Introduction
Amazonia, the largest tropical forest, is paramount as a functioning ecosystem for human welfare. It covers 5.3 million km2 across nine South American countries and provides life-supporting resources such as food, water and medicines, regulates weather and rainfall, and stores carbon (Fearnside, Reference Fearnside2003, Reference Fearnside2017; Soares-Filho et al., Reference Schielein and Börner2006; Malhi et al., Reference Lovejoy and Nobre2008; Hubau et al., Reference Hubau, Lewis, Phillips, Affum-Baffoe, Beeckman and Cuní-Sanchez2020; Harris et al., Reference Harris, Gibbs, Baccini, Birdsey, Bruin and Farina2021). Nonetheless, 5 decades of continuous and unregulated expansion of agriculture and settlements have transformed southern Amazonia, adjacent ecotonal forests and the northern Cerrado into the largest global deforestation frontier (Kirby et al., Reference Jiménez-Muñoz, Mattar, Barichivich, Santamaría-Artigas, Takahashi and Malhi2006; FAO, 2016; Silva et al., Reference Sales, Santiago, Biggs, Mullan, Sills and Monteverde2019; Montibeller et al., Reference Miranda, Zago, Rubio, Santos, Ludwig, Passos and Miranda2020), known as the Arc of Deforestation, significantly affecting the functioning of the Amazonian ecosystem (Nobre et al., Reference Nepstad, McGrath, Alencar, Barros, Carvalho and Santilli2016; Gatti et al., Reference Gatti, Basso, Miller, Gloor, Domingues and Cassol2021).
There are 52 primate species in the Arc of Deforestation. The majority are endemic to this region and approximately half are threatened or near threatened with extinction (Costa-Araújo et al., Reference Costa-Araújo, Regolin, Martello, Souza-Alves, Hrbek and Ribeiro2021b). At the same time, there is a scarcity of baseline data on these primates, including a significant taxonomic deficit (Costa-Araújo et al., Reference Costa-Araújo, Melo, Canale, Hernández-Rangel, Messias and Rossi2019; Byrne et al., Reference Byrne, Costa-Araújo, Farias, Silva, Messias, Hrbek and Boubli2021), which hampers the protection of these species (Rylands & Mittermeier, 2014). An understanding of species diversity, distribution and abundance is therefore necessary to clarify and quantify extinction risks and to provide a scientific foundation for the conservation of the poorly studied primates endemic to the Arc of Deforestation.
Investment in research into and the conservation of primates is especially important as they play a vital role in ecosystem functioning (Peres et al., Reference Paim, Bizri, Paglia and Queiroz2016; Trolliet et al., Reference Silva2016; Heymann et al., Reference Heymann, Culot, Knogge, Tirado Herrera, Noriega Piña and Klapproth2017), and they are the most vulnerable vertebrate group globally (Arroyo-Rodríguez et al., Reference Arroyo-Rodríguez, González-Perez, Garmendia, Solà and Estrada2013, Reference Arroyo-Rodríguez, Galán-Acedo, Fahrig and Fuentes)2017), with c. 60% of all primate species threatened to some degree (Estrada et al., Reference Estrada, Garber, Rylands, Roos, Fernandez-Duque and Di Fiore2017). Moreover, primates are valuable as flagship species (Dietz et al., Reference Defler and García1994; Chapman et al., Reference Chapman, Bicca-Marques, Dunham, Fan, Fashing and Gogarten2020), and so the protection of their habitats in the Arc of Deforestation will not only contribute to their conservation but also provide protection to other species endemic to this region, contributing to biodiversity conservation with impacts at local, regional and global scales.
Here we identify the areas of suitable habitat, delimit the geographical distribution, estimate the population size and assess the conservation status of Vieira's titi monkey Plecturocebus vieirai (Plate 1). This species was recently discovered in the Arc of Deforestation (Gualda-Barros et al., Reference Gualda-Barros, Nascimento and Amaral2012) but is known only from its pelage colour and occurrence in three localities and is therefore considered Data Deficient (Alonso et al., Reference Alonso, Boubli and Miranda2018; IUCN, 2021). Using our findings, we discuss the threats to primates and the opportunities for biodiversity conservation coupled with climate change mitigation and income generation in the Arc of Deforestation using P. vieirai as an emblematic example, a species that we assess to be Critically Endangered based on the IUCN Red List criteria (IUCN, 2019).
