There are sex-dependent physiological differences in erythropoiesis and lipoprotein metabolism, as well as liver and kidney function in adulthood (Kasarinaite et al., Reference Kasarinaite, Sinton, Saunders and Hay2023; Murphy, Reference Murphy2014). These differences are largely mediated by sex hormones, principally testosterone and estradiol, the most potent androgen and estrogen respectively (Kasarinaite et al., Reference Kasarinaite, Sinton, Saunders and Hay2023; Palmisano et al., Reference Palmisano, Zhu, Eckel and Stafford2018; Palmisano et al., Reference Palmisano, Zhu and Stafford2017).
Erythropoietic activity is generally higher in males than in similarly matched females due to the activity of androgens. The average adult female hemoglobin is about 12% lower than in similarly matched males (Murphy, Reference Murphy2014). The mechanism of androgen action on erythropoiesis is not fully understood; however, it has been observed that testosterone may stimulate erythrocytosis by increasing the relative concentration of erythropoietin (EPO) while suppressing hepcidin (Bachman et al., Reference Bachman, Travison, Basaria, Davda, Guo, Li, Connor Westfall, Bae, Gordeuk and Bhasin2014). The suggested mechanisms may include testosterone-stimulating EPO secretion through hypoxic sensing or the induction of hypoxia. Alternatively, testosterone could participate in renal physiology by acting on renal peritubular fibroblasts to release EPO (Bachman et al., Reference Bachman, Travison, Basaria, Davda, Guo, Li, Connor Westfall, Bae, Gordeuk and Bhasin2014). Moreover, testosterone could also induce erythrocytosis by acting directly on bone marrow erythroblasts or by increasing red cell survival. EPO activity increases cell mass or erythrocytosis by suppressing hepcidin to increase iron availability. These observed androgenic mechanisms, however, are not universal, as previous studies have indicated otherwise (Bachman et al., Reference Bachman, Travison, Basaria, Davda, Guo, Li, Connor Westfall, Bae, Gordeuk and Bhasin2014; Guo et al., Reference Guo, Schmidt, Fleming and Bhasin2020).
Similarly, sex-dependent physiological lipid homeostasis, liver functions and kidney functions are mediated by sex hormones. Sex hormones exert their influence on hepatic and renal cells through receptor-dependent signaling via either genomic or nongenomic pathways (Kasarinaite et al., Reference Kasarinaite, Sinton, Saunders and Hay2023). Estrogens act on cells through the alpha (ERα) and beta (ERβ) receptors. Concerning the liver, mice devoid of ERα were found to have increased insulin resistance and increased lipid storage. Androgens act through the androgen receptors (AR) on cells. The activation of androgen receptors on hepatocytes results in increased lipid synthesis and reduced fatty acid β-oxidation (Kasarinaite et al., Reference Kasarinaite, Sinton, Saunders and Hay2023). The role of estrogen in lipid homeostasis has been established. Premenopausal women tend to have reduced cardiovascular risk when compared to similarly aged men, postmenopausal women r women with polycystic ovarian syndrome (Palmisano et al., Reference Palmisano, Zhu, Eckel and Stafford2018). This is because estrogen is positively associated with increased hepatic synthesis of more and larger circulating HDL particles, which are involved in transporting cholesterol back to the liver for excretion through their conversion to bile salts in a process known as reverse cholesterol transport (Palmisano et al., Reference Palmisano, Zhu, Eckel and Stafford2018; Palmisano et al., Reference Palmisano, Zhu and Stafford2017). Additionally, estrogen facilitates the delivery of triglyceride-rich VLDL to the liver, enabling the liver to transport triglycerides to prevent their accumulation. Thus, the production of triglycerides and VLDL may be higher in females than in males; however, their clearance from circulation is faster in females due to estrogen-induced lipoprotein lipase activity. Estrogen, unlike testosterone, may play a hepato-protective role in liver physiology or pathophysiology (Palmisano et al., Reference Palmisano, Zhu, Eckel and Stafford2018; Wang et al., Reference Wang, Magkos and Mittendorfer2011). There is a sex-dependent difference in kidney physiology and function in adulthood. The prevalence of chronic kidney diseases (CKD) is said to be higher in women than in similarly aged men; however, the prevalence of end-stage renal disease (ESRD) is relatively higher in men (Bairey Merz et al., Reference Bairey Merz, Dember, Ingelfinger, Vinson, Neugarten, Sandberg, Sullivan, Maric-Bilkan, Rankin, Kimmel and Star2019). The progression of renal diseases seems higher in men than in women, although men have an increased capacity than women to maintain the glomerular filtration rate (GFR) by increasing the filtration fraction (Xu et al., Reference Xu, Zhang, Zhang, Wang, Zuo and Wang2010). The relatively slow progression of renal diseases in women is attributable to the reno-protective function of estrogen (Bairey Merz et al., Reference Bairey Merz, Dember, Ingelfinger, Vinson, Neugarten, Sandberg, Sullivan, Maric-Bilkan, Rankin, Kimmel and Star2019; Goldberg & Krause, Reference Goldberg and Krause2016).
