Introduction
The obesity epidemic is recognised as one of the major public health problems facing the world( 1 ). The number of obese people in the world has more than doubled since 1980. By 2014, more than 1·9 billion adults were overweight, and among these more than 600 million were obese. In percentages, 39 % of adults were overweight in 2014 and 13 % were obese( 2 ).
The majority of body alterations related to obesity occur initially in peripheral tissues( Reference Galic, Oakhill and Steinberg 3 – Reference Knight 5 ), such as adipose tissue, liver and muscles. Nevertheless, some studies have already revealed that obesity can also cause central nervous system (CNS) impairments( Reference Convit 6 , Reference Shefer, Marcus and Stern 7 ).
The alterations related to obesity include low-grade chronic inflammation. As obesity has been considered as an inflammatory disease, it has aroused interest in the therapeutic manipulation of inflammation( Reference Coelho, Oliveira and Fernandes 8 – Reference Johnson, Milner and Makowski 10 ). In this context, certain substances may play an important role in mediating inflammation and related alterations( Reference Muñoz and Costa 11 ). Some studies have shown that n-3 PUFA, which are essential fatty acids with several important biological effects( Reference Wall, Ross and Fitzgerald 12 – Reference Gomez-Pinilla 14 ), could contribute to treating obesity and related metabolic alterations( Reference Buckley and Howe 15 – Reference Campbell and Bello 17 ).
Thereby, considering that n-3 PUFA could improve metabolic alterations related to obesity, the aim of this narrative review was to summarise the literature concerning the application of n-3 PUFA as an obesity treatment. First, we provide a brief description of the obesity pathophysiology, including alterations that occur in peripheral tissues and in the CNS. Next, we define n-3 PUFA, describe its sources and the general effects. In the sequence, we explore the main topic linking obesity and n-3 PUFA.
Methods
The literature research was conducted in PubMed and SciELO electronic databases, by the selection of articles related to the topic published between 2007 and 2017. A combination of the following keywords was used: obesity, inflammation, brain, CNS, n-3 and fish oil. The following combination of terms was searched: obesity AND inflammation, obesity AND inflammation AND brain, obesity AND brain, obesity AND central nervous system, omega-3, obesity AND omega-3, obesity AND fish oil, obesity AND eicosapentaenoic acid, obesity AND docosahexaenoic acid.
Studies using animal models, clinical trials and review articles were included. Clinical trials that tested the use of n-3 PUFA with lifestyle modification were included. Review articles were included both to identify other papers and to provide a view of what has already been revised about the topic. Regarding animal studies, the focus was on the effects of n-3 PUFA on diet-induced obesity, more similar to obesity in humans. Therefore, studies using obesity animal models by genetic modification or applying other interventions to lead to obesity (e.g. castration in rabbits) were excluded. Studies involving any surgical intervention (e.g. bariatric surgery) were also excluded.
The search was carried out by two individual researchers. After screening by keywords, the titles and abstracts of articles were examined to determine whether they contained relevant data for this review. The two researchers carried out the reading of the titles and abstracts and selected the articles following the inclusion and exclusion criteria established. After that, both researchers had a consensus meeting to align any disparity in the inclusion or exclusion of articles. Subsequently, the complete reading of the articles was carried out. The original articles that addressed the use of n-3 PUFA to treat obesity and related alterations are included in Tables 1 and 2.
Table 1 Review of animal studies on n-3 PUFA for obesity (and related alterations)

↓, Decrease. ↑, increase; AO, argan oil; cHF, maize-oil-based high-fat diet; FO, fish oil; HF, high fat; HFD, high-fat diet; HFHF, high-fat/high-fructose; HFHS, high-fat/high-sucrose; Icv, intracerebroventricular; MO, microalgal oil; SC, standard chow; WAT, white adipose tissue; HFru, high-fructose diet.
* The column ‘Duration’ refers to the time that the n-3 PUFA was tested, not the complete experiment.