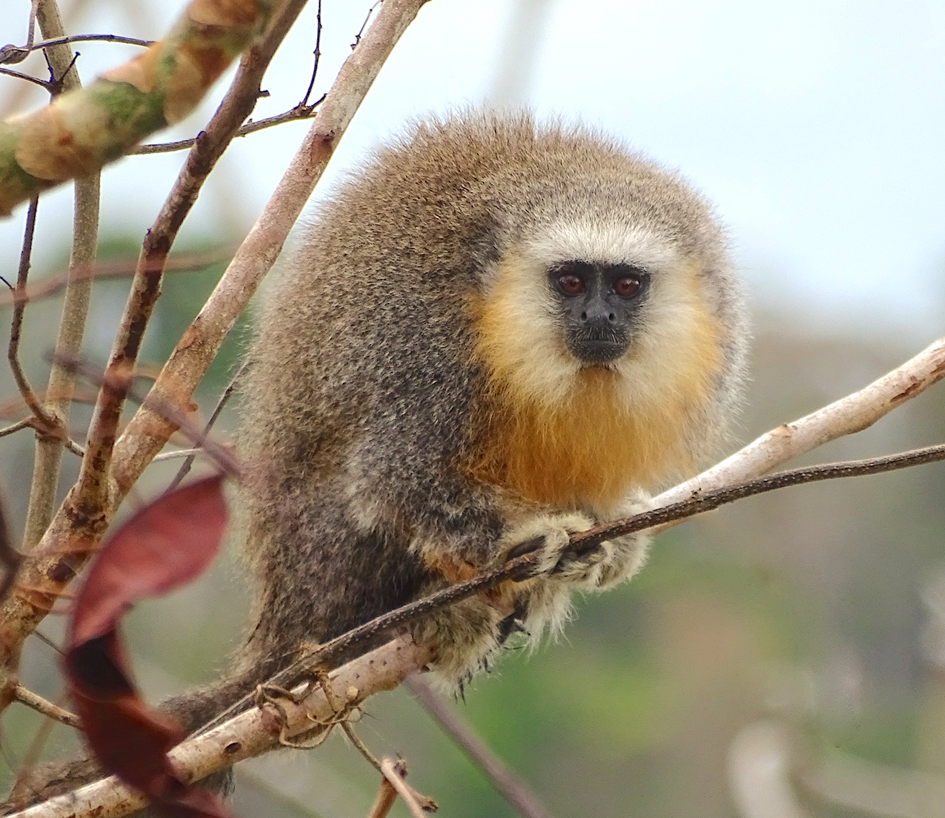
Plate 1 Vieira's titi monkey Plecturocebus vieirai. Photo: F. Reis.
Study area
The study area is located in the Tapajós–Xingu interfluve, a region within the Arc of Deforestation that is especially susceptible to land-use change (Laurance et al., Reference Kriticos, Webber, Leriche, Ota, Macadam and Bathold2002; Fig. 1). The Arc of Deforestation extends across southern Amazonia, ecotonal forests and the northern Cerrado from its eastern edge in the states of Pará and Maranhão to the state of Acre in the west (Fearnside et al., Reference Fearnside, Righi, Graça, Keizer, Cerri and Nogueira2009; Silva et al., Reference Sales, Santiago, Biggs, Mullan, Sills and Monteverde2019). This region accounts for almost half of the total global land-use changes during 1990–2015 (FAO, 2016). Although sustainable development was expected for the Tapajós–Xingu region 2 decades ago (Nepstad et al., Reference Mourthé, Hilário, Carvalho and Boubli2002), it became an epicentre of deforestation as a result of logging and slash-and-burn clearance for agriculture and cattle ranching as well as legal and illegal gold mining, affecting even protected areas and Indigenous lands (Printes, Reference Peres and Cunha2017; Montibeller et al., Reference Miranda, Zago, Rubio, Santos, Ludwig, Passos and Miranda2020).