The Twin Testosterone Transfer (TTT) hypothesis suggests that opposite-sex (OS) females are exposed to relatively higher prenatal testosterone than same-sex (SS) females. This occurs as a female fetus gestates near her male co-twin, who has higher levels of prenatal testosterone (Ahrenfeldt et al., Reference Ahrenfeldt, Christensen, Segal and Hur2020). It has been suggested that there could be a fetal-fetal transfer of testosterone since amniotic fluid can permeate the fetal skin and the placenta until week 18 of gestation when testosterone production in male fetuses may peak (Zheng & Cohn, Reference Zheng and Cohn2011). According to the Organizational hypothesis, prenatal exposure to testosterone may cause masculinization in the brain, leading to the development of male-typical traits. Evidence of male-typical traits in females exposed to prenatal testosterone can be seen in congenital adrenal hyperplasia (CAH), a condition characterized by altered steroidogenesis due to 21-hydroxylase deficiency (Richards et al., Reference Richards, Browne, Aydin, Constantinescu, Nave, Kim and Watson2020). Aside from developing male-typical cognitive and behavioral traits, females exposed to excess prenatal testosterone may also experience male-typical physiological or pathophysiological functions of the liver, kidney and lipid homeostasis, since prenatal testosterone exposure may correlate positively with current circulating testosterone in adulthood (Claahsen-van der Grinten et al., Reference Claahsen-van der Grinten, Speiser, Ahmed, Arlt, Auchus, Falhammar, Flück, Guasti, Huebner, Kortmann, Krone, Merke, Miller, Nordenström, Reisch, Sandberg, Stikkelbroeck, Touraine and Utari2022; Ronalds et al., Reference Ronalds, Phillips, Godfrey and Manning2002). These observations, however, are not universal, as previous findings are either conflicting or negative, or produced smaller effect sizes (Cohen-Bendahan et al., Reference Cohen-Bendahan, Buitelaar, Van Goozen and Cohen-Kettenis2004; Marczak et al., Reference Marczak, Misiak, Sorokowska and Sorokowski2018; Medland et al., Reference Medland, Loehlin and Martin2008)
Traditionally, no separate reference intervals are provided for SS and OS females when performing routine hematological and biochemical laboratory investigations. However, if the TTT hypothesis is true, then it is expected that OS females will have male-typical reference values due to their relative exposure to prenatal testosterone. Hitherto, no known study has tested the TTT hypothesis in Ghana regarding hematological and biochemical variables. The study aims to explore the differences in hematological and biochemical variables between SS and OS females.
