Introduction
Noroviruses (NoVs) are recognised as a leading cause of sporadic cases and outbreaks of acute gastroenteritis (AGE) across all age groups [Reference Patel1, Reference Matthews2]. They are transmitted primarily through the faecal-oral route either by direct person–person spread and with consumption of contaminated food or water [Reference Patel1, Reference Green, Knipe and Howley3, Reference Glass, Parashar and Estes4]. NoVs are non-enveloped RNA viruses belonging to the Caliciviridae family and are classified into seven distinct genogroups (GI-GVII) on the basis of the major capsid protein VP1 sequence. GI, GII and GIV NoVs infect humans and they have been divided into more than 30 genotypes based on the sequences of polymerase (ORF1) and capsid proteins (ORF2) [Reference Vinjé5]. NoV GII.4 genotype has been associated with the vast majority of NoV related cases of AGE worldwide although many genotypes, belonging to both GI and GII genogroups are involved in foodborne and waterborne outbreaks [Reference Siebenga6, Reference Hewitt7]. NoVs are highly contagious owing to their high stability in the environment and their ability to infect at low doses. In fact, just 10–100 virions of NoV may be sufficient to cause AGE [Reference Lindesmith8, 9]. NoVs are considered the major cause of water-related disease in semi-closed institutions, including restaurants, schools, hospitals, nursing homes and cruise ships [Reference Maunula, Miettinen and von Bonsdorff10–Reference Moreira and Bondelind12]. To confirm NoV as a cause of outbreaks, the presence of four epidemiological features of disease, vomiting in more than half of affected persons, a mean incubation period of 24/48 h, a mean duration of illness of 12–60 h and the absence of bacterial pathogens in stool culture, should be evaluated [Reference Kaplan13]. Waterborne NoV outbreaks can be caused by contaminated drinking water despite correct chlorine concentration and in the absence of coliform bacteria [Reference Maunula, Miettinen and von Bonsdorff10, Reference Nygard14–Reference Zhou17]. The aim of this study was to investigate an outbreak of NoV gastroenteritis associated with drinking water involving a high school class travelling from Palermo (West Sicily) to Acireale (East Sicily) on an educational tour in May 2016. Stool samples were collected soon after the onset of AGE symptoms to ascertain the aetiology of illness. The source of infection was investigated collecting epidemiological data through an online questionnaire and, in order to explore a possible transmission route, laboratory tests on water from the municipal network were performed.
Material and methods
Epidemiological investigation
From 20 to 22 May 2016 an AGE outbreak occurred in Mascali, a small town near Taormina, involving a school class of 28 students, 13 girls and 15 boys aged between 14 and 18 years and a 54-year-old female teacher, during a trip to participate in a theatre festival in Acireale. A total of 25/29 persons were affected by nausea, vomiting, abdominal cramps, diarrhoea and fever while they were staying at a seaside resort in Mascali, on the way to Acireale. To obtain data on symptoms and the correlated risk factors all participants filled an online questionnaire investigating personal data (age and sex), travel and accommodation details and food consumption over 48 h before symptoms presentation, time of onset and duration of symptoms, therapy and/or hospitalisation required and history of contact with people affected by diarrhoea and/or vomiting (Table 1). The severity of illness was analysed by the Vesikari severity scale allowing to distinguish non-severe (score <11) and severe (score ⩾11) AGE (Table 1). Vesikari score was calculated from maximum number of episodes/day and duration of both diarrhoea and vomiting (score 0–3 for each of the 4 parameters), temperature (score 0–3), level of dehydration (score 0–3) and treatment required (score 0–2) [Reference Givon-Lavi, Greenberg and Dagan18]. A case-control study was conducted to establish if food consumed before the onset of symptoms was associated with the disease. A case was defined as a student attending the tour and reporting diarrhoea and/or vomiting within 36 h from the first shared meal during the tour, while students who did not present any of the previous symptoms or those showing the first symptoms with a delay of ⩾48 h (secondary cases) were used as controls. Variables were studied using odds ratio (OR) and its 95% CI and statistical associations were determined using the χ 2 test, with the level of statistical significance set at 0.05 (Table 2).
