Aquaculture, the husbandry and farming of aquatic animals and plants, has expanded faster in recent decades than any other livestock sector. It achieved a 7·5 % annual growth rate between 1990 and 2009, eclipsing the rapid growth rates of the pig (<2·5 %) and poultry (<5 %) sectors( Reference Troell, Naylor and Metian 1 ). In comparison, the overexploitation of wild fishery stocks has led to their contribution to world food stocks flat-lining. Approximately 30 % are overfished, more than 60 % fully fished and <10 % have remaining capacity( 2 ). In response to expanding demand from growing and better off populations, the rise of aquaculture has been timely but its development has not been evenly distributed nor without criticism, especially regarding environmental and social impacts( Reference Troell, Naylor and Metian 1 , Reference Naylor, Hardy and Bureau 3 , 4 ). The characteristics of aquaculture, growing rapidly from an artisanal and marginal activity, unknown in most of the World, to a position where there are now major complementarities and, potentially, resource allocation conflicts with terrestrial livestock and conventional fisheries are reviewed in the present paper. Aquatic products, ‘fish’, often remain neglected in the current discourse regarding food security( Reference Béné, Arthur and Norbury 5 ) despite its importance in world trade, human nutrition and support for livelihoods more broadly. This theme is also explored in the paper.
Why farm? The continuance of wild fisheries in seafood supplies
‘The motivations for people in traditional societies to begin farming fish and shellfish are lost in time but an assessment of terrestrial farming may offer some clues’. The agricultural economist Ester Boserup( Reference Boserup 6 ) would answer that farming began because ‘it was necessary’ or as another observer noted ‘People did not invent agriculture and shout for joy. They drifted or were forced into it, protesting all the way’( Reference Tudge 7 ). It was also, likely, a gradual process. Certainly, the transition from hunting to farming of terrestrial food occurred over a longer time frame and geography than that for aquatic products. A process of proto-agriculture characterised by an opting in and out of plant and animal cultivation depending on need, and coaxing more food out of the environments depending on need was part of a broader repertoire of responses to times when demands for wild foods outstripped supplies. Beveridge and Little( Reference Beveridge, Little and Costa-Pierce 8 ) made a parallel case for the likelihood of such proto-aquaculture occurring in the same way it has for agriculture. It is clear that aquaculture began independently and at various times in different parts of the world and at several points along the aquatic food supply line, between water and plate. The farming of fish and shellfish is by definition an activity of settled societies, originating among both fishing and wetland farming cultures as well as at points of trade. It seems also to have not been exclusively about food provision; Beveridge and Little( Reference Beveridge, Little and Costa-Pierce 8 ) found that historically in some contexts culture and religion were often powerful motivations to invest in fish culture and the same has been demonstrated in some contemporary experience( Reference Harrison, Stewart and Stirrat 9 ).
Since the end of World War II growth of global fish (fish + shellfish) supplies have outstripped population growth, effectively increasing annual per capita supplies from 9·9 kg in the 1960s to 18·4 kg in 2009( 10 ). Growth has been fuelled by rising demand for livestock and fish, the result of increased economic access, changes in trade policies and market liberalisation, urbanisation and marketing( Reference Kearney 11 ). During the first half of the post-war period increases in fish supply came from capture fisheries, thanks to massive private and public investments that resulted in a proliferation of larger, more robust and increasingly mechanised fishing craft and more effective means of locating, catching and preserving fish until landed( Reference Beveridge, Thilsted and Phillips 12 ). By the late 1970s, however, the majority of fish stocks was fully or overexploited( Reference Arnason, Kelleher and Willmann 13 ). Today, capture fisheries is dominated in production and employment terms by small-scale artisanal tropical fisheries. While aquaculture accounted for only 3–6 % of global fish supplies in the 1970s in the subsequent decades it has consistently been among the fastest growing animal source food sectors, to the extent that one fish in two now consumed is farmed( 2 , 4 ). Any future expansion of supplies must come from aquaculture.
Fisheries currently sustain the livelihoods of more than 40 million full-time and part-time fishers directly, an estimated 90 % of whom are small-scale or artisanal and the balance in industrial sector. Furthermore, an estimated 120 million are supported through fisheries-related activities, through employment in value chains etc. In contrast more than one-third, or approximately 1 billion people, are employed in agriculture globally. In poorer counties, the proportion of employment is higher, reaching 60 % in sub-Saharan Africa( Reference Hishamunda, Bueno and Menezes 14 ).
