more standardized than they were when the specification was created in 1971, so it was reasonable not to use the NPR specifications and to use heritage spacecraft data instead. The NASA PPO confirmed that research is currently being funded through competed Planetary Protection Research (PPR) Opportunities in Space and Earth Science (ROSES) calls to learn more about encapsulated bioburden, a problem that affects all missions. Furthermore, JPL is working with the NASA PPO and INL to establish statistical approaches that utilize distribution bounds instead of strictly using ultra conservative upper bounds.
Microbial reduction and additive effect testing
Applying microbial reduction credit to vegetative organisms was also identified as a potential gap, as there is no specific guidance on how to apply this credit for Europa Clipper or other missions. When using heat microbial reduction, a biological indicator is used to determine whether necessary conditions were met to kill a specified number of microorganisms for a given sterilization process. Biomedical industries use Bacillus atrophaeus (ATCC 9372), which was the previous NASA standard. However, NASA has adopted the use of Bacillus sp. (ATCC 29669) where D-values are between 20 and 50 times longer than those of the previous standard. The new biological indicator has a higher D-value, which leads to longer cycle times. This effect allows less hardy vegetative organisms to be exposed to a longer duration of heat providing a longer chance to be killed. For example, since all burden calculations are treated this way, a 3-log reduction credit is applied to the bioburden calculation for the entire spacecraft, even though most microorganisms are not as hardy as Bacillus sp. (ATCC 29669). In addition, not all heat tolerant organisms are spore-forming, thus, more data are needed to determine the amount of vegetative reduction credit to apply. The external SMEs recommended leveraging the European Space Agency (ESA) data points and other partners to bolster support for using another biological indicator. Until that time, a conservative approach is needed, and NASA will continue to use ATC29669.
Dry heat microbial reduction for NASA hardware typically occurs at temperatures between 104 and 125°C (National Aeronautics and Space Administration, 2005). Limited data have been generated at temperatures greater than 200°C, so questions remain on what log reduction should be applied in those cases. Currently for Europa Clipper, PP engineers are limited to a 4-log credit reduction above 200°C, as a part of conservatism from Mars Atmosphere and Volatile Evolution (MAVEN) on surfaces and Mars Cube One (MarCO) on encapsulated and mated specifications. Recent JPL data showed that the hardy spores Bacillus sp. (ATCC 29669) was killed at temperatures much lower than 500°C for 0.5 s (between 200 and 225°C) (Schubert and Beaudet, Reference Schubert and Beaudet2011). Reviewers agreed that additional studies are needed in this area, particularly regarding high impact heating. Reproducing historical data may provide valuable inputs, but the workshop participants agreed that we need more recently published scientific data to help determine values, where data extrapolation is not possible.
In addition to HMR credit in pre-flight, many of the bio-regions identified on Clipper will experience varying degrees of radiation, desiccation, space vacuum and extreme cold temperatures in-flight. While the biological model investigates the lethality of these in-flight factors individually, many of these surfaces will be exposed to the conditions simultaneously or in a sequence that may offer additive effects for microbial reduction. Currently, there is minimal data about these additive effects on microbial populations and the overall reduction credit that occurs for a microbial population. Several of the external SMEs acknowledged the gap in synergistic research for microbial populations and believed that JPL's experimental approach, exposing cleanroom relevant organisms to flight conditions and analysing using metagenomics, was an appropriate first step to close this gap.
Metagenomics and biological archiving
The NASA Standard Spore Assay provides limited visibility to understanding the total microbial population that may exist on spacecraft or found in cleanrooms (Moissl et al. Reference Moissl, Osman, Duc, Dekas, Brodie, DeSantis and Venkateswaran2007, Vaishampayan et al. Reference Vaishampayan, Probst, Duc, Bargoma, Benardini, Andersen and Venkateswaran2013). During the workshop it was noted that DNA analysis and metagenomics sequencing has been used to analyse low biomass environmental samples from Mars 2020, JPL spacecraft assembly facility cleanroom and on the International Space Station (ISS). Although it was not a feasible method for Europa Clipper, metagenomics offered an attractive supplement for PP implementation as it was not biased by cultivation methods, and it allowed a larger population fraction to be considered for risk-based analysis. Risk, in these cases, could be evaluated in terms of functional genes and/or taxonomic similarity to known problem organisms and potentially identify how to mitigate the risk of contamination. It could allow real-time analysis, yielding risk assessments in less than 48 h, given optimized sample extraction/preparation and data analysis. Moreover, these databases could provide clues to mission science results and questions which can be applied in a broader sense, in context of flight system contamination. To capitalize on the full benefits from metagenomic technologies, several early investments need to be made in low biomass sampling to decrease the possibility of potential contamination, DNA extraction to increase overall sample size and optimization of throughput rates, and biological archiving.
JPL currently maintains an archive collection consisting of approximately 4000 spacecraft-associated microorganisms isolated from the spacecraft and cleanrooms from 7 Mars missions dating back to 1974. These archives prove important for PP because the isolates provide additional data points to support updates to the PP implementation process and allow for greater genomic analysis and characterization as platforms have emerged and improve over the decades. At the time of the workshop Clipper was not archiving microorganisms; however, adopting this practice, like what the Mars Program has done historically, could expand the current archive and support future risk assessment and mitigation efforts using metagenomics or future technologies.