Table 2 Review of human studies on n-3 PUFA for obesity (and related alterations) (Mean values and standard deviations and 95 % confidence intervals)
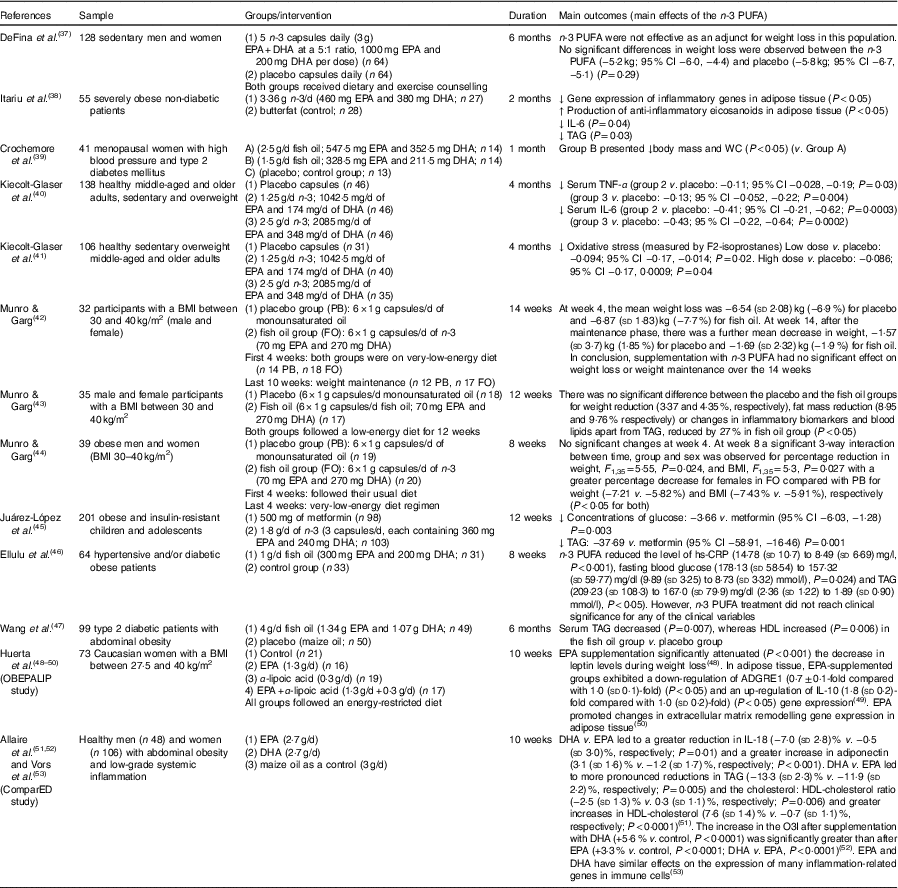
↓, Decrease. ↑, Increase; ADGRE1, adhesion G protein-coupled receptor E1; CRP, C-reactive protein; hs-CRP, high-sensitivity C-reactive protein; O3I, Omega-3 Index; WC, waist circumference.
Obesity
The World Health Organization( 2 ) defines obesity as an abnormal or excessive fat accumulation that can harm an individual’s health. One of the major problems of obesity is being both a disease and a risk factor for several other disorders, such as cardiovascular diseases, type 2 diabetes mellitus, respiratory diseases, musculoskeletal disorders and some cancers, which have a great impact on quality of life and longevity of people( 2 , Reference Knight 5 , Reference Bhaskaran, Douglas and Forbes 54 ).
Changes in dietary patterns and physical activity in recent decades, such as excessive consumption of food or high-energy foods, and sedentary lifestyle are major factors that contribute to the genesis of obesity( 2 ). If the total daily energy intake surpasses the amount of energy spent, the excess is stored as TAG in cells called adipocytes, which form the white adipose tissue( Reference Coelho, Oliveira and Fernandes 8 ). Adipose tissue responds rapidly to excess nutrient consumption by adipocyte hypertrophy and hyperplasia( Reference Halberg, Wernstedt-Asterholm and Scherer 55 ). Therefore, obesity is characterised by increased storage of fatty acids in an expanded mass of adipose tissue( Reference Galic, Oakhill and Steinberg 3 ).
White adipose tissue, along with its important role in energy storage, is an important endocrine organ( Reference Galic, Oakhill and Steinberg 3 , Reference Coelho, Oliveira and Fernandes 8 ). This tissue produces many bioactive molecules, such as cytokines (when secreted by adipose tissue, the cytokines can be called adipokines or adipocytokines), which not only serve as regulators of systemic metabolism but also have immunoregulatory properties( Reference Galic, Oakhill and Steinberg 3 , Reference Ouchi, Parker and Lugus 56 , Reference Exley, Hand and O’Shea 57 ).