Table 1. Clinical features, severity evaluation according to the scoring scale of Vesikari [Reference Givon-Lavi, Greenberg and Dagan18] and laboratory testing results of 25 subjects involved in the AGE outbreak that occurred in Mascali in May 2016

VS, Vesikari score; IVR, intravenous rehydration; ORT, oral rehydration therapy; HOS, hospitalisation.
a Calculated based on the previous columns of clinical features (Maximum score: 20).
Table 2. Analysis of food consumed in the shared lunch on Day 1 (19 May 2016) at the restaurant of the resort in Mascali
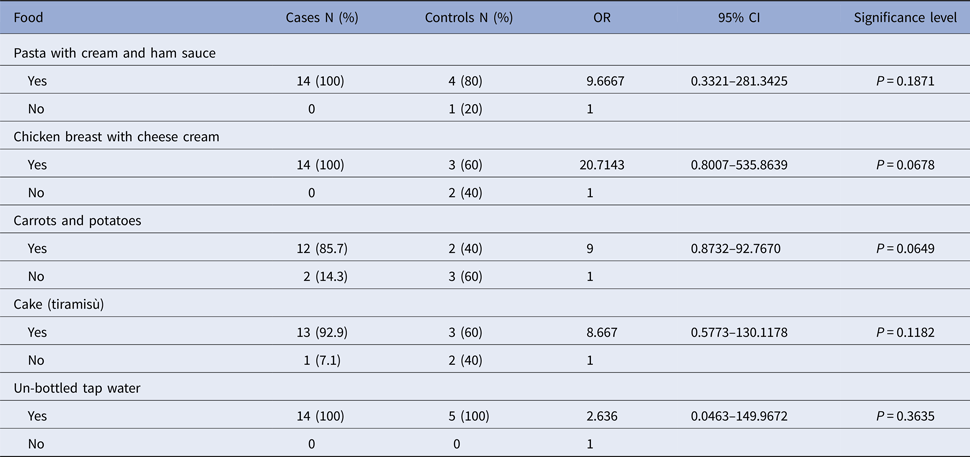
A total of 19 subjects filled the section of the online survey concerning food consumption. Fourteen subjects falling ill within 36 h from the first shared meal (first wave) were considered as cases. Three putative secondary cases, showing the first symptoms with a delay ⩾48 h (second wave), were used as controls together with two asymptomatic subjects.
OR, odds ratio; CI, confidence interval.
NoV detection in stool samples
A stool sample was obtained from each of the 29 travellers, soon after (3–5 days) the onset of the outbreak. No stool samples could be obtained from the food-handlers working at the resort. All samples were tested for NoV at the Enteric Viruses Laboratory of the University of Palermo, member of the Italian Study Group for Enteric Viruses (ISGEV), by a Real-Time polymerase chain reaction (RT-PCR) assay that allows to differentiate GI and GII NoVs [Reference Kageyama19]. Total RNA was extracted from stool samples using QIAamp Viral RNA Mini Kit (Qiagen, Hilden, Germany) and cDNA was generated by MMLV reverse transcriptase (Invitrogen, Carlsbad, CA). Samples were considered positive for NoVs when cycle threshold (ct) of RT-PCR was ⩽30 and low-positive when 30<ct⩽35. For all NoV-positive samples two genomic fragments were amplified by conventional RT-PCR using Superscript III One-Step RT-PCR system with Platinum Taq DNA polymerase (Invitrogen) and primers JV12a/JV13b, targeting 326 bps of the RdRp region A [Reference Vinje and Koopmans20] and either COG1F/GISKR or COG2F/GIISKR, amplifying a 315 bps 5′portion of GI or GII VP1 protein [Reference Kageyama19, Reference Kojima21].
Environmental investigation
From May through August 2016, a total of 32 tap water samples were collected from the Mascali municipal network. In particular, seven samples were collected at the resort where the outbreak occurred and from its water entry (R), two in the city centre of Mascali (M), 15 in the village of Fondachello (F), on the seaside, three in Carrabba village (C), far from the coast, and two and three, respectively, from the water well ‘Carlino’ (W) and the source ‘Bufardo and Torregrossa’ (S) which feed the municipal system and provide drinking water to the Mascali area (Fig. 1, Table 3). One litre water samples were sent by the local health authorities to the SIALAB laboratory in Avola, Siracusa and 100 ml aliquots were screened for coliforms, E. coli and enterococci as faecal indicators, by standard bacteriological methods according to UNI EN ISO 9308-1:2014.