Material and Methods
Study Design and Population
The study, conducted from January to September 2022 in Tamale, employed a cross-sectional design. Tamale, the largest city in the northern part of Ghana, is characterized by a diverse cultural landscape, with the major cultural group being the Dagomba, forming part of the larger linguistic group known as the Mole-Dagomba. Participants were young adults who were recruited from the Tamale Metropolitan area of the Northern region by convenient sampling. Twins who were known to the researchers and met the eligibility criteria were contacted for their consent before enlistment to participate in the study. In addition, through word of mouth from those enlisted or other persons, other twins were contacted and enlisted. The twins identified ancestrally as Ghanaians whose parents and grandparents were indigenous Ghanaians. The study was faced with the logistical challenge of having young adult twins live separately, often pursuing educational or professional engagements. The study included 10 dizygotic (DZ) pairs of twins comprising 10 OS males (25%) and 10 OS females (25%). In addition, 10 pairs of SS females, comprising 20 individual female twins (50%), were also recruited. The twins were aged from 18 to 27 years, with no known history of chronic diseases or hormonal abnormalities that could significantly impact hematological and biochemical measurements. The OS male and OS female pairs were clearly DZ twins. However, determining the zygosity status of the SS females was not possible due to the challenges mentioned earlier.
Data Collection and Measurements
Socio-demographic data were collected through an interviewer-administered semi-structured questionnaire. Measurements of standing height and body weight were taken with a stadiometer and body weighing scale to the nearest 0.1 cm and 0.1 kg respectively. Each participant stood bare-foot with the hills, buttocks, back and the back of the head touching the scale. The hands were on the side and the head was positioned in the Frankfort plane. The stadiometer plate was then lowered gently to touch the head and the height was then recorded. The body weight was measured with the participant wearing light clothing, and bare foot with the hands on the side. The body mass index (BMI) was then calculated using the formula body weight/(height (m)2. Fasting venous blood samples were collected into ethylenediaminetetraacetic acid (EDTA) and gel-separator tubes for a complete blood count analysis using the Norma Icon-5 automated hematology analyzer. Serum samples, obtained by clotting the gel-separator tube samples, were stored at −20°C until analysis. Serum testosterone levels were measured using the ELISA technique (Monobind Inc., Lake Forest, CA, USA), while biochemical variables were determined using a BT 1500 automated biochemistry analyzer (Biotechnica Instruments, SPA, Italy). All sample collections were conducted between 8:00 am and 12:00 pm local time to minimize diurnal variability.
Statistical Analysis
Data were entered into an Excel spreadsheet and analyzed using SPSS (v27). The distribution of data was assessed with the Shapiro-Wilk test, and categorical data were summarized as frequency (percentage), while parametric continuous variables were presented as mean ± standard deviation. It was expected that OS male variables would either be higher or lower for hematological or biochemical parameters. Similarly, OS female variables were expected to follow male-typical patterns. Therefore, a one-tailed t tests was performed. SPSS lacks a dedicated procedure or dialog box specifically designed for conducting a one-tailed test for differences in means. The process for a one-tailed test aligns with that of a two-tailed test. To obtain the significance for a one-tailed test, the displayed significance is divided by two. Given the symmetrical distribution of the t statistic, the ‘significant’ tails in a two-tailed test, where a .05 criterion signifies a .025 significance in each tail, will be equivalent. As we are only interested in one of these tails, the significance is halved to determine the statistical significance of the t statistic. However, the differences in the distribution of age and BMI data between groups were assessed using the unpaired t test (2 tailed). A p value < .050 was considered statistically significant. The standardized mean differences (Cohen’s d) were also calculated given to the relative smaller sample size. Cohen’s d was interpreted as: similar (d < 0.20), small (0.20 ≤ d < 0.50), moderate (0.50 ≤ d < 0.80), large (d ≥ 0.80) (Cahan & Gamliel, Reference Cahan and Gamliel2011).
Results
Sociodemographic Characteristics
The sociodemographic characteristics of the study population are summarized in Table 1. Male twins constituted 25% of the total study population, while the remaining participants were OS female (25.0%) and SS female (75%) twins. The majority of the study population belonged to the Mole-Dagomba cultural group (65%), and the majority identified as Muslim (57.5%).
Table 1. The socio-demographic characteristics of the study population

Note: The results are summarized as frequency and percentages.