The phrase ‘the livelihood of last resort’ has been coined for fisheries, and the concept of deteriorating fisheries being a poverty trap is supported by recent studies. Cinner et al.( Reference Cinner, Daw and McClanahan 15 ) identified the poorest fishers as the least likely to exit from a fishery in decline, although globally fishing is regarded as a traditional and noble occupation that may be passed down through generations. Amongst the rural poor, fishing activity may decline in favour of alternative income generating activities but not cease totally and fishers may be especially reluctant to cease if they have significant investment in fishing vessels and gear( Reference Cinner, Daw and McClanahan 15 ). The availability of alternative livelihood opportunities has been recognised as a key step and aquaculture has been identified as a possible option. However, this has given variable results, depending on how lucrative the diversification strategies are in comparison with fishing. Seaweed farming in the Philippines, for example, has produced mixed results( Reference Hill, Rowcliffe and Koldewey 16 ). Fishing may be preferred because of its ability to provide occasional very high returns in comparison with activities such as seaweed farming that are unproven in terms of providing long-term security. The viability of a small-scale seaweed culture operation is governed by many of the same challenges as other small-scale aquaculture initiatives, including the availability of quality seed( Reference Fei, Bao and Lu 17 ) and access to lucrative markets( Reference Lentisco and Needham 18 ). Seaweed farming can be particularly labour intensive and there are still significant technical hurdles for many species. Sheriff et al.( Reference Sheriff, Little and Tantikamton 19 ) found that the success of grouper and Asian sea bass cage farming by fishers in southern Thailand was dependent on a number of factors, including access to credit and the substitution of financial for natural capital. The factors that have led to the persistence of fisheries and varied development of aquaculture are now considered.
Modern emergence of aquaculture: an uneven picture
Opportunity rather than necessity has arguably been the major driver of modern aquaculture, which only has a history of four to five decades with major increases in the past two decades. Some key exceptions aside, the rapid growth in aquaculture has been linked as much to broader, the so-called ‘immanent’ development, than specific innovations( Reference Belton and Little 20 ). Increased human population, but more importantly increasing wealth and per capita consumption of fish, especially in wealthier Western countries, has driven incentives for aquaculture as an enterprise at a household and increasingly at the corporate level. Urbanisation has made self-sourcing impractical for most, fuelling the trade in fish as a commodity. Historically fish culture has often been a peri-urban phenomenon, driven by easy access to inputs and markets( Reference Bunting, Little, de Zeeuw and Drechsel 21 ). In contrast, rural populations in South and Southeast Asia traditionally secured aquatic products with little to no effort, as an output of wetland-based agroecosystems( Reference Gregory and Guttman 22 , Reference Amilhat, Lorenzen and Morales 23 ). Flooded rice-fields produce a large variety and significant volumes of aquatic animals as co-products and efforts to diversify away from rice monoculture typically resulted in deeper pond areas( Reference Little, Surintaraseree and Innes-Taylor 24 ). Smallholder production in such systems( Reference Amilhat, Lorenzen and Morales 23 ) has responded to increased demand and seasonal shortfalls, often evolving into commercially orientated but still largely household managed systems in Asia( Reference Belton, Ahmed and Murshed-e-Jahan 25 ). These include shrimp production that has grown strongly in the past three decades since hatchery juveniles have become available, in spite of disease-related setbacks. The sector, dominated by two species, Litopenaeus vanammei and Penaeus monodon, remains characterised by a large range of production systems and culture intensities. In contrast, the farming of Atlantic salmon (Salmo salar), another global industry, is characterised by its highly standardised cage-in-coastal water production system. Growing at an average annual rate of 16 % since 1985, Norweigan interests dominate globally, producing more than 50 % of the total harvest in-country( Reference Asche, Roll and Sandvold 26 ) and with significant interests elsewhere (Canada, Chile, Scotland). Developing international trade for such species has driven transformation further and in the case of salmon led to consolidation of production among fewer larger enterprises( Reference Asche, Roll and Sandvold 26 , Reference Shepherd and Little 27 ). Improved productivity of larger farms, increasing levels of specialisation of production and refined regulation have all contributed to consolidation, for which there is a general emergent trend across the seafood sector. Österblom et al.( Reference Österblom, Jouffray and Folke 28 ) identified thirteen lead firms in the sector, more than half of which were located in the Asia Pacific region, badging them as keystone actors because of their analogy to the disproportionate impact that keystone species in ecosystems exert on ‘ecosystems’. These keystone actors currently control 11–16 % of the global marine catch and 19–40 % of the most important stocks, including Alaskan pollock (Gadus chalcogrammus) and various tunas. The thirteen firms include the top two fishmeal and fish oil producers, 68 % of salmon and 35 % of shrimp feed producers, as two of the most reliant sectors on fishmeal and fish oil, and therefore exert an influence at both ends of the food chain.