Obesity leads to changes in adipokine secretion. Progressive increase of the adipocytes and consequent expansion of the adipose tissue leads to reduced blood supply, with consequent hypoxia, which is related to necrosis and infiltration of macrophages into adipose tissue. Infiltrated macrophages form crown-like structures surrounding adipocytes, leading to adipokine overproduction, which includes pro-inflammatory mediators such as TNF-α, IL-6 and IL-1β ( Reference Galic, Oakhill and Steinberg 3 , Reference Johnson, Milner and Makowski 10 , Reference Maury and Brichard 58 , Reference Mathis 59 ).
Obesity is characterised by a low-grade chronic inflammation, once the elevation of inflammatory markers and cytokines, as well as the presence of macrophages infiltrated into the white adipose tissue, can be detected( Reference Johnson, Milner and Makowski 10 ). This low-grade chronic inflammation in adipose tissue spreads to systemic inflammation and contributes to the onset and progression of associated metabolic disorders, such as insulin resistance, type 2 diabetes mellitus, hyperlipidaemia, atherosclerosis and metabolic syndrome( Reference Ouchi, Parker and Lugus 56 , Reference Donath and Shoelson 60 , Reference Moore and Tabas 61 ). Although most of the changes were initially identified and reported in peripheral tissues, studies conducted in the past decade have shown that obesity is also related to brain changes( Reference Convit 6 , Reference Shefer, Marcus and Stern 7 , Reference Cai 62 ).
Obesity and the brain
Studies have shown that both excessive consumption of saturated fats and obesity can lead to brain damage( Reference Convit 6 , Reference Shefer, Marcus and Stern 7 , Reference Thaler, Yi and Schur 63 ). More specifically, although little is known about the effects of obesity on the brain( Reference Freeman, Zhang and Nair 64 ), studies have found associations between obesity and abnormalities in the hypothalamus( Reference Thaler, Yi and Schur 63 , Reference Milanski, Degasperi and Coope 65 , Reference Moraes, Coope and Morari 66 ), hippocampus, prefrontal cortex and striatum( Reference Boitard, Cavaroc and Sauvant 67 , Reference Nummenmaa, Hirvonen and Hannukainen 68 ).
The hypothalamus is a brain structure that plays a central role in the regulation of energy homoeostasis, integrating multiple metabolic signals from peripheral organs and modulating eating behaviour and energy metabolism( Reference Velloso and Schwartz 69 – Reference Drougard, Fournel and Valet 72 ). Therefore, changes at this site may cause neural control loss and make room for obesity onset and worsening( Reference Williams 70 ).
The hypothalamus rapidly responds to metabolic challenges (e.g. hyperenergetic diet)( Reference Kälin, Heppner and Bechmann 73 ). Overnutrition causes hypothalamic inflammation, which disrupts the normal homoeostasis of energy intake and expenditure, as well as alters insulin secretion and sensitivity( Reference Shefer, Marcus and Stern 7 , Reference Thaler, Yi and Schur 63 ). Unlike inflammation in peripheral tissues – a process that develops over weeks of high-fat-diet feeding in rodent models – markers of hypothalamic inflammation are elevated within 1 to 3 d of high-fat-diet exposure, before weight gain( Reference Thaler, Yi and Schur 63 , Reference Kälin, Heppner and Bechmann 73 ). Therefore, the excess nutrients, as well as circulating inflammatory cytokines seen in obesity, such as TNF-α, IL-1β and IL-6, activate intracellular inflammatory pathways in a variety of target cells( Reference Thaler, Choi and Schwartz 74 – Reference Gregor and Hotamisligil 76 ).
By using MRI in humans, Thaler et al.( Reference Thaler, Yi and Schur 63 ) have found evidence of increased gliosis in the mediobasal hypothalamus of obese individuals, suggesting that, similarly to what occurs in rodents with obesity induced by a high-fat diet, obesity in humans is associated with the neuronal injury in the hypothalamus, which is a crucial brain area for body weight control. Thereby, the hypothalamus functions may be altered when inflamed, leading to an imbalance between food intake and energy expenditure( Reference Cai 62 , Reference Milanski, Degasperi and Coope 65 ).