Male and Female Twin Variabilities
Hematological and biochemical variables between male and female twins were compared (Table 2). Male twins exhibited significantly higher levels of red blood cell (RBC) count (p = .031), hemoglobin (p < .001), serum creatinine (p = .009), gamma-glutamyl transferase (p = .001), total protein (p = .005), globulins (p = .002), and total testosterone (p < .001). However, fasting total cholesterol (p = .025) and low-density lipoprotein (LDL) (p = .013) were significantly higher in female twins. The significant findings had medium (0.50 ≤ d ≤ 0.80) to large (d > 0.80) effect sizes.
Table 2. Comparison of hematological and biochemical variables between male and female twins

Note: The results are summarized as mean ± SD. The differences between the groups were determined by unpaired t test (2-tailed) for anthropometric variables and unpaired t test (1-tailed) for hematological and biochemical variables. OS: opposite-sex, SS: same-sex; RBC, red blood cell count; HGB, hemoglobin; WBC, white blood cell count; LYMP, lymphocytes; PLT, platelets; CRT, creatinine; AST, aspartate aminotransferase; ALT, alanine aminotransferase; ALP, alkaline phosphate; CGT, gamma-glutamyl transferase; TBiL, serum total bilirubin; DBiL, direct bilirubin; IBiL, indirect bilirubin; TCHOL, total cholesterol; HDL, high-density lipoprotein; LDL, low-density lipoprotein; VLDL, very low density lipoprotein; TRIG, triglycerides; TT, total testosterone.
Cohens d: similar (d < 0.20), *small (0.20 ≤ d < 0.50), **medium (0.50 ≤ d ≤ 0.80) and ***large (d > 0.80).
Same-Sex and Opposite-Sex Female Twin Variabilities
Variabilities in hematological and biochemical variables between OS and SS female twins were compared (Table 3). Serum total bilirubin (TBiL) (p = .006), indirect bilirubin (IBil) (p = .005), fasting LDL (p = .008), and circulating total testosterone (p = .007) were significantly higher in SS than OS female twins. However, serum alanine transaminase (ALT) and albumin levels were significantly higher in OS than SS female twins.
Table 3. Comparison of hematological and biochemical variables between same- and opposite-sex female twins
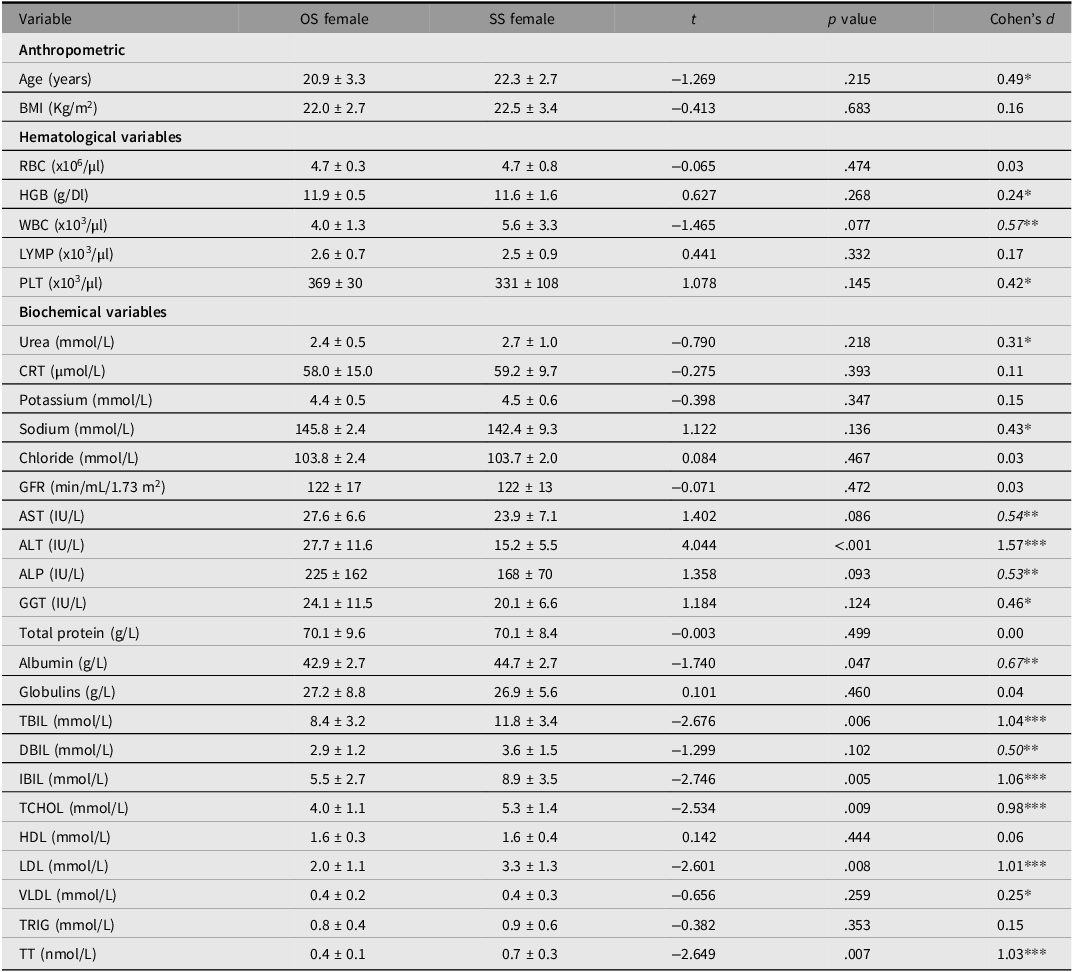