Ensuring aquaculture is sustainable or at least responsible has become a key driver for OECD (Organisation for economic co-operation and development) economies, particularly in North America and Europe, and private sector governance where certification, offered by a range of organisations, has emerged as a major force. This contrasts with the major centre of production and consumption, the Asia Pacific region, for which national and intraregional trade remains largely outside such standards( Reference Bush, Belton and Hall 29 ). Production in farmed carps, catfish and tilapias has grown strongly in this region and probably constitutes more than 80 % of global fin-fish aquaculture (Fig. 1). Growth of major carps and tilapias is especially strong and is likely to dominate growth into the near future (Fig. 2). The sustainable intensification of such lower trophic species, especially in terms of the feed ingredients used in their diets, warrants at least as much attention as the critical focus on salmonids and shrimp to date and this is considered in a later section.
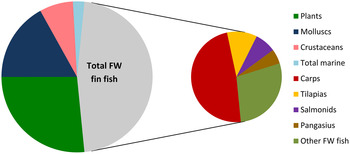
Fig. 1. Global aquaculture production by volume in 2014( 42 ).

Fig. 2. Growth of major aquaculture fin-fish species until 2014 and extrapolated projections to 2030 (dotted line), millions of tonnes( 42 ).
The rapid growth in aquaculture in some parts of the world suggests that technical barriers can be overcome given the right context for development and is often achieved largely by commercial actors. However, recent history suggests that a pioneer development phase can occur without significant levels of conventional research, as demonstrated by the rapid growth of the striped catfish (Pangasianodon hypophthalmus) sector in Vietnam between 2000 and 2008 that outpaced Norwegian salmon production, with only a fraction of the research and development investment (Fig. 3). The recent decline in striped catfish outputs reflects market constraints rather than significant technical challenges and the fish remains highly competitive in global white fish markets; investment in research at this point is likely to have impressive returns in terms of profitability. These examples, however, contrast with those where aquaculture, as either a subsistence or commercially orientated activity, has developed far more slowly or indeed has never become established, even when supported by targeted assistance. A long and disappointing history of promoting subsistence-orientated aquaculture in sub-Saharan Africa has been the subject of some analysis( Reference Brummett, Lazard and Moehl 30 , Reference Beveridge, Phillips, Dugan, Andrews-Couichan, Franz and Ravet 31 ) but aquaculture has generally failed to be sustained, even where fish has dietary importance, in contexts as varied as Sri Lanka to Caribbean and Pacific Islands( Reference Murray 32 , Reference Leschen and Little 33 ). Failure has been linked to a misunderstanding of demand and, often, a lack of any competitive advantage of start-up aquaculture enterprises with established fisheries. The global aggregate decline in importance of fisheries obscures important local differences. The European Union continues to rely massively on wild catches, of which a substantive proportion is imported (60 % of the overall seafood supply)( Reference Bostock, Lane and Hough 34 ). because of substitution of cheaper products from local fisheries and imported wild and cultured products.

Fig. 3. Norwegian salmon and Vietnamese catfish production, 2000–2014 along with cumulative numbers of peer reviewed articles for each to 2016( 42 ).
Generalised aquaculture statistics also lead to the wrong conclusions and disguise its real status. Just as the aquaculture sector in Europe, with some exceptions, has failed to grow, double digit growth characterises expansion in Asia. Drilling further down into the data, however, shows that Atlantic salmon production is now more important than beef in Scotland, at least as far as total farm revenues are concerned( 35 ). Mediterranean sea bass and bream production has hugely increased in Turkey, whilst a boom in aquaculture has failed to materialise in some countries in Asia, such as Malaysia, despite Government support and rapid expansion in neighbouring countries. More than 65 % of Indonesia's massive output are marine seaweeds, mainly supplying markets for hydrocolloids (caregeenan) widely used in food processing, although there has also been rapid growth of shrimp, milkfish, tilapia and various catfish (Fig. 4).

Fig. 4. Aquaculture production in selected Southeast Asian countries in 2004 and 2014( 42 ).
Furthermore, modern aquaculture development can be characterised as another green rather than a blue revolution, as most fish production occurs inland in freshwaters rather than the sea( Reference Edwards 36 ). The reality thus inevitably mutes the expectations of mariculture being a panacea for food security since a reliance on terrestrially derived feed ingredients remains, which are heavily constrained by land and fresh water availability( Reference Roberts, Newton and Bostock 37 ). The exceptions to this ‘norm’ are filter-feeding bivalves and seaweeds, for which expanded production is not linked to such constraints but which are still subject to market and site availability factors.
Thus, the trends in seafood production are more complex than often presented, as are the challenges to aquaculture becoming a major source of food and nutrition where it is most required. An examination of the geography of nutritional reliance on seafood can inform our understanding of its spatial development, to which we now turn.