The hippocampus, the brain structure involved in cognition, memory, learning and emotions, is also vulnerable to the inflammatory process present in obesity( Reference Shefer, Marcus and Stern 7 , Reference Boitard, Cavaroc and Sauvant 67 , Reference Kanoski and Davidson 77 – Reference Miller and Spencer 79 ). Excessive consumption of food rich in saturated fat and sugar, besides being related to obesity, is associated with hippocampal impairment( Reference Davidson, Hargrave and Swithers 78 ). Therefore, cognitive function may also be impaired by high-fat intake and by obesity( Reference Miller and Spencer 79 , Reference Park, Park and Choi 80 ).
In rodent studies, Moroz et al.( Reference Moroz, Tong and Longato 81 ) showed that high-fat-diet feeding increased lipid peroxidation in the hippocampus, as indicated by high 4-hydroxynonenal levels. Park et al.( Reference Park, Park and Choi 80 ) also showed that consumption of a high-fat diet leads to increased lipid peroxidation and impairs neurogenesis in the hippocampus. Jeon et al.( Reference Jeon, Jeong and Shin 82 ) showed that a high-fat diet promotes an increase in hippocampal TNF-α expression and activates microglia. Boitard et al.( Reference Boitard, Cavaroc and Sauvant 67 ) reported that the intake of a high-fat diet increases the expression of pro-inflammatory cytokines in the hippocampus.
Studies have shown that obesity may also be related to changes in the reward system, highlighting abnormalities in the prefrontal cortex and striatum( Reference Nummenmaa, Hirvonen and Hannukainen 68 , Reference Tomasi and Volkow 83 , Reference Farr, Li and Mantzoros 84 ). It has been shown that dopamine levels in the medial prefrontal cortex and in the striatum of rats prone to obesity have been reduced( Reference Geiger, Behr and Frank 85 ). In addition, a study on rodents has shown hypofunction of the dopaminergic system in obese rats( Reference Mathes, Nehrenberg and Gordon 86 ). Dysregulation of the dopaminergic system is associated with changes that include addiction and hyperphagia( Reference Mathes, Nehrenberg and Gordon 86 ). Regarding humans, a link has been found between the elevation of BMI and low dopaminergic activation in the striatum in obese women( Reference Stice, Spoor and Bohon 87 ). Furthermore, MRI of the brain of young women showed striated hypofunction in those who were prone to weight gain( Reference Stice, Yokum and Blum 88 ).
Obesity treatment
Obesity treatment is extremely important. One should not only seek weight loss but the individual’s metabolic health as well. Lifestyle modification (intervention focusing on diet and exercise) constitutes the conventional and first-choice treatment for obesity. The failure of these measures, mainly with regard to the maintenance of the results, has emphasised the need for adjuvant therapeutic resources( Reference Pucci and Finer 89 ).
Obesity treatment remains a major challenge. Food re-education therapy and exercise often do not achieve satisfactory results. Pharmacological therapy has been shown to be fraught with serious adverse effects, and many drugs have been withdrawn from the market because of unfavourable risk–benefit relationships( Reference Pucci and Finer 89 ).
In view of increasing obesity rates in the world, as well as the recurrent treatment difficulties currently observed, several studies are required to broaden treatment options. In this context, studies have shown that n-3 PUFA have the potential to help treat obesity( Reference Golub, Geba and Mousa 16 , Reference Campbell and Bello 17 , Reference Itariu, Zeyda and Hochbrugger 38 , Reference Flachs, Ruhl and Hensler 90 , Reference Hensler, Bardova and Jilkova 91 ).
n-3 PUFA
n-3 Fatty acids (ω-3, n-3 or w-3) are a family of PUFA, which are vital for the functioning of the body, termed essential fatty acids( Reference Surette 92 , Reference Gómez Candela, Bermejo López and Loria Kohen 93 ). The term ‘n’ refers to the position of the first double bond, and n-3 PUFA have the first double bond between the third and fourth carbon atoms( Reference Arbex, Bizarro and Santos 94 ). The major n-3 PUFA are α-linolenic acid (ALA) (eighteen carbons, three double bonds), EPA (twenty carbons, five double bonds) and DHA (twenty-two carbons, six double bonds)( Reference Gómez Candela, Bermejo López and Loria Kohen 93 – Reference Anderson and Ma 95 ).