Fig. 1. Location of sampling sites along the municipal water network and results of bacteriological and NoV
screening: N° samples positive / N° samples tested. The red sections of the network indicate uncertain route, most probably subjected to leakage. The urban area was divided into sections: R, Resort; F, Fondachello; C. Carrabba; M, Mascali city centre; W, Carlino well; S, Bufardo and Torregrossa source.
chlorination device.
Table 3. Water sampling and results of Coliforms, E. coli, Enterococci and NoV detection

Samples from the resort area (R) are highlighted in bold.
Unfortunately, although the suspect of a water source for the viral outbreak was quickly communicated, only in August and November 2016, following the implementation of control measures, local authorities provided water samplings of appropriate volume to perform viral tests (10 l). Five water samples of 10 l each were collected from different sampling sites in F and R areas for viral screening (Table 3) and were concentrated according to the Italian National Institute of Health (Istituto Superiore di Sanità, ISS) protocols [Reference Istituto Superiore, Lucia and Massimo22] through an ultra-filtration system (Sartoflow® Slice 200 Benchtop Crossflow System, Sartorius AG, Goettingen, Germany), using appropriate membranes (SG Hydrosart 10 kDa) pretreated with 300 ml of 3% Beef Extract (BE) at pH 7, by recirculating it for 10 min in the system, at a pressure not higher than 1.30 bar. Elution and recovery of viruses attached to the membranes were obtained by recirculating 200 ml of 3% BE at pH 9.5 for 3 h until a 10–12 ml volume of eluate was obtained. The pH of the eluate was then checked with litmus paper and if necessary brought to the value of 7 with HCl. Concentrated water samples were simultaneously tested for NoV in three laboratories: Enteric Viruses Laboratory, University of Palermo; Istituto Zooprofilattico Sperimentale della Sicilia (IZS), Palermo; and Istituto Superiore di Sanità (ISS), Rome. Total RNA was extracted from 500 and 560 µl of a concentrated water sample using BioMèrieux NucliSENS® Magnetic Extraction System (BioMèrieux) and QIAamp Viral RNA Mini Kit (Qiagen, Hilden, Germany) respectively, according to manufacturer's instructions. Bonded RNA was eluted by 100 µl of Elution Buffer. Amplifications were performed at least in duplicate on whole and 1:10 diluted extracts. A Real-Time RT-PCR assay according to ISO/TS 15216-2:2013, two in-house Real-Time RT-PCR protocols and the One-Step RT-PCR methods already used for amplifying ORF1 and ORF2 fragments in NoV-positive faecal samples were performed [Reference Kageyama19–Reference Kojima21, Reference da Silva23].
Nucleotide sequences analysis and phylogenetic analysis
PCR amplicons were purified by commercial columns (CleanSweep PCR Purification, Thermo Fisher Scientific, Vilnius, Lithuania) and sequenced by Sanger method and all nucleotide sequences were aligned using ClustalW. To identify NoV genotypes, RdRp and VP1 sequences were submitted to the RIVM NoV genotyping tool (http://www.rivm.nl/mpf/norovirus/typingtool). Phylogenetic trees were constructed using MEGA 7 and Neighbour-Joining method (bootstrap 1000 replicates).
Sequences from stool and water samples are available at NCBI with the following accession numbers: MG517455 for the major capsid protein obtained from a water sample; MG517456 and MG517461, respectively, for the major capsid protein and RNA-dependent-RNA-polymerase, as representatives of the sequences obtained from the stool samples of those involved in the outbreak.