The nutritional imperative
Seafood constitutes nearly 16 % of all global animal protein, more than 5 % of all protein and an estimated 4·5 billion people rely on seafood for 15 % or more of their animal protein( Reference Béné, Arthur and Norbury 5 ). A conventional focus on protein in diets has undervalued the key importance of fats, especially the highly unsaturated fatty acids, and micronutrients of which seafood are concentrated sources( Reference Domingo, Bocio and Falcó 38 ). The dynamic trade in seafood has raised the issue of emerging global inequity in terms of continued affordable and available seafood given current trends( 4 ). The significant diversity in current consumption of seafood (as a % of animal protein) and spatial importance of aquaculture as characterised by production intensity or contribution to the economy suggests some important mismatches. Although high production in South and Southeast Asia corresponds with this being an area of high consumption, the swathe of high consumption across west and central Africa is yet to be supported by high levels of indigenous aquaculture, despite high growth since 2000( 2 , 4 , Reference Hall, Delaporte and Phillips 39 ). Wild stocks, mainly imported cheap marine pelagic species and local freshwater fisheries, currently support most consumption needs, but intensive aquaculture has now become established in several areas of West Africa( Reference Kassam 40 , Reference Gabriel, Akinrotimi and Bekibele 41 ) and imports of farmed fish from China have also accelerated( 42 ).
In terms of importance to overall economies, aquaculture generally remains <2 % of gross domestic product, with the exceptions of Bangladesh and Vietnam where, relative to the economy as a whole, it is highly developed and important (Fig. 5). The origins of aquaculture in Asia have been linked to the importance of aquatic-relative to terrestrial-derived food in densely settled floodplains and estuarine deltas. Original sites of aquaculture that have been sustained to the modern era include the heavily populated river deltas of Southern China and coastal ponds of Java( Reference Beveridge, Little and Costa-Pierce 8 ). In contrast population densities have increased relatively recently in the Mekong Delta( Reference Keskinen, Kummu, Keskinen and Varis 43 ) and Bangladesh( Reference Streatfield and Karar 44 ).

Fig. 5. Global contribution of aquaculture to gross domestic product (GDP) by country( Reference Little and Bostock 107 ).
The consumption of aquatic v. terrestrial livestock products is a good indicator of their comparative dietary importance and a rapid assessment of the number of food vendors can be indicative, such as that conducted in Kolkata (Fig. 6). Expenditure on fresh and preserved fish exceeded that of all terrestrial meat combined in one recent study in rural Cambodia( Reference Kesaro, Schmidt and Shivakoti 46 ). In comparison with terrestrial livestock products, and particularly for poor consumers, processed forms of aquatic food are often nutritionally critical. Their importance to food security through their roles in smoothing seasonality of food supply and public health are often overlooked, or perceived as public health risks because of their association with parasites and/or adulterants of various types( Reference Little, Bush and Belton 47 , Reference Krause 48 ). Understanding how farmed and wild fish fulfil different roles in the diet remains poor; typically, even in areas where fish culture is well established, farmers and non-producers continue to source and consume both( Reference Morales, Little and Immink 49 , Reference Karim, Little and Kabir 50 ). This has implications for livelihoods both local to, and at distance from, production, and value chain analysis is increasingly used as the lens to assess such impacts( Reference Ponte, Kelling and Jesperson 51 ). It also prompts the issue of differentiating wild and farmed products, which is considered in the following section.

Fig. 6. Proportion of different food stuffs sold at market stalls in Kolkata, India( Reference Little, Kundo and Mukherjee 45 ).
Wild and farmed: the linkages
The relationship between wild stocks and farmed aquatic animals remains closely intertwined. Most products end up side by side on menus or on seafood displays, sometimes poorly identified or even the subject of fraudulent claims( Reference Pardo, Jiménez and Pérez-Villarreal 52 ). Some farmed products depend on stocking juveniles harvested from the wild or at least produced from breeding animals removed from wild habitats. Increasingly, farmers have moved towards closed cycle production, whereby captive bred breeding animals produce juveniles under controlled conditions and are increasingly the subject of selection, or other hatchery techniques, to improve their performance. An important proportion of the global harvest is produced from so-called enhanced fisheries, where natural yields are increased by stocking hatchery-produced juveniles and the imposition of management rules( Reference De Silva, Moehl and Satia 53 ).
Both fattening of wild juveniles and enhanced fisheries fall between closed cycle aquaculture and exploitation of wild stocks but tend to target different consumers and face different challenges.
Some of the world's most expensive seafood is based on harvest of wild juveniles before being farmed to a finished product. Technical control of the whole breeding cycle for the bluefin tuna (Thunnus oreintalis) and European eel (Anguilla anguilla), despite significant progress, have yet to reach commercially viable levels( Reference Endo, Ishida and Ryosuke 54 , Reference Mordenti, Casalini and Mandelli 55 ). The harvest of juvenile European eels attracts significant criticism and, as an endangered species, their harvest has been made illegal in the European Union. In contrast, some types of such capture-based aquaculture are widely perceived as being low impact and sustainable, such as the collection of spat for on growing of bivalves such as the blue mussel (Mytilus edulis). In contrast the stocking of hatchery produced juveniles in freshwater impoundments, rivers and coastal waters, also known as culture-based fisheries, has stabilised or improved access to aquatic food for food insecure inland communities. Marine ranching has had a more mixed impact, although forming the basis of major processing and export industries in the West Coast North America and (canned Pacific salmon).