Methods
Data collection
We collected new occurrence records in field expeditions within the Tapajós–Xingu interfluve during 2015–2019 to model areas of suitable habitat and to delimit the geographical distribution of P. vieirai. We reviewed the literature and examined specimens housed in museum collections in Brazil, the USA and Europe for additional occurrence records. To estimate the size and density of the P. vieirai population, we surveyed four areas during 2016–2018 using the linear transect method (Buckland et al., Reference Buckland, Anderson, Burnham and Laake1993). In each area we established a transect of 5 km covering mature and secondary forests. Each transect was surveyed at a constant speed of c. 1.25 km/h twice daily, at 07.00–11.00 and 14.00–18.00 by DAS, LFS and RMA. We noted the number of individuals and the perpendicular distance between the group and the transect using a GPS and a measuring tape; we considered each of the two daily surveys to be independent (Peres & Cunha, Reference Nogueira, Yanai, Vasconcelos, Graça and Fearnside2011). The four forest areas surveyed are on the east bank of the middle Teles Pires River in the municipalities of Cláudia and Sinop, Mato Grosso State, Brazil, c. 50 km from the the type locality of P. vieirai.
Data analysis
We used the occurrence records, and environmental variables from WorldClim 2.1 (Fick & Hijmans, Reference Fick and Hijmans2017) and CliMond (Kriticos et al., Reference Kirby, Laurance, Albernaz, Götz, Fearnside and Bergen2012), to model habitat suitability and delimit the geographical distribution of P. vieirai. We selected only spatially independent records (n = 33) from our dataset (n = 99 records), applying a threshold of 10 km between records. We eliminated autocorrelated environmental variables to avoid model overfitting (Pearson's correlation test r > 0.80, P < 0.05; Supplementary Table 1; Callegari-Jacques, Reference Callegari-Jacques2003; Mateo et al., Reference Marques, Marimon-Junior, Marimon, Matricardi, Mews and Colli2013). We converted the 11 environmental variables selected to a 2.5-min scale using the raster package (Hijmans & Etten, Reference Hijmans and Etten2012) in R 4.1 (R Core Team, Reference Peres, Emilio, Schietti, Desmoulière and Levi2018). We modelled habitat suitability using four algorithms adequate for the type of data available (presence and pseudo-absence records), using the Biomod2 package (Thuiller et al., Reference Silva, Kawanishi, Henschel, Kittle, Sanei and Reebin2016) in R: artificial neural networks (Ripley, Reference Phillips, Anderson and Shapire1996), generalized boosted models (Friedman, Reference Friedman2001), random forest (Breiman, Reference Breiman2001) and maximum entropy (Phillips et al., Reference Pegas and Castley2006). We selected these four algorithms to avoid model overfitting that could result from the use of algorithms suitable for presence-only and presence and true absence records from long-term surveys (Andrade et al., Reference Andrade, Velazco and Júnior2020; Silva et al., Reference Ripley2020). For each algorithm we established five datasets, each composed of 10,000 background records randomly distributed throughout the study area. We then used 70% of the records for training and 30% for evaluating model fitting. In total we performed 200 runs (four algorithms, 10 runs of cross-validation, five sets of random background points), with 1,000 iterations each. To check the accuracy of models we used true skill statistical (TSS) analysis (Allouche et al., Reference Allouche, Tsoar and Kadmon2006) and the area under the curve value (AUC) of the receiver operating characteristic curve, incorporating a binomial probability as a null model (Phillips et al., Reference Pegas and Castley2006). The AUC and TSS values vary from 0 to 1 based on the specificity and sensitivity of the species’ response to environmental variables. We selected models with AUC > 0.7 and TSS > 0.4 (Buisson et al., Reference Buisson, Thuiler, Casajus, Lek and Grenouillet2010) using the mean suitability value of each grid (2.5-min scale) and the minimum omission method (Silva et al., Reference Russon, Wallis, Russon and Wallis2017, Reference Ripley2020). To identify the environmental variables that best explain the occurrence of the species we used the jackknife test (Phillips et al., Reference Pegas and Castley2006).