Note: The results are summarized as mean ± SD. The differences between the groups were determined by unpaired t test (2-tailed) for anthropometric variables and unpaired t test (1-tailed) for hematological and biochemical variables. OS: opposite-sex, SS: same-sex; RBC, red blood cell count; HGB, hemoglobin; WBC, white blood cell count; LYMP, lymphocytes; PLT, platelets; CRT, creatinine; AST, aspartate aminotransferase; ALT, alanine aminotransferase; ALP, alkaline phosphate; CGT, gamma-glutamyl transferase; TBiL, serum total bilirubin; DBiL, direct bilirubin; IBiL, indirect bilirubin; TCHOL, total cholesterol; HDL, high-density lipoprotein; LDL, low-density lipoprotein; VLDL, very low density lipoprotein; TRIG, triglycerides; TT, total testosterone.
Cohens d: similar (d < 0.20), *small (0.20 ≤ d < 0.50), **medium (0.50 ≤ d ≤ 0.80) and ***large (d > 0.80).
Discussion
The study sought to determine variabilities in hematological and biochemical variables between OS and SS females. The levels of total testosterone, hemoglobin, serum creatinine, total protein and gamma-glutamyl transferase were significantly higher in males than females. However, these variables were not significantly higher in OS than SS females as was expected.
The higher levels of serum creatinine, gamma-glutamyl transferase and hemoglobin and lower fasting lipids in men than women is consistent with previous studies. These sex-dependent variabilities are attributable to the differential activities of testosterone and estrogen on erythropoiesis, kidney and liver physiology as well as lipid homeostasis (Kasarinaite et al., Reference Kasarinaite, Sinton, Saunders and Hay2023; Murphy, Reference Murphy2014; Palmisano et al., Reference Palmisano, Zhu, Eckel and Stafford2018). The circulating testosterone in average adult men is about 2−3 times higher than similarly aged premenopausal women. Testosterone is synthesized from cholesterol, primarily in the Leydig cells of the testes in men and the ovaries in women, while estrogen is synthesized in various tissues, including the ovaries in women (Alemany, Reference Alemany2022). Sex-dependent variabilities are not apparent before puberty but become more pronounced during adolescence, where there is a surge in testosterone in males and estrogen in females. After puberty, sex hormone levels begin to decline, with corresponding effects on physiological processes (Palmisano et al., Reference Palmisano, Zhu, Eckel and Stafford2018).