In contemporary debate, polarised positions are frequently taken whereby aquaculture is framed as sustainable and in ascent and fisheries unsustainable and in decline but entrenched positions ensure that these are frequently challenged and that inverse positions are advanced( Reference Naylor, Hardy and Bureau 3 , Reference Pauly, Christensen and Guénette 56 – Reference Dierberg and Kiattisimkul 60 ).
The sustainable status of aquaculture has often focused on a narrow Western view of aquaculture based on mariculture of carnivorous species. Many farmed, especially the juvenile stages of carnivorous fish, species remain dependent on wild fish stocks processed as marine ingredients (fishmeal and fish oil) for feed. As sustainable catches of the small pelagic species that underpin the major share of the global resource base have been reached, marine ingredients represent a declining component of most farmed fish diets as feed formulators seek to substitute them with cheaper plant ingredients and improve the functionality of the replacement products. The arrival of lower trophic farmed species, which are generally less dependent on marine ingredients, such as striped catfish and tilapias, into the international seafood trade in the last 10–15 years has also realigned the fish in-fish out( Reference Jackson 61 ) relationship with steep declines in the levels of marine ingredients commonly used in most aquaculture diets( Reference Jackson 61 ). The large differences in dietary dependence are mostly related to interspecific differences in natural feeding habit. Fishmeal consumption of farmed Atlantic salmon still exceeds that of the omnivorous striped catfish in Vietnam by more than a factor of five( Reference Tacon and Metian 62 ) but inclusion levels are dropping quickly for much of the industry. Innovation towards low and non-fishmeal diets is dynamic, e.g. the recent announcement for a commercial salmon fishmeal-free diet. Innovation of this type is not uniform throughout the sector, however. From a general but highly influential critique of the use of marine ingredients in aquaculture focusing on salmon and shrimp( Reference Naylor, Goldburg and Primavera 63 ), more recent and specific analyses have turned to Asia and especially China( Reference Cao, Naylor and Hernriksson 64 ). Such studies acknowledge progress and opportunities as well as threats associated with the rapid growth and changing status of aquaculture.
The nature of the marine ingredients industry has evolved in parallel with the fisheries and aquaculture industries. All three sectors have had to find efficiency savings through better utilisation of waste and other resources, so that now an estimated 35 % of all marine ingredients are sourced from fisheries and aquaculture by-products that were previously treated as waste(Marine Ingredients Organisation (IFFO)/University of Stirling, unpublished results). The role of aquaculture itself becoming a major source of marine ingredients and strategies to enhance their value is considered later.
Delinking aquaculture feeds from marine ecosystems
A decline in reliance on marine ingredients in feed, largely because of their high unit costs, has been a major driver to change in the aquaculture sector. The increasing influence of eco-standards on international trade is also driving reductions in their use, although sustainability concerns for terrestrial feed ingredients have attracted less attention( Reference Troell, Metian and Beveridge 65 ). A major challenge is maintaining the nutritional quality for human consumption of fish in which vegetables, mainly n-6 oils, have substituted for marine lipids, mainly n-3 oils( Reference Beveridge, Brummett and Craig 66 ). A recent consumption study( Reference de Roos, Sneddon and McDonald 67 ), however, demonstrated that even fish-fed diets relatively low in fish oil (‘eco-diets’) nonetheless deliver high nutritional outcomes. Longer term, the use of high EPA-transgenic Camelina sativa oil may prove a viable alternative to maintain availability of this vital ingredient( Reference Betancor, Sprague and Sayanova 68 ), provided it gains acceptance by regulators, retailers and consumers. The search for alternative feed ingredients continues (see e.g. https://www.foodsofnorway.net)( 69 ) as for livestock in general, together with improved processing of ingredients and prophylactic health strategies through use of pro and pre-biotics. Novel ingredients such as insects show promise, although this has yet to be demonstrated on a commercial scale( Reference Barroso, de Haro and Sánchez-Muros 70 ) or gain regulatory approval in key markets. Potentially, their role in adding value to wastes through production of a quality feed ingredient can be achieved with minimal competition for resources. Similarly, the use of waste or low-value feedstocks for microbial and fungal protein has resulted in mature technologies and products, some of which already have full regulatory approval for use in livestock feeds( Reference Moen 71 ) or are already in the marketplace supporting the move of shrimps away from reliance on marine ingredients( Reference Glencross, Tabrett and Irvin 72 ). The higher relative interest in these products by the aquatic rather than the terrestrial sector reflects the former's continued dependence on high trophic species for marine ingredients. High trophic aquatic animals have a comparatively high demand for protein and also face a continuing challenge to inclusion of high levels of dietary soya. The costs of alternatives and the risks associated with investment at the necessary scale are the key constraints to the use of these types of ingredient( Reference Moen 71 ). Critiques of aquaculture frequently label it as a high-impact food sector but farmed seafood typically shares supply chains for feed ingredients with terrestrial livestock and actually consumes little more than 4 % of the total used( Reference Troell, Naylor and Metian 1 ). Life cycle assessments underline the importance of feed to the overall environmental impacts, including freshwater, land and greenhouse gas emissions for all livestock, fed-aquaculture included( Reference Roberts, Newton and Bostock 37 , Reference Pelletier 73 – Reference Nijdam, Rood and Westhoek 77 ) to an extent that in many cases food conversion ratios may be used as crude indicators of environmental impact. Innovation to reduce impacts of feeds mostly occurs upstream at the levels of ingredient sourcing, production and processing, but we now turn to environmental interactions in and around the farm.