Using ArcGIS 10.1 (Esri, Redlands, USA), we delimited the geographical distribution of P. vieirai from the occurrence records, the ensemble model, knowledge of the distribution of congeneric, neighbouring species and considering that rivers restrict the occurrence of primate species in Amazonia (Mourthé et al., Reference Mourthé, Hilário, Carvalho and Boubli2022). Finally, we overlaid the species distribution with raster layers of vegetation classes (Souza et al., Reference Silva, Costa, Rossi, Vale, Lima and Lima2020), elevation (which is a constraint for species occurrence; Jarvis et al., 2008) and forest cover (Soares-Filho et al., Reference Schielein and Börner2006), to extract the area of suitable habitat available for P. vieirai in the present (2020) and in the future (2044). We used a threshold of 24 years, representing three P. vieirai generations (Veiga et al., Reference Soares-Filho, Nepstad, Curran, Cerqueira, Garcia and Ramos2011; Defler & García, Reference Crouzeilles, Valle, Cerqueira and Grelle2012), as a baseline to estimate the availability of suitable habitat in the future and to assess the species' conservation status. We calculated the extent of occurrence and the area of suitable habitat (Brooks et al., Reference Brooks, Pimm, Akçakaya, Buchanan, Butchart and Foden2019) in 2020 and in 2044 using the R package red (Cardoso, Reference Cardoso2017, Reference Cardoso2018). We validated the area of suitable habitat using our dataset of location records overlain on a binary map of presence or absence predicted from the environmental variables.
We used the data from our linear transect surveys to estimate population density and sighting rates with Distance 7.1 (Thomas et al., Reference Silva, Fernandes-Ferreira, Montes and Silva2010). We carried out this analysis by pooling all sightings from the four survey areas using the hazard-rate model, a simple polynomial adjustment and 45 m as the effective sighting distance (χ 2 = 0.80; df = 3; P = 0.84); the final model was selected using the Akaike information criterion. We used the oldest available satellite data of forest cover over the species range (1985; Souza et al., Reference Silva, Costa, Rossi, Vale, Lima and Lima2020) as a conservative baseline to extract an approximate estimate of the original habitat area of P. vieirai.
Results
We gathered 96 occurrence records of P. vieirai in addition to the three records available from the species description: 90 from fieldwork, four from the literature (Miranda et al., Reference Mateo, Vanderpoorten, Munoz, Laenen and Desamore2014; Vendramel, Reference Souza, Shimbo, Rosa, Parente, Alencar and Rudorff2016) and two from specimens stored in the Museu Paraense Emílio Goeldi (MPEG 246, 21837; Supplementary Table 2). All records are from 150–410 m altitude and within the boundaries of Amazonian forests of the Tapajós domain, except for three records that fall within the boundaries of the Cerrado (Instituto Brasileiro de Geografia e Estatística, 2019) but were obtained in the Amazonia–Cerrado ecotonal forest (Silva et al., Reference Sales, Santiago, Biggs, Mullan, Sills and Monteverde2019). We also gathered a new record of Plecturocebus moloch on the north bank of the Cristalino River (9.552926° S, 55.928568° W; Fig. 2), which is the southernmost documented record of this species in the Tapajós–Xingu interfluve and helped us to delimit the geographical distribution of P. vieirai, which is expected to occur on the opposite bank of the river according to our modelling (see below).
Our combined results from habitat modelling and occurrence records show that P. vieirai occurs in an area of 226,054 km2 and is limited in the east by the Xingu River, in the north by the Irirí River, in the west by the Irirí, Cristalino and Teles Pires rivers and extending southwards to the upper Teles Pires and Xingu rivers (Fig. 2). The linear transect surveys totalled 1,192 km of trails walked, with 78 group encounters and 86 individuals observed in the four areas, resulting in a mean density of 0.03 groups/ha and 0.07 individuals/ha (Table 1), with mean group sizes ranging from 1.5 (95% CI 1.0–4.2) to 2.62 (95% CI 2.13–3.23).