It was expected that androgen-dependent hematological and biochemical variables would be higher in OS females than in SS females. However, this expectation was not met in the current study. Instead, fasting total cholesterol and LDL levels were higher in the SS females. This, however, aligns with the finding that total testosterone was also relatively higher in the latter than in the former.
The test of the TTT hypothesis is inconclusive. Previous studies have relied on phenotypic traits as the measure of prenatal testosterone exposure, as the direct measurement is problematic due to the occurrence of the effect primarily in the first trimester of pregnancy (Zheng & Cohn, Reference Zheng and Cohn2011).
The second-to-fourth (2D:4D) digit ratio is considered the putative marker of prenatal testosterone exposure; however, other cognitive, behavioral and pathologies may also serve as alternatives (Manning et al., Reference Manning, Scutt, Wilson and Lewis-Jones1998; Zheng & Cohn, Reference Zheng and Cohn2011). The 2D:4D ratio is negatively correlated with prenatal testosterone exposure. While some previous twin studies provided evidence for the TTT hypothesis, subsequent validation studies in larger twin populations found no support (Cohen-Bendahan et al., Reference Cohen-Bendahan, Buitelaar, Van Goozen and Cohen-Kettenis2004; Medland et al., Reference Medland, Loehlin and Martin2008; van Anders & Hampson, Reference van Anders and Hampson2005; Voracek & Dressler, Reference Voracek and Dressler2007). Several explanations have been offered for the lack of evidence for the TTT. First, much of the evidence has been derived from animal studies, and some authorities argue that findings from these studies cannot be directly extrapolated to humans. Moreover, strong evidence of TTT has been observed only in female litters of nonhuman mammals gestating between two male litters (Ahrenfeldt et al., Reference Ahrenfeldt, Skytthe, Möller, Czene, Adami, Mucci, Kaprio, Petersen, Christensen and Lindahl-Jacobsen2015). Second, amniotic testosterone levels may peak at a time when the effect of prenatal testosterone on the developing brain has already passed. Lastly, amniotic fluid testosterone levels may be too low to exert an effect on the developing fetal brain, as OS twins show no genital virilization when compared to individuals with congenital adrenal hyperplasia (Medland et al., Reference Medland, Loehlin and Martin2008).
The current study possesses several strengths. First, it is likely the first of its kind to test the TTT hypothesis in an indigenous Black African twin population, specifically examining hematological and biochemical variables. Second, the inclusion of OS males in the study aimed to ascertain sex-dependent variabilities in these variables, providing evidence for the potential role of testosterone in the observed differences. Lastly, to avoid assumptions from previous studies, current testosterone levels were estimated, ensuring a more accurate representation of hormonal profiles. However, the study faced limitations, notably a relatively smaller sample size compared to other studies (Medland et al., Reference Medland, Loehlin and Martin2008). This limitation arose from the challenge of gathering adult twins, many of whom are separated due to educational or work commitments. In addition, determining the zygosity status of the SS females was not possible due to logistical challenges.
Conclusion
The findings did not lend support to the TTT hypothesis, as OS females did not display male-typical patterns in hematological and biochemical variables. It appears that the TTT effect may either not occur in OS females or may not be potent enough to significantly influence these variables.
Acknowledgments
The authors extend their heartfelt gratitude to all participants and sincerely acknowledge the invaluable support provided by the staff of the Department of Biomedical Laboratory Science at the University for Development Studies.
Financial supports
This research received no specific grant from any funding agency, commercial or not-for-profit sectors.
Competing of interests
None.
Ethical standards
The study adhered to the guidelines outlined in the 1964 Declaration of Helsinki and its subsequent amendments. Approval was obtained from the Institutional Review Board of the University for Development Studies. Before sampling, written informed consent was secured from all participants, and voluntary participation with the option to opt-out at any stage was emphasized. Participation was not limited by religious, cultural, or political affiliations.