Environmental challenges at farm and landscape
Expectations that aquaculture would be a novel source of highly nutritious food, thus relieving pressures on scant terrestrial resources, have proved to be less revolutionary than hoped. Like all human activities, aquaculture takes resources which, using inputs of energy, capital and labour, it transforms into products valued and traded by society. Impacts may be split into those occurring directly at the farm and indirect impacts occurring throughout the value chain, both up and downstream of production. Aquaculture needs space on land or in coastal waters, lakes or reservoirs in which to develop production systems. Water is needed both for physical support of farmed aquatic animals and to supply oxygen and disperse and assimilate wastes. Seed (spores, spat, post-larvae, fry or fingerlings) is required to stock the systems, and fertilisers and feeds must often be used to increase production. Energy may be required to pump water and aerate ponds, to import seed and feed onto the farm and to process and transport produce to markets. Wastes, uneaten food, faeces and metabolic wastes and chemicals (including medicines), as well as escaped organisms (including farm animals and pathogens), are inevitably released, treated or untreated, into the environment. Farms, through their physical presence alone, may also have an effect on ecosystem services and biodiversity( Reference Beveridge, Brummett and Craig 66 ).
Water use
The direct and indirect use of water, in contrast to terrestrial livestock, does not always imply consumption. Advocates of marine agronomy (marineagronomy.org) point to the independence of salt tolerant plants from limited freshwater supplies and the same is true for filter feeding animals. However, fed fin-fish and crustaceans both have varying dependencies on freshwater, whether grown either in the marine or freshwater environment related firstly to feed provision and secondly to environmental services. The water required for the environmental services; oxygen, support and dispersal of wastes, remains mostly in the biosphere and may then become unusable for other purposes such as for drinking but may also be enhanced as a source of nutrition for integrated agriculture or unaffected for use in industry( Reference Troell, Naylor and Metian 1 , Reference Beveridge, Brummett and Craig 66 , Reference Verdegem, Bosma and Verreth 78 ). How usable it is may depend on the intensity of aquaculture and the level of subsequent dilution. Assuming that little water used for environmental services is actually consumed( Reference Beveridge, Brummett and Craig 66 ), it is usually far exceeded by the amount required for provision of feed( Reference Troell, Naylor and Metian 1 , Reference Roberts, Newton and Bostock 37 ). Therefore, feed used to edible yield ratio is the key to overall livestock production ratio and unfed systems, such as marine molluscs, have a massive advantage over all fed livestock in terms of freshwater and land use. However, filter feeders can accumulate toxins from their surrounding environment and under such conditions require large amounts of energy to clean them using pure water in depuration processes. In contrast cage-farmed fish, such as Atlantic salmon, which still have fishmeal and fish oil in their diets do not have such post-harvest energy demands and have high edible yield to harvested yield ratios. Shellfish also require large quantities of energy on site for general maintenance compared with fin-fish( Reference Fry 79 ). Large greenhouse gas emissions related to energy could be mitigated by encouraging producers (e.g. reduced costs; tax breaks) to use cleaner energy, such as from wind and solar technologies.