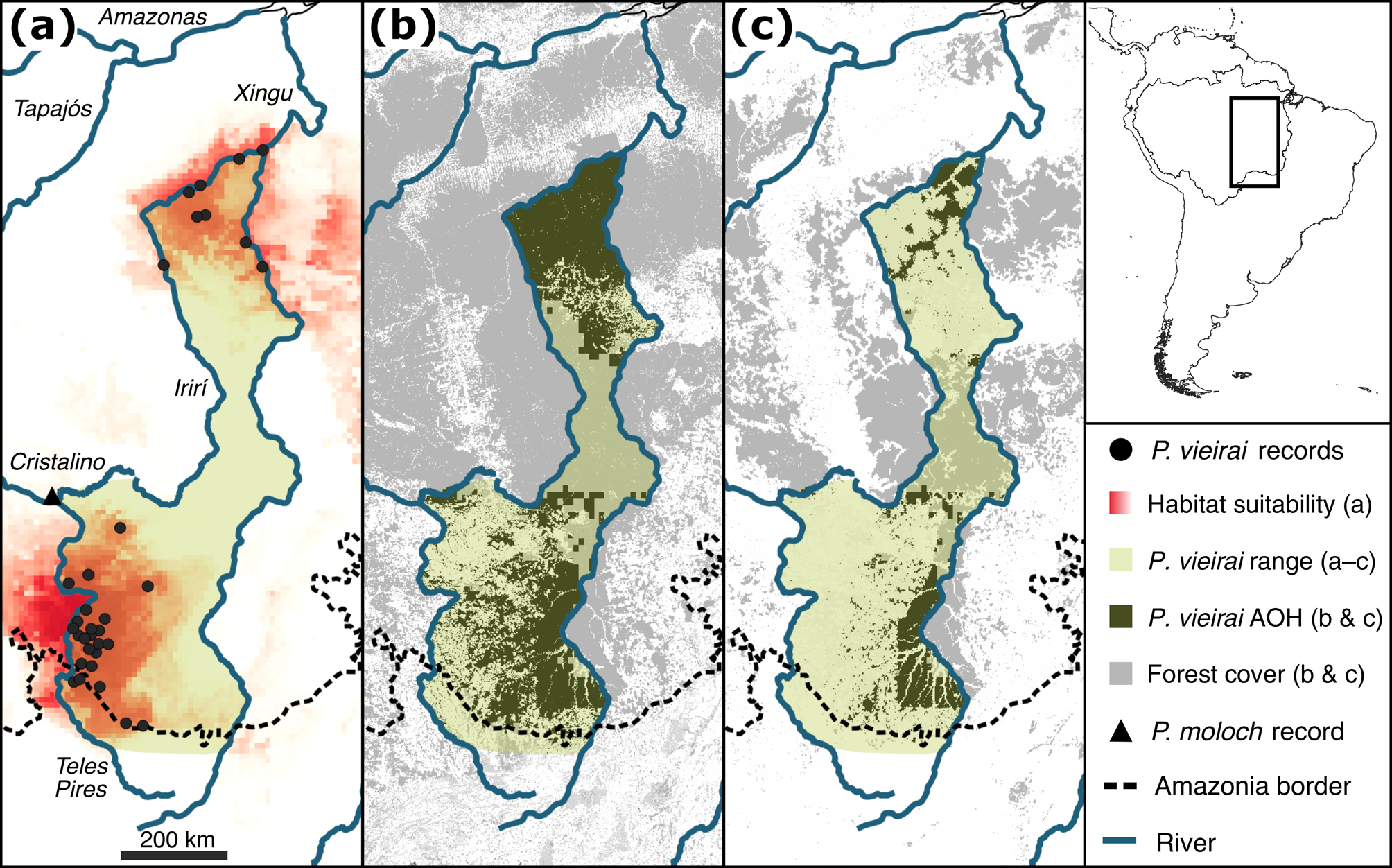
Fig. 2 Geographical distribution and potential habitat of Plecturocebus vieirai in the Tapajós–Xingu interfluve: (a) records of P. vieirai and Plecturocebus moloch, and habitat suitability modelled using environmental variables; (b) suitable forest habitat area in 2020; (c) modelled potentially suitable habitat area in 2044. AOH, area of habitat (based on environmental suitability and forest availability).