Lipid components, especially fatty acids, play an important role in the structure of cell membranes and in metabolic processes( Reference Arbex, Bizarro and Santos 94 ). Because n-3 PUFA cannot be synthesised by the body, they need to be obtained from dietary sources( Reference Surette 92 , Reference Arbex, Bizarro and Santos 94 ). These sources may be foods of plant origin, in the form of ALA, or from some species of fish, in the form of EPA and DHA( Reference Ellulu, Khaza’ai and Abed 13 , Reference Surette 92 , Reference Anderson and Ma 95 ).
ALA is found in flaxseed, soyabean, chia, rapeseed and walnuts, and it can be metabolised in EPA and DHA via elongase and desaturase enzymes( Reference Arbex, Bizarro and Santos 94 , Reference Simopoulos 96 ). However, humans are inefficient in performing this synthesis from ALA( Reference Anderson and Ma 95 , Reference Barceló-Coblijn and Murphy 97 , Reference Brenna, Salem and Sinclair 98 ), as these enzymes are influenced by innumerable aspects, such as smoking, alcohol consumption, diabetes, stress and ageing( Reference Anderson and Ma 95 , Reference Simopoulos 96 , Reference Brenna, Salem and Sinclair 98 , Reference Calder 99 ). Sea fish, such as sardines, salmon, tuna, mackerel and herring, are the main sources of EPA and DHA( Reference Arbex, Bizarro and Santos 94 , Reference Calder 99 ).
n-3 PUFA are present in cell membranes, particularly in the lipid bilayer of the plasma membrane, and depending on their proportion in the membranes they may undergo changes in fluidity and, therefore, in their functions( Reference Gómez Candela, Bermejo López and Loria Kohen 93 ). DHA, in particular, is one of the most abundant components in the brain’s structural lipids( Reference Simopoulos 96 , Reference Agrawal and Gomez-Pinilla 100 ) and is a key component of neuronal membranes at signal transduction sites, which indicates that its action is vital for brain function( Reference Gomez-Pinilla 14 , Reference Agrawal and Gomez-Pinilla 100 ). The incorporation of EPA and DHA into the diet can influence not only lipid composition and structure of cell membranes but also the physiological responses that depend on these membranes, as is the case with cell signalling mechanisms( Reference Farooqui 101 ). There is evidence that inadequate intake of maternal n-3 PUFA may lead to abnormal development and function of the CNS( Reference Sun, Simonyi and Fritsche 102 ). DHA-derived lipid mediators are neuroprotective and ameliorate neurological disorders( Reference Sun, Simonyi and Fritsche 102 ). There is also evidence that DHA may ameliorate cognitive decline and affect behavioural symptoms in major neuropsychiatric disorders such as dementia, schizophrenia and depression( Reference Lauritzen, Brambilla and Mazzocchi 103 ).
n-3 PUFA derived from fish oil (EPA and DHA) exerts important effects on the inflammatory pathways, acting as anti-inflammatory agents( Reference Wall, Ross and Fitzgerald 12 , Reference Ellulu, Khaza’ai and Abed 13 , Reference Chapkin, Kim and Lupton 104 , Reference Yates, Calder and Ed Rainger 105 ), because of its ability to interact with the major inflammatory signalling pathways, as well as its suppressive effect on the production of cytokines( Reference Yates, Calder and Ed Rainger 105 , Reference Lorente-Cebrián, Costa and Navas-Carretero 106 ). The anti-inflammatory mechanisms of action exerted by EPA and DHA include suppression of NF-κB, reduction of eicosanoid production, as well as alteration of membrane organisation, particularly those related to the functions of Toll-like receptors and T-lymphocyte signalling( Reference Chapkin, Kim and Lupton 104 , Reference Flock, Rogers and Prabhu 107 ). In addition, the anti-inflammatory properties of EPA and DHA are mediated also through the inhibition of TLR signalling pathways( Reference Flock, Rogers and Prabhu 107 ).