Intensification of aquaculture
The environmental impacts of aquaculture are largely determined by species, system, production methods (i.e. whether extensive, semi-intensive or intensive), location and quality of management. Biodiversity is closely associated with the provision of ecosystem services( Reference Hall, Delaporte and Phillips 39 ). More product for less environmental impact, while retaining or improving the high dietary value of farmed seafood and ensuring high welfare outcomes for both the animals produced the people involve, are critical components of sustainable intensification( Reference Little, Bunting and Madramootoo 80 , Reference Garnett, Röös and Little 81 ). The environmental imperative for aquaculture, whereby auto-pollution can undermine productivity at the individual enterprise and broader, zonal and even global levels of production, has been a major incentive to rapid change in the sector. Managing aquatic stocks within the carrying capacity of the culture environment, well known to terrestrial pastoralists, has a particular significance for a fish farmer needing to maintain both levels of nutrition and water quality because of the acute impacts of any deterioration in the latter on the survival and growth of the stock( Reference Little, Bunting and Madramootoo 80 ). Access to plentiful water at low-cost and good system design that allows for removal of solid wastes are critical, but improvements in feeds and feed delivery that reduce waste have also been transformative( Reference Verdegem, Bosma and Verreth 78 ). This includes better nutritional formulation, pellet integrity and feed systems, all of which have reduced waste and improved feed efficiencies. Simple changes to earthen pond design have increased productivity by a factor of three in China, for example( Reference Cremer, Chappell and Zhang 82 ). Poor solids removal has been a common cause of failure in highly capitalised intensive recirculation systems and a major focus for research( Reference Barrut, Blancheton and Callier 83 ). Generally, energy efficiency increases with intensification, but access to consistent and affordable power for aeration or pumping remains a key limitation to cost effective intensification in many contexts. Tropical countries may have advantages in their potential for using solar power in transformations away from fossil-fuel-based energy. Low- and medium-income countries have often been less equipped to adapt to volatility in the fossil-fuel sector( Reference Gulati, Jacobs and Naidoo 84 ) but there are implications for reliance on various green energy supplies, including costs and reliability. Overall, there are trade-offs between various impacts, both environmental and social, and recently there have been efforts to examine these interactions through a ‘nexus’ approach that connects seemingly disparate objectives with food security being the link between them( Reference Leese and Meisch 85 ).
Aquaculture may compete with or complement agriculture for nutrients, water land and energy. This is often related to the nature of the aquaculture, particularly if it is integrated within local food systems or develops as a specialised and stand-alone activity. Detrimental effects may occur through intensification of livestock and crop production that can produce environmental impacts on aquaculture and vice versa. For example the use of agrochemicals in and around fish farms or within rice–fish systems can have negative impacts on survival and productivity of both farmed and wild aquatic animals in receiving waters( Reference Little and Muir 86 ). Management approaches can be used to mitigate worst impacts and models of chemical behaviour can guide better practice( Reference Rico, Phu and Satapornvanit 87 ). Intensive aquaculture, especially if occurring in geographical clusters, can impact on surrounding water quality to the detriment of both the aquaculture enterprise itself and other water users( Reference Soto, Aguilar-Manjarrez and Hishamunda 88 ). Apart from poorer water quality and its impact on performance, over development can lead to rapid spread of disease and economic loss( Reference Soto, Aguilar-Manjarrez and Hishamunda 88 ).
Integrated approaches
A parallel trend to intensification of farmed seafood production is integration occurring at different points in the value chain.
Traditional forms of aquaculture typically developed under conditions of nutrient scarcity and were often closely integrated with other human activities through necessity( Reference Ruddle and Zhong 89 ). A general trend to intensification has rendered many low-input traditional systems obsolete( Reference Edwards 36 ), although they are being used as templates for reducing the environmental impacts of intensive aquaculture where surplus nutrients (as wastes and by-products) can be recycled through associated food production. This is equivalent to the concept of ‘ecological leftovers’ advanced by Garnett( Reference Garnett 90 ) as a potential lens for increased sustainability of livestock production. Central to integrated aquatic production is the concept of farming filter feeding (non-fed or extractive) species alongside fed species, and in some cases aquatic plants that can take advantage of dissolved nutrients that result from such high-input systems. Typically the different components are quite separate enterprises, the sharing of space and nutrients occurring on an informal or opportunistic basis. Commercial systems exist in both freshwater and marine contexts, particularly where they have co-evolved. Such systems are widespread in coastal China. In recent years the concept, termed Integrated multitrophic aquaculture( Reference Chopin, Buschmann and Halling 91 – Reference Troell, Joyce and Chopin 93 ) has become the focus of research interest, particularly to mitigate the environmental impacts of intensive salmonid cage culture. Challenges remain to ensure the individual components are economically viable especially within the very different business enterprise and regulatory contexts of Europe and North America.