Table 1 Survey effort, number of sightings, sighting rates and density estimates (per ha) for Vieira's titi monkey Plecturocebus vieirai obtained during 3 years (2016–2018) of systematic transect surveys in the southern part of the species range, Cláudia and Sinop municipalities, Mato Grosso State, Brazil.

1 Locality names as per Supplementary Table 2.
Considering the data on past and projected future forest cover (Soares-Filho et al., Reference Schielein and Börner2006; Souza et al., Reference Silva, Costa, Rossi, Vale, Lima and Lima2020) and our new range delimitation for P. vieirai, in 1985 forests covered 90% of the range of P. vieirai (204,191 km2) but in 2044 only 30% (54,731 km2) of these forests will remain. Our modelling shows that suitable habitat (Table 2) for P. vieirai is currently 56% of the original area and that 58% of this is within protected areas and Indigenous lands. By 2044, according to our model, only 14% of the original P. vieirai habitat will remain, 88% within protected areas and Indigenous lands.
Table 2 Extent of occurrence, suitable habitat, suitable habitat in protected areas and forest cover for P. vieirai in 2020 and after three P. vieirai generations (2044), with actual (2020) or predicted habitat loss (2044).

1Relative to original forest cover in 1985.
2Relative to suitable habitat.
We found that variables related to rainfall (specifically the wettest quarter and the driest month) explain 60% of the likelihood of P. vieirai occurrence (AUC = 0.999, TSS = 0.996) and therefore these variables are the best indicator of habitat suitability for this species (Supplementary Table 3).
Discussion
Our findings indicate that habitat loss as a result of conversion of forest into large-scale agricultural monocultures and pastures for cattle ranching is the main threat to P. vieirai. Our model showed that because of deforestation the habitat of P. vieirai has been reduced by 56% and only 14% will remain by 2044. We expect a concomitant decline in the population size of P. vieirai. We assess P. vieirai to be Critically Endangered (Costa-Araújo et al., Reference Costa-Araújo, Melo, Silva Júnior, Buss, Boubli, Hrbek and Canale2022) based on IUCN criterion A3bc (IUCN, 2019), given that our estimates suggest a projected population size reduction of ≥ 80% within the next three P. vieirai generations (A3), and that these estimates are based on an index of abundance that is appropriate for the taxon (b) and a decline in the area of occupancy of > 80% (c).
Additionally, decreases in the suitability of any remaining habitat because of climate change compound the threats to the long-term survival of P. vieirai. Greenhouse gas emissions from human activities are causing extended dry seasons, increased frequencies of droughts and forest fires and reductions in rainfall, forest biomass and net primary production in southern Amazonia (Jiménez-Muñoz et al., Reference Jarvis, Reuter, Nelson and Guevara2016; Fearnside, Reference Fearnside2017; Brando et al., Reference Brando, Soares-Filho, Rodrigues, Assunção, Morton and Tuchschneider2020; Sales et al., Reference Sales, Santiago, Biggs, Mullan, Sills and Monteverde2020; IPCC, 2021). Conversely, we found that precipitation has a positive relationship with habitat suitability for P. vieirai, and forest biomass and primary production also have a positive relationship with titi monkey occurrence (Costa-Araújo et al., Reference Costa-Araújo, Melo, Silva Júnior, Buss, Boubli, Hrbek and Canale2021a). Therefore, climate (IPCC, 2021) and forest (Soares-Filho et al., Reference Schielein and Börner2006) changes projected for southern Amazonia will negatively affect the biotic and abiotic dimensions of the P. vieirai niche in the next quarter of a century, further exacerbating the threats to the species’ long-term survival.
Habitat loss from deforestation and degradation because of climate change have distinct implications for the conservation of P. vieirai populations at the northern and southern extents of the species’ range. Deforestation and forest fires are and will remain high (Soares-Filho et al., Reference Schielein and Börner2006; Brando et al., Reference Brando, Soares-Filho, Rodrigues, Assunção, Morton and Tuchschneider2020) in the southern portion of the range of P. vieirai, where most forests are on private lands and where there are few and only small protected areas. Although deforestation has been and is expected to continue to be low (Soares-Filho et al., Reference Schielein and Börner2006) in the northern portion of the range of P. vieirai because of the existence of an extensive system of protected areas and Indigenous lands, this habitat is not safe from degradation driven by climate change.