EPA and DHA exert their anti-inflammatory effects by decreasing the production of pro-inflammatory eicosanoids derived from arachidonic acid, as well as by serving as substrates for production of lipid mediators with pro-resolution properties, such as resolvins, protectins and maresins( Reference Flock, Rogers and Prabhu 107 , Reference Martínez-Fernández, Laiglesia and Huerta 108 ). These specialised pro-resolving lipid mediators have potent immunoregulatory actions as they activate specific mechanisms to promote resolution of inflammation( Reference Flock, Rogers and Prabhu 107 , Reference Martínez-Fernández, Laiglesia and Huerta 108 ). Mediators derived from EPA are known as E series resolvins, and those derived from DHA are named as D series resolvins, whereas protectins and maresins are derived only from DHA( Reference Flock, Rogers and Prabhu 107 , Reference Martínez-Fernández, Laiglesia and Huerta 108 ).
Furthermore, other documented n-3 PUFA effects include cardiovascular benefits such as reduction of TAG, cardiac arrhythmias, blood pressure and platelet aggregation( Reference Flock, Rogers and Prabhu 107 , Reference Lee, O’Keefe and Lavie 109 , Reference Bermúdez Menéndez de la Granda and Sinclair 110 ). Studies have also shown that an n-3 PUFA-enriched diet may be able to inhibit neuroinflammation and delay oxidative stress and cell apoptosis( Reference Farooqui 101 , Reference Trépanier, Hopperton and Orr 111 ). Therefore, considering that inflammation and related changes are strongly linked to many chronic diseases (among them obesity), the ingestion of n-3 PUFA (mainly in the form of EPA and DHA) can play a fundamental role in preventing and treating these diseases( Reference Campbell and Bello 17 , Reference Simopoulos 96 , Reference Flock, Rogers and Prabhu 107 ).
n-3 PUFA and obesity
n-3 PUFA has the potential to induce a number of effects that may be useful for the treatment of obesity as this substance can attenuate weight gain and reduce body fat( Reference Buckley and Howe 15 ). Most studies on n-3 PUFA and obesity have focused on peripheral tissues, as well as on plasma and serum. n-3 PUFA have shown several beneficial effects on obesity-related changes in animals, such as reduced body-fat gain, reduced fat accumulation in visceral region, improved lipid profile, insulin resistance, glucose intolerance and hepatic steatosis, as well as inflammation reduction in peripheral tissues( Reference Rokling-Andersen, Rustan and Wensaas 20 , Reference Kalupahana, Claycombe and Newman 22 , Reference Liu, Xue and Liu 24 , Reference Rossmeisl, Medrikova and van Schothorst 27 , Reference Bargut, Mandarim-de-Lacerda and Aguila 28 ). The effect of n-3 PUFA in the brain alterations related to obesity was less studied, with hypothalamus being the major focus of the investigation. n-3 PUFA showed beneficial effects on modulating inflammation and hypothalamic function in rodents( Reference Cintra, Ropelle and Moraes 23 , Reference Pimentel, Lira and Rosa 26 , Reference Viggiano, Mollica and Lionetti 33 ) and was able to reverse the negative effect on the brain-derived neurotrophic factor (BDNF) gene expression caused by obesity in the hypothalamus of rats( Reference Abdel-Maksoud, Hassanein and Gohar 31 ). A review of animal studies on n-3 PUFA for obesity (and related alterations) is summarised in Table 1.
In humans with overweight or obesity, studies have shown that n-3 PUFA treatment can modulate adipose tissue, reduce adiposity, improve inflammation (adipose tissue and serum), reduce TAG and reduce oxidative stress in plasma, indicating that n-3 PUFA may be beneficial to help treat obesity( Reference Itariu, Zeyda and Hochbrugger 38 , Reference Kiecolt-Glaser, Belury and Andridge 40 , Reference Kiecolt-Glaser, Epel and Belury 41 ). A review of human studies on n-3 PUFA for obesity (and related alterations) is summarised in Table 2.
n-3 PUFA, especially EPA and DHA, have been shown to play an important role in the treatment of obesity( Reference Flock, Rogers and Prabhu 107 , Reference Flachs, Rossmeisl and Bryhn 112 , Reference Lorente-Cebrián, Costa and Navas-Carretero 113 ). A systematic review with meta-analysis conducted by Bender et al.( Reference Bender, Portmann and Heg 114 ) showed evidence that the consumption of fish or encapsulated fish oil (rich in n-3 PUFA) is related to slight reductions in body weight and waist circumference. However, the authors of that study concluded that further research is needed to clarify the possible mechanisms by which n-3 PUFA can lead to weight reductions( Reference Bender, Portmann and Heg 114 ).