Control measures
After outbreak notification, on 21 May, the resort was closed for 2 days. On 25 May, following the first results of the bacteriological analyses on municipal water samples, the use of municipal water for human consumption was temporarily restricted (use of water was permitted only for cleaning purposes) in the Carrabba and Fondachello villages and in the area surrounding the resort. From 25 May the reservoir collecting the water from the Carlino well and its chlorination device were submitted to maintenance work and a new chlorination device was installed on the conduit bringing the water from the Bufardo source. Actually, the Bufardo source had been connected to the municipal network in 2003 but unfortunately a dedicated chlorination station had not been planned at that time. Therefore, unchlorinated water entered the system near Fondachello village on the seaside (Fig. 1), possibly diluting chlorine concentrations in water treated at the Carlino reservoir. Moreover, the exact path of the branch of the water network situated downstream the Bufardo source water connection was not marked in the maps of the municipality (Fig. 1, red line), indicating that this section had never been submitted to maintenance. The area surrounding the unmaintained section of the water pipeline was scattered with unauthorised cottages that were not connected to the sewerage systems. Likely, untreated waste from cesspools was unloaded directly into the soil, generating a risk of contamination of the municipal water system through leakage. No work had been made on the water pipelines before the outbreak but sections of the network had been temporarily closed during the winter season on the seaside (Fondachello). Local health authorities advised the connection of all households in the area to the sewerage system and the replacement of the water system section without maintenance.
Health authorities also raised attention on AGE cases notification at all local Emergency Departments and Family Practitioners but there was no further evidence of AGE outbreaks in the months following the intervention.
Results
Data collected through the online questionnaire compiled 7–10 days after the outbreak revealed that none of the 28 students and the teacher taking part in the school trip showed gastroenteritis symptoms at the beginning of the journey and they had no contact with people who suffered from diarrhoea and/or vomiting in the previous days. Travellers had their first shared meal on 19 May (Day 1), 2016, at lunch on their arrival to the resort in Mascali. The list of foods included in the menu is shown in Table 2. Conversely, the dinner on Day 1 was not shared and the students went to dine in three different fast food restaurants in Acireale. On 20 May (Day 2), 17 students (58.6% of the travellers) presented with vomit and/or diarrhoea, falling ill 26–36 h after lunch on Day 1, strongly suggesting that exposure occurred on the first shared meal. Some students also vomited in the presence of the other travellers on the bus bringing them to Acireale. Five more students were symptomatic in the early hours of the following day (21 May, Day 3, from 0:30 am to 8:00 am), as a continuation of the wave of illnesses of the previous day. The teacher and one more student fell ill at 8:00 pm and 11:00 pm of Day 3, respectively, for a second wave of illnesses occurring >48 h from the first shared meal and <36 h from the first cases, with the last student falling sick on 22 May (Day 4) at 3:00 am (Fig. 2, Table 1). On the whole, the mean incubation period was 33.2 h. Based on the online survey, the severity of illness was evaluated by the Vesikari severity scale, grouping symptomatic travellers into severe (11/25 = 44%) and non-severe (14/25 = 56%) cases (Table 1). Vomiting and fever were the predominant symptoms, affecting 22 out of 25 (88%) symptomatic subjects, while diarrhoea was present in 14 out of 25 (56%). Hospitalisation for rehydration therapy was necessary for 13 students (91% [10/11] of severe and 21% [3/14] of non-severe cases). Emergency room (ER) or family practitioner visits were necessary for 21 students and 13 (62%) of them initiated an antibiotic therapy. Two students indicated that other family members had gastroenteric symptoms more than 24 h after their return home, on Day 3, 4 and 5. All those involved in the outbreak recovered within 4 days, with an average duration of illness of 38.4 h. A case-control study of the risk associated with eating foods at lunch on Day 1 or with attending different restaurants at dinner on Day 1 was attempted. A total of 19 subjects filled the section of the online survey concerning food consumption. Fourteen of the responders were among the 22 cases falling ill within 36 h from the first shared meal (first wave), while the remaining five responders were three travellers with supposed secondary infections (showing symptoms with a delay ⩾48 h; second wave) and two asymptomatic students that were used as controls. No statistically significant associations with any food items served at lunch on Day 1 was identified (P < 0.05; Table 2). However, all food consumed on that occasion was to some extent associated with the risk of disease, the highest OR being obtained with chicken (OR: 20.7143, P: 0.0678; Table 2). Actually, most of the cases ate all foods included in the menu, while one of the two asymptomatic controls did not participate in the meal and the other had a pasta dish only. No statistically significant association was found for attending a particular fast food restaurant at the dinner on Day 1 (OR: 2.5, P: 0.3635).