Therefore, the populations in the southern portion of the range of P. vieirai require immediate conservation efforts. Beyond protecting primate populations (Paim et al., Reference Nepstad, Schwartzman, Bamberger, Santilli, Ray and Schlesinger2019), the demarcation of Indigenous lands and establishment of public and private protected areas in the Tapajós–Xingu interfluve would safeguard other endemic species and protect representative tracts of Amazonia and the Amazonia–Cerrado ecotone, a unique and almost entirely unprotected ecosystem (Nepstad et al., Reference Moutinho, Guerra and Azevedo-Ramos2006; Marques et al., Reference Malhi, Roberts, Betts, Killeen, Li and Nobre2019). These protected areas would also contribute to reducing the extent of land-use change and fire regimes in this region, maintain carbon stocks and sinks and generate income through REDD+ projects (Nogueira et al., Reference Nogueira, Yanai, Vasconcelos, Graça and Fearnside2018). For the same reasons, the effective protection of reservas legais (an area of 80% that legally must be preserved as forest in all private land holdings in Brazilian Amazonia) needs to be enforced and private landowners rewarded appropriately (Schielein & Börner, 2018).
Law enforcement, the establishment of public protected areas and the demarcation of Indigenous lands rely on governmental administration, but changes in policies and demonstrated inaction and a lack of regulation have resulted in increasing threats to Amazonian forests, biodiversity and traditional peoples in Brazil (Ferrante & Fearnside, Reference Ferrante and Fearnside2019; Begotti & Peres, Reference Begotti and Peres2020). Moreover, the establishment of private protected areas and the protection of reservas legais are unattractive to landowners profiting from the conversion of forests into agricultural landscapes in the Tapajós–Xingu region (Crouzeilles et al., Reference Costa-Araújo, Silva, Boubli, Rossi, Canale and Melo2012; Printes, Reference Peres and Cunha2017). The model of income generation and the policies in Brazil that permit unsustainable extraction of natural resources in the Arc of Deforestation needs to shift towards the protection of the livelihoods of local people, biodiversity, climate change mitigation and the maintenance of the ecosystem services provided by Amazonia (Moutinho et al., Reference Montibeller, Kmoch, Virro, Mander and Uuemaa2016; Nobre et al., Reference Nepstad, McGrath, Alencar, Barros, Carvalho and Santilli2016; Carvalho et al., Reference Carvalho, Mustin, Hilário, Vasconcelos, Eilers and Fearnside2019).
In this context, private landowners are the key stakeholders (Nepstad et al., Reference Mourthé, Hilário, Carvalho and Boubli2002; Soares-Filho et al., Reference Schielein and Börner2006, Fearnside, Reference Fearnside2017) for biodiversity conservation and the protection of representative tracts of remaining forests in the Arc of Deforestation, which would contribute to climate change mitigation (Fearnside, Reference Fearnside2003, Reference Fearnside2009; Nogueira et al., Reference Nogueira, Yanai, Vasconcelos, Graça and Fearnside2018). The owners of large farms are responsible for 70% of deforestation in private areas in Amazonia (Fearnside, Reference Fearnside2017) but could change their model of land use, which is currently putting at risk the ecosystem services and the resources provided by the biome (Lovejoy & Nobre, Reference Laurance, Albernaz, Schroth, Fearnside, Bergen and Venticinque2018). The official custodians of natural resources in protected areas (traditional and native peoples, local and federal governments) are also key conservation stakeholders (Nepstad et al., Reference Moutinho, Guerra and Azevedo-Ramos2006).