Workshop SME participants agreed on the important role metagenomics data can play in characterizing microbial functionality and recommended a standard protocol be developed to understand sampling efficiencies and data computational and visualization tools. Furthermore, the SMEs commented that archiving Clipper microorganisms was an essential component to leveraging these genetic-based tools, so it is recommended NASA invest in archiving resources. After the workshop was completed the Europa Clipper project started archiving isolates in a minimalistic manner.
SME conclusions on PP inputs
During the workshop, the NASA PPO and external SMEs suggest the additional recommended parameters for radiation and PPEL conversions were reasonable but stressed that the Europa Clipper Project should use NPR 8020.12D specification values for recontamination for inputs to the PPEL until researchers can generate new values through a data-driven, peer-reviewed process to balance risk. In addition to using the quantitative PRA, other qualitative techniques can be applied to continuously improve with mission best practices and leverage lessons learned despite the uniqueness of each mission.
This workshop discussed the current state of the Europa Clipper Planetary Protection Probabilistic Risk Assessment and evaluated its validity for PP requirements regarding icy moon flight missions. Discussions resulted in important and useful understanding of the importance of PP to the PRA process, validated current input parameters and identified future research investments in PP that would help better quantify parameters. Overall, the Europa Clipper Probabilistic Model was accepted and proven mathematically sound. The panel recommended that the conservatism assumed should be relaxed in several key areas. Based on these recommendations from the workshop, several PP input parameters were identified to change in the PRA (Table 1).
Table 1. Workshop decisions and agreements

aUpdated values decided during the workshop.
While reviewing the PRA inputs during this workshop, it became clear that there were several PP knowledge gaps that needed to be addressed by technology and future research investments. The last day of the workshop focused on understanding those gaps and discussing a research path forward. NASA released a 2017 report, ‘Planetary Protection Technologies for Planetary Science Instruments, Spacecraft, and Missions (Pugel and Rummel Reference Pugel and Rummel2018)’ that outlined technical and engineering challenges facing PP and identified areas of development that could be funded by NASA to provide future capabilities. Similarly, the JPL PP group drafted a technology map that highlighted near and long-term investments that could benefit Europa Clipper, offer additional parameters to support the biological model and improve the PP discipline. These investments included additional molecular biology techniques and equipment for experimental testing of microorganisms, research and development of new materials compatible with PP bioburden reduction modalities and data on microbial reduction methods in a space flight environment with multiple modalities occurring concurrently.
Additionally, on day 3 of the workshop, per direction of the PPO and SME recommendations, the project team updated three parameters (1) impact debris to surface grid effect, (2) obliquity threshold for ricochet and (3) radiation sterility (Table I). If more updated parameters are applied to the PRA model in context with the SME recommendations and the outlined workshop discussions, the PRA yields a P C less than the PP requirement of 1 × 10−4 probability of contaminating the Europan ocean. The updates to these three parameters were enough to close the model, and any additional updates (as seen in Table I) would further decrease the probability. Following the workshop, the Europa Clipper project signed a memorandum of understanding (MOU) between JPL and NASA HQ on the overall workshop decisions and agreements outlined above. This MOU set forth a framework of cooperation Europa Clipper Probabilistic Model was accepted, with CBE results demonstrating compliance with the Level 1 Planetary Protection Requirement.
Acknowledgements
The Workshop organizers acknowledge the support of Dr Lisa Pratt (NASA Planetary Protection Officer at the time of the workshop), and Barry Goldstein (JPL, Europa Clipper Program Manager at the time of the workshop), for their support to the Europa Clipper Project and organizing the workshop. Collectively, the invited experts contributed in many ways to the deliberations and recommendations at the workshop. The workshop was carried out at the Jet Propulsion Laboratory, California Institute of Technology, under a contract with the National Aeronautics and Space Administration (80NM0018D0004). The Europa Clipper planetary protection workshop invited SMEs included:
• Corien Bakermans, Ph.D. (Microbiology – Permafrost – Penn State, Altoona)
• Andrei Gribok, Ph.D. (Probability Theory, Statistics and Applied Mathematics – Idaho National Laboratory)
• Aaron Regberg, Ph.D. (Microbiology–Fungi – NASA–Johnson Space Center)
• Brent Christner, Ph.D. (Microbiology – Deep Ice – University of Florida)
• Mark Saunders (Spacecraft Systems Engineering; Retired – Langley Research Center)
• Jeffrey S. Parker, Ph.D. (Physics- Orbital Mechanics/Dynamics – Advanced Space)
• Martin Heinstein (Physics – High Velocity Impact Heat – Sandia National Laboratory)
• Geoff Collins, Ph.D. (Europa Science/Geology – Wheaton College)
• Simon Kattenhorn, Ph.D. (Europa Science/Geology/Tectonics – University of Alaska, Anchorage)
• Alyssa Rhoden, Ph.D. (Europa Science/Geology – Southwest Research Institute, Boulder)
• Robert Youngblood, Ph.D. (Risk Analysis/Reliability Engineering – Idaho National Laboratory)
• Andrew Schuerger, Ph.D. (Microbiology – Community – University of Florida)
• Bill Taber, Ph.D. (Applied Mathematics/Probability – NASA–Jet Propulsion Laboratory)
• David Littlefield, Ph.D. (Cratering Physics – University of Alabama, Birmingham)