Fig. 2. Timeline of the NoV disease outbreak in Mascali.
The Real-Time RT-PCR assay allowed to detect GII genogroup NoV genome in all the 25 samples from symptomatic subjects, although two of them showed high Ct values, indicating lower shedding of viral genomes (Table 1). The samples from four asymptomatic students were negative for both NoV genogroups. By sequence analysis, a NoV genotype could be defined for 20/25 NoV-positive stool samples. All NoV strains from travellers involved in the outbreaks belonged to the same genotype, GII.P2/GII.2, showing >99% nucleotide identities (nt id) in both fragments (ORF1 and ORF2) analysed.
Thirty-two water samples were collected and analysed for bacterial contamination (Table 3). Seven samples were taken from the resort (site R) and the remaining 25 from five other sites (C, F, M, S, W), representative of the entire municipal water network. The majority (10/12) of the water samples collected immediately after the onset of the outbreak, between 21 May and 16 June 2016, at the resort (site R) and at sites F and C, located close to the resort, on the seaside and in the interior, respectively, were positive for coliform bacteria. More than 1000 colony-forming units (CFU)/100 ml (up to 2700 CFU/100 ml) were detected in four samples, while their absence is required in 100 ml by the Standard of Water Quality in Italy and seven of 11 samples tested were also Escherichia coli-positive (up to 900 CFU/100 ml). Enterococci were searched in 11 samples and three of them were found positive for this faecal contamination indicator. From 7 July to 16 August 2016, after control measures on the water network were undertaken, 13 water samples were collected for follow-up laboratory examination of the water system at F, R and C sites. All of them were negative for bacterial indicators of faecal contamination. No faecal bacteria were identified in the seven samples collected from M, W and S sites, including the well and the source that fed the water network, at any sampling time, before and after implementing the control measures. The research of NoV genome was conducted on concentrated water samples collected on 1 August from R and F sites (Table 3). NoV genome was detected in a single sample collected from the water entry of the resort involved in the outbreak (site R). Actually, only two out of 26 One-Step RT-PCR repetitions were positive for NoV viral capsid gene. The two ORF2-positive results were obtained at the University of Palermo Laboratory using both 1:10 diluted and undiluted extracted RNA. The sequence analysis of the partial ORF2 portion (289 bp) indicated the presence of the same GII.2 capsid genotype detected in the stool samples of the students involved in the outbreak, showing 98.9% nt id. No ORF1 NoV sequence could be obtained from the NoV-positive water sample. Follow-up analyses for NoV genome, conducted on water samples collected on 20 November both at R and F sites found no positive samples.
By sequence alignment, the NoV sequences obtained from Mascali outbreak samples were phylogenetically closely related in both ORF1 and ORF2 (99 and 98.9% of nt id, respectively) to a GII.P2/GII.2 strain responsible for a sporadic paediatric gastroenteritis case occurred in Palermo in January 2016 (PA 36/16, accession numbers MG517464 and MG517460, for ORF1 and ORF2, respectively) and previously detected at the Enteric Viruses Laboratory, University of Palermo. Whereas, three GII.P2/GII.2 Italian strains (PA 280/11, PA 366/11 and PA 3/14, accession numbers MG517462, MG517465 and MG517463, for ORF1 and MG517457 to −59, for ORF2, respectively), detected in Palermo in 2011 and 2014, were more distantly associated with the outbreak strain both in RdRp and VP1 (90% to 97.4% nt id and 96.1% to 98.1% nt id, respectively, in ORF1 and ORF2).
Discussion
Epidemiological features, such as the presence of vomiting in more than half (88%) of symptomatic subjects, the 33.2 h average incubation period and the 38.4 h average duration of illness, suggested a NoV aetiology for the investigated outbreak [Reference Kaplan13]. The detection of NoV genome in all 25 faecal samples from symptomatic subjects fully complies with the requirement of detection of at least one positive sample out of four tested that is considered to be sufficient to ascertain the aetiology of a NoV outbreak [Reference Duizer24]. Moreover, molecular typing indicated that a single viral strain, belonging to genotype GII.P2/GII.2, infected all symptomatic subjects involved in the outbreak.