A meta-analysis conducted by Du et al.( Reference Du, Jin and Fang 115 ) revealed that fish oil had no effect on the reduction of body weight and BMI in overweight or obese individuals. However, waist circumference and hip:waist ratio were significantly reduced in individuals taking fish oil supplementation, especially when combined with life modification intervention. The authors conclude that current evidence does not prove that fish oil intake may decrease body weight in overweight or obese adults, but that these individuals may benefit from reduced abdominal fat. They have also emphasised that the results should be treated with caution and suggested a large-scale investigation over a long period to draw definitive conclusions( Reference Du, Jin and Fang 115 ).
A more recent meta-analysis conducted by Zhang et al.( Reference Zhang, Liu and Zhao 116 ) also showed that a statistically non-significant difference was revealed in weight loss between n-3 PUFA and placebo, whereas n-3 PUFA might effectively reduce waist circumference and TAG levels in overweight and obese adults. The authors also suggested more studies to explore and clarify this issue( Reference Zhang, Liu and Zhao 116 ).
n-3 PUFA, especially EPA and DHA, can modulate adipocyte number by regulating adipocyte proliferation, differentiation and apoptosis. n-3 PUFA also regulates pathways related to fat storage and fat mobilisation, decreasing lipid accumulation processes and favouring adipocyte oxidative metabolism by promoting mitochondrial biogenesis and fatty acid oxidation. In addition, n-3 PUFA can modulate adipocyte insulin sensitivity and glucose utilisation( Reference Martínez-Fernández, Laiglesia and Huerta 108 ).
Other mechanisms by which EPA and DHA can help to treat obesity are related to the capacity of this substance to inhibit NF-κB activity and TLR-mediated inflammatory signalling( Reference Flock, Rogers and Prabhu 107 ). n-3 PUFA can also reduce adipose tissue inflammation by regulating the production of pro-inflammatory cytokines, by decreasing M1 macrophage infiltration and by reducing the formation of n-6-derived pro-inflammatory lipid mediators( Reference Martínez-Fernández, Laiglesia and Huerta 108 ). Fish oil supplementation can inclusively polarise macrophages and microglia towards the anti-inflammatory phenotype( Reference Bashir, Sharma and Elahi 117 , Reference Inoue, Tanaka and Masuda 118 ). Moreover, the treatment with n-3 PUFA can increase the production of lipid mediators with pro-resolution properties (resolvins, protectins and maresins) in adipose tissue( Reference González-Périz, Horrillo and Ferré 119 , Reference Neuhofer, Zeyda and Mascher 120 ) and promote the reduction of adipose tissue and systemic inflammation( Reference Itariu, Zeyda and Hochbrugger 38 ).
Inflammation also links obesity with the development of insulin resistance and hepatic steatosis( Reference Flock, Rogers and Prabhu 107 , Reference González-Périz, Horrillo and Ferré 119 ). The adverse metabolic changes associated with insulin resistance occur as a result of inflamed adipose tissue. Thus, EPA and DHA also may improve insulin sensitivity by generating pro-resolving lipid mediators and promoting alternatively activated macrophages( Reference Flock, Rogers and Prabhu 107 ). González-Périz et al.( Reference González-Périz, Horrillo and Ferré 119 ) showed that dietary intake of n-3 PUFA, by triggering the formation of n-3 PUFA-derived resolvins and protectins, had insulin-sensitising actions in adipose tissue and liver and improved insulin tolerance in obese mice, as well as alleviated hepatic steatosis( Reference González-Périz, Horrillo and Ferré 119 ).
Most studies utilise a combination of DHA and EPA as n-3 PUFA supplement, which may mask the effects of each individual fatty acid. The individual effect of EPA and DHA must be considered( Reference Huang, Chien and Chen 121 ). In a rodent study that compared EPA and DHA supplementation, Bargut et al.( Reference Bargut, Santos and Machado 36 ) showed that DHA had the most prominent action in white adipose tissue metabolism, modulating pro- and anti-inflammatory pathways and alleviating adipocyte abnormalities (caused by a high-fructose diet). In human studies comparing supplementation of EPA v. DHA, Allaire et al.( Reference Allaire, Couture and Leclerc 51 ) showed that, related to modulation of specific markers of inflammation and blood lipids, DHA is more effective than EPA. However, Vors et al.( Reference Vors, Allaire and Marin 53 ) showed that EPA or DHA has similar effects on the expression of many inflammation-related genes in immune cells of men and women at risk for cardiometabolic diseases.