As an important tool for the conservation of species and habitats (Russon & Wallis, Reference Printes2014), we believe that primate-watching tourism could be a viable, sustainable alternative to monoculture agriculture and cattle-ranching expansion in the Arc of Deforestation. Firstly, there is a wealth of opportunities for primate watching in the Arc of Deforestation, with 52 species of primates (Costa-Araújo et al., Reference Costa-Araújo, Regolin, Martello, Souza-Alves, Hrbek and Ribeiro2021b). Secondly, primate-watching services on private lands, public protected areas and Indigenous lands are permitted under Brazilian environmental regulations. Thirdly, the logistics to facilitate ecotourism in the Arc of Deforestation are met by a network of roads, airports and lodging facilities. Additionally, regulations for the creation of private protected areas in Brazil are compatible with primate-watching tourism and biodiversity protection (Crouzeilles et al., Reference Costa-Araújo, Silva, Boubli, Rossi, Canale and Melo2012). This scenario would facilitate the establishment of primate-watching activities in protected areas, Indigenous lands and private areas within the Arc of Deforestation, a region where logistic capacity (one of the main constraints on ecotourism; Pegas & Castley, Reference Nobre, Sampaio, Borma, Castilla-Rubio, Silva and Cardoso2014) exists.
For example, a shift from agriculture to primate watching focused on P. vieirai in the private lands of the Tapajós–Xingu interfluve would contribute to reducing habitat loss in the southern portion of the range of P. vieirai and to mitigating climate change, in turn contributing to protecting P. vieirai populations in the northern part of its range. It is equally important to elect politicians committed to biodiversity conservation, the welfare of traditional peoples and climate change mitigation (Silva, Reference Rylands and Mittermeier2005; Carvalho et al., Reference Carvalho, Mustin, Hilário, Vasconcelos, Eilers and Fearnside2019), so that all key stakeholders can work together to improve outcomes for natural areas in the largest global deforestation frontier (Moutinho et al., Reference Moutinho, Guerra and Azevedo-Ramos2018).
Conclusion
Deforestation, mainly driven by expansion of monoculture agriculture and cattle ranching, is a ubiquitous threat to P. vieirai, responsible for the past, present and projected future habitat losses and consequent decreases in the populations of this species. These types of land use in the Arc of Deforestation have contributed directly to climate change, another major threat to P. vieirai, which we expect to cause habitat degradation and population declines even within protected areas.
Primate watching could be a profitable and sustainable alternative to the exploitation and overexploitation of natural resources on private, public and Indigenous lands and could be a way to shift from the current land-use model in the Arc of Deforestation towards biodiversity conservation. In the long term, the forests protected for and by tourism will serve as carbon stocks and sinks, contributing to the mitigation of climate change.
The traditional model of predatory extraction of natural resources in the Arc of Deforestation is pushing primate species to the brink of extinction and transforming Amazonia into a source, rather than a sink, of carbon emissions. An alternate model of land use and income generation is needed if we are to protect the unique natural and human heritages of Amazonia and its life-supporting ecosystem services and products.
Acknowledgements
We thank Conselho Nacional de Desenvolvimento Científico e Tecnológico (140039/2018-1; 316321/2020-6), Coordenação de Aperfeiçoamento de Pessoal de Nível Superior (CAPES; 001; 88881.189052/2018-01) and Fundação de Amparo à Pesquisa do Estado do Amazonas for scholarships; the Conservation Leadership Programme, Global Wildlife Conservation's Margot Marsh Primate Action Fund and CAPES AUX/PE 3261/2013 for funding fieldwork; Idea Wild, Consórcio Usina Hidroelétrica Teles Pires and Consórcio Usina Hidroelétrica Sinop for supporting fieldwork; and Jessica dos Anjos for providing the record of Plecturocebus moloch at the Cristalino River. RC-A's field data were collected during his doctorate research at the Programa de pós-graduação em Ecologia of the Instituto Nacional de Pesquisas da Amazônia, and he led the writing while affiliated with the Mastozoology Collection of the Museu Paraense Emílio Goeldi.
Author contributions
Conception: RC-A, GRC; data collection design: RC-A, FRM, RVR, GRC; data collection: all authors; mapping and species distribution modelling: LGS, FPS, with inputs from RC-A; population density analysis: FRM; writing: RC-A, with inputs from all authors.
Conflicts of interest
None.
Ethical standards
Fieldwork followed the code of best practices for field primatology of the International Society of Primatologists and otherwise abided by the Oryx guidelines on ethical standards.