NoVs are highly infectious and can easily spread from person to person but also through contaminated food or water. Therefore, settings such as health care institutions, schools and touristic resorts are mainly affected, since close contact and shared food services facilitate transmission. In Mascali, two waves of transmission were observed over three days, being consistent with food/waterborne followed by person-to-person and/or airborne (vomit) transmission (Fig. 2). The first wave of cases presented on 20 May 2016 after a single shared meal, while the last case was detected on 22 May. Exposure to vomit may have been responsible for some of the secondary cases occurred after the first wave of illness. Vomiting produces aerosols that can be inhaled and swallowed. The extremely low infectious dose of NoV makes the inhalation of vomit aerosols an effective transmission route in settings where there is close contact, like on a bus trip [Reference Solano25].
All food consumed in the only shared lunch on 19 May at the resort was associated with the risk of disease (Table 2), but the association was inconclusive since all cases shared the same dishes included in the lunch menu on Day 1. Although many foods were potentially involved, our attention focused on the tap water. In fact, trivial information from local health authorities reported that local population avoided tap water consumption and relied on bottled water for drinking and cooking. Many small outbreaks of gastroenteritis involving restaurants and family groups had been reported in the same area, especially at the beginning of the summer season, when vacation houses and recreational activities were re-opened after a period of low water demand. Illness caused by NoV is common and if the level of water contamination is low, the number of cases remains limited and water can persist as unrecognised source of infection. If polluted water was served for drinking and used for cooking and washing dishes in the Mascali resort at Day 1 a generalised contamination of food and surfaces could have occurred. The potential role of the food-handlers as a source of contamination could not be analysed in this study but should also be taken into account. If water contamination has already been present in the area for a long time, infections may have occurred among the local food-handlers. The analysis of water samples taken immediately after the outbreak revealed the presence of bacterial indicators of faecal contamination in the water used in the resort, with the only exception of a sample of filter purified water used both for cooking and as drinking water. When extending the sampling to other areas of the municipal water network, many other sites, mostly on the seaside, showed faecal pollution, which demonstrates a poor protection of the water network from faecal leakage (Fig. 1). NoV contamination of waters generally originates from sewage since these enteric pathogens, as other enteric viruses affecting humans, are mostly species-specific and are shed at high concentrations in the stools of patients. When the analyses on the stools of the students made it clear that the outbreak had been caused by NoV, the viral genome was also searched in appropriate volumes of water samples, but this happened when correction measures on the water network had already been taken. Nevertheless, a single water sample, obtained 2 months after the outbreak (1 August 2016), still contained genome fragments that could be correlated to the same NoV genotype causing the outbreak. Although the finding of NoV genome does not demonstrate that entire infecting viral particles were present in the water, it could indicate that a NoV belonging to the same genotype involved in the outbreak was still circulating in the local population several weeks after the outbreak and it was someway able to penetrate the water network, probably indicating continuing faecal contamination through leakage from sewage systems. NoV is particularly resistant in the environment and also to chlorine, therefore transmission through chlorinated water is not exceptional [9, Reference Moreira and Bondelind12, Reference Kauppinen and Miettinen26]. Drinking water from municipal supplies fed by lake or groundwater has already been identified in the past as the source of waterborne NoV outbreaks in Italy [Reference Boccia27–Reference Giammanco29] and NoV is now considered one of the pathogens causing the largest number of affected consumers in drinking waterborne outbreaks [Reference Moreira and Bondelind12]. NoV can persist in water longer than 15 days [Reference Seitz30] and it is resistant to chlorine disinfection when free chlorine levels or pre-treatment processes are inadequate [Reference Kingsley31, Reference Shin and Sobsey32]. Moreover, microfiltration filters labelled as certified by NSF Standards 53 or 58 (with a pore size ranging from 0.05 to 5 µm) are not effective in removing viruses and can even decrease chlorine concentration in filtered water [33]. Actually, the owner of the resort involved in our outbreak was encouraged to serve tap water as drinking water at the restaurant relying on the effectiveness of such a filter purifier. Unfortunately, we did not have a tap water sample from the restaurant of the resort taken before correction measures and whose volume was appropriate for virus detection. Therefore, we could not demonstrate the failure to eliminate viral pollution of the microfiltration system. In Mascali, correction measures on the water network consisted in the maintenance of the old chlorination device and in the addition of a new one at a key point located upstream and close to suspected illegal sewage dumping (Fig. 1), but as poor protection of the pipeline from sewage leakage risked to reproduce faecal pollution episodes, renovation of the municipal water system was also advised, as well as systematic control of illegal sewage dumping. The additional chlorination device was apparently the most effective intervention, since right after its activation faecal bacterial pollution indicators disappeared. However, NoV genome was detected in a water sample taken 6 weeks after the last detection of bacterial indicators. Unfortunately, detection of faecal contamination of drinking water is only based on the use of bacterial indicators despite no correlation between levels of enteric bacteria and enteric viruses has been found [Reference Gerba34–Reference Lucena36].