As EPA and DHA can exert different effects, the ratio of EPA:DHA could affect the outcomes. It is observed that there is considerable variability in the ratio of EPA:DHA in the studies, and this should be taken into account. Shang et al.( Reference Shang, Liu and Zhou 122 ) investigated diets with different ratios of DHA:EPA (2:1, 1:1 and 1:2) and showed that a lower DHA:EPA ratio seems to be more beneficial for alleviation of high-fat-diet-induced liver damage in mice, and a DHA:EPA ratio of 1:2 mitigated the inflammatory risk factors.
The form of n-3 PUFA formulations (as TAG, ethyl ester, free fatty acid or phospholipid) could also affect the bioavailability and actions of n-3 PUFA. Tang et al. ( Reference Tang, Li and Xu 123 ) compared the effects ofTAG, ethyl ester, free fatty acid and phospholipid forms of n-3 PUFA (DHA) on lipid metabolism in mice (fed high-fat or low-fat diet) and showed that DHA-bound phospholipid showed effective bioactivity in decreasing hepatic and serum total cholesterol, TAG levels and increasing n-3 PUFA concentration in liver and brain. Rossmeisl et al.( Reference Rossmeisl, Jilkova and Kuda 124 ) showed that, compared with TAG, n-3 PUFA (DHA and EPA) administered as phospholipids are superior in preserving a healthy metabolic profile under obesogenic conditions, possibly reflecting better bioavailability and improved modulation of the endocannabinoid system activity in white adipose tissue.
Another important topic that should be mentioned is the ratio n-6/n-3 PUFA. As vegetable oils (soyabean, corn, sunflower, safflower and cottonseed oils), which are rich in n-6 PUFA, became popularised, there was a significant increase in n-6 PUFA intake( Reference Huang, Chien and Chen 121 ). Western diets contain excessive levels of n-6 PUFA and very low levels of n-3 PUFA, leading to an unhealthy n-6/n-3 ratio of 20:1, instead of 1:1 during evolution( Reference Simopoulos 125 ). A high n-6 intake and a high n-6:n-3 ratio is pro-thrombotic, pro-inflammatory and are associated with weight gain in both animal and human studies, whereas a high n-3 PUFA intake decreases the risk for weight gain( Reference Simopoulos 125 ). Therefore, a balanced n-6:n-3 ratio is also important for the prevention and management of obesity (it is essential to decrease the n-6 PUFA in the diet while increasing the n-3 PUFA)( Reference Simopoulos 125 ).
Conclusion
In conclusion, n-3 PUFA has shown a large number of effects that could be beneficial to treat obesity and related alterations. Animal and human studies have shown that in peripheral tissues (and in the blood) n-3 PUFA can reduce body and visceral fat, inflammation, hepatic steatosis and oxidative stress, as well as improve insulin sensitivity and lipid profile. In the brain, animal studies have shown that n-3 PUFA can reduce hypothalamic inflammation and apoptosis, improving hypothalamic function, and that it was able to reverse the negative effect on the BDNF caused by obesity in the hypothalamus. The effect of n-3 PUFA on body weight has shown contradictory results.
Therefore, the use of n-3 PUFA to help obesity treatment still needs further investigation, given that there are still many gaps on its effects, especially to clarify the mechanisms by which n-3 PUFA could help treat the disease. Most studies have focused on peripheral tissues, but brain aspects have been poorly explored. The focus has been only on the hypothalamus, and thus the effect of the n-3 PUFA on other brain structures has not been explored in animal models of obesity.
In addition, there were many differences in the methods of the studies, both with animals and with humans, which limit the comparison of the data and generalisation of the results. In general, it seems to us that the beneficial effect of n-3 PUFA would not be directly on weight, but on metabolic changes related to obesity.
Acknowledgements
This research received no specific grant from any funding agency or from commercial or not-for-profit sectors.
A. H. M. wrote the manuscript. M. F. U. assisted in writing and reviewing the tables, and revised the English grammar of the manuscript. B. X. F. and N. A. R. S. gave substantial contributions to data acquisition. G. T. R. revised the manuscript. All authors have read and approved the final version of the manuscript.
The authors declare that there are no conflicts of interest.