According to ISGEV enteric virus circulation surveillance data, in 2016 the circulation of GII.P2/GII.2 NoV strains in Palermo, home town of the travellers involved in the outbreak, was the highest ever observed from the starting of local NoV surveillance in 2004. GII.P2/GII.2 was the fourth most common genotype in 2016 (12.2% prevalence), following GII.P4/GII.4 Sydney 2012 (28.4%), GII.P16/GII.2 (18.9%) and GII.Pe/GII.4 Sydney 2012 (17.6%). GII.P2/GII.2 strains were mostly observed starting from July 2016, leaving space for the possibility that their increased circulation was linked to the introduction of the ‘Mascali clone’. However, a couple of GII.P2/GII.2 strains (including PA36/16) genetically close to the ‘Mascali clone’ had been already detected in January 2016 in Palermo and GII.P2/GII.2 NoV had sporadically been observed also in 2011 and 2014. Moreover, the circulation of GII.P2/GII.2 NoVs has been increasing worldwide over the last period [Reference Cannon37]. Therefore, the unusually high circulation observed in Palermo might just reflect the global epidemiology of GII.P2/GII.2 strains. Noteworthy, the older GII.P2/GII.2 NoV strains detected in Palermo were closely related in the ORF2 (98.9% nt id) to the GII.P16/GII.2 Nashville strain (KY865307) which is supposed to have generated the recombinant GII.P16/GII.4 clone recently circulating epidemically worldwide [Reference Cannon37, Reference Ruis38] and were more distantly associated (95.3% nt id) to the GII.2 capsid sequence (AB662868) of another putative GII.P16/GII.4 ancestor from Japan [Reference Iritani39].
The increasing frequency of detection of waterborne NoV outbreaks calls for the introduction of routine monitoring for viruses of drinking waters and for the definition of affordable and reliable indicators for the presence of NoV in drinking waters. The use of ultrafiltration systems as a concentration method in order to recover viruses from environmental waters combined with RT-PCR to detect viral genomes has been demonstrated to grant a recovery rate of >50% of multiple viruses [Reference Olszewski40] and is at present one of the most sensitive approaches for the detection of NoVs, although the discrimination between viable and nonviable viruses remains a problem. The burden of NoV outbreaks, either food associated or waterborne, is still poorly defined due to the lack of specific diagnostic tools in many clinical laboratories. NoV epidemics should always be suspected when the main symptom is vomiting and the duration of disease is short (<48 h). Waterborne transmission is suggested by the rapid spread of disease and the high attack rate. Care should be taken to perform sensitive diagnostic tests on a sufficient number of stool samples in order to identify NoV as the causative agent of enteritis outbreaks, possibly preferring genome screening over antigen detection. Only the systematic detection of NoV outbreaks can guide in the choice of the safety procedures that could be most appropriate in containing such microbiological hazard.
Acknowledgements
The Enteric Viruses Laboratory of the University of Palermo participates to the Italian Study Group for Enteric Viruses (ISGEV; http://isgev.net). The authors would like to thank Dr Marco Romano for English language editing. This research received no specific grant from any funding agency, commercial or not-for-profit sectors.
Declaration of Interest
All authors declare no conflict of interest.
Ethical standards
The ethics committee approval was not required as this was a public health problem regulated by legislation on diseases under public health surveillance.