Research into integrated mariculture, targeting the retention and reuse of nutrients, is faced with the challenge of dealing with saline effluents. Inland aquaculture is more likely to be integrated with other forms of human activity, however, either formally or informally. Scarcity is ensuring that freshwater reuse is becoming increasingly multipurpose, by default. Thus, cages in common property water bodies enrich water with nutrients subsequently used for agriculture, and on-farm ponds act as reservoirs to irrigate subsistence or cash food crops nearby( Reference Beveridge, Brummett and Craig 66 ). The practice of livestock waste disposal in ponds is still common in many parts of the tropics, even where high-quality fish feeds are available, as it can reduce costs compared with complete feed-based production and reduce risks associated with livestock production. Risks to human health and potentially, greater greenhouse gas emissions( Reference Astudillo, Thalwitz and Vollrath 94 ), of waste-based aquaculture need to be considered but both can be dramatically reduced through good design. Although use of formulated diets to intensify production is a clear trend, retention and in some cases reintroduction of polycultures to produce a range of species in the same pond is widespread( Reference Diana 95 ). Much of this tendency is related to reducing risks and accessing local markets, although such practices may also improve water quality and, subsequently, productivity gains for the system as a whole. Whilst returns for the primary species remain critical, the impacts on local food security of secondary species harvested from such systems have often been ignored.
Intensification and integration are far from being mutually exclusive. Although farm intensification has often rendered the ability to horizontally integrate systems more difficult, it has opened opportunities through vertical integration which were not common or efficient in more traditional systems. The selling of by-products from fish and shrimp processing is a prime example, where previously volumes were too low, or products undervalued, to make this viable, it is now common in the salmon, tilapia, striped catfish and shrimp industries. Nevertheless, in contrast to terrestrial livestock, seafood processing is often linked to export markets, especially in Asia. The industry for processing seafood by-products thus still remains underdeveloped compared with its terrestrial counterparts.
By-product utilisation
Ultimately, the proportion of the animal that can be utilised as food or indirectly in subsequent value chains is critical to the overall profitability and environmental impact. Markets are well established for all parts of terrestrial animals, including for example leather, gelatin and other food additives but less so for aquatic where much of the by-product may be wasted or poorly utilised. Where terrestrial animals are most frequently sold as various portions or cuts, aquatic food may still often be found sold live or with minimal processing.
Aquaculture itself, particularly through reuse of by-products of processing, is becoming a major source of fishmeal and oil. The trend is being encouraged by moves to process fish close to source and making cost effective collection and processing of a wide variety of by-products viable. Hence, for striped catfish in Vietnam, stomachs and belly flaps are used as direct human food locally. New markets for higher value products, such as collagen and gelatine extracted from skin before frames and other remains are processed into lower grade fishmeal used for pigs and other fish production, are emerging( Reference Newton, Telfer and Little 96 ). Higher value protein concentrates produced from processing wastes of salmon and other high-value fish species are being developed for disease-susceptible juvenile production in both aquaculture and terrestrial livestock. Functional properties are being increasingly claimed and demonstrated for such products in both human and animal nutrition( Reference Suetsuna 97 – Reference Manikkam, Vasiljevic and Donkor 100 ). In the shrimp industry, chitin from shell by-product is being directed to manufacture various grades of chitosan that have wide ranges of applications from waste water treatment to biomedical uses. The speed of change in adding value to farmed seafood is remarkable and signifies the sector maturing and becoming more competitive with other animal products.
Aquaculture and changing impacts on livelihoods
The motivation for developing any aquaculture enterprise is increasingly driven by commercial objectives. Low input–low output, subsistence orientated aquaculture remains common in some parts of the world, especially where fish is everyday food, such as in much of Asia. Households still dependent on agriculture for much of their income typically use their aquatic resources as a ‘bank’ strategically( Reference Béné, Steel and Luadia 101 ); while selling or gifting some of their crop they will also continue buying in fish from the market and/or exploiting wild stocks. These approaches can offer reasonable income and security at a lower risk compared with high investments required for intensification. Opportunities to supply lucrative markets, however, tends to encourage intensification and attract entrepreneurs to the sector( Reference Belton, Ahmed and Murshed-e-Jahan 25 ), supported by the development of a range of upstream and downstream services. Growth in export-led markets from low- and medium-income countries-based production, initially for shrimp and more latterly for white fish species (tilapia and striped catfish), has often transformed geographical areas where it is concentrated. Clusters of production and processing have become relatively prosperous, generally related to growth in employment opportunities in the value chain as a whole. Such dynamism can also stimulate competition and quality improvement and the rise of larger-scale commercial aquaculture. Smallholders may, however, still persist in such contexts, for example shrimp culture in Thailand, in parallel with company and corporate development. Private sector standard development with its inherent need for traceability is likely to become a major factor in ensuring access to OECD markets, although penetration to other markets has scarcely begun( Reference Bush, Belton and Hall 29 ). Marginalisation of smaller-scale producers and their exclusion from the more lucrative value chains, such as has occurred in other sectors, is considered a real threat. Collective action, assisted by different domestic and international organisations, including the certifiers themselves, offers some hope that smallholder producers can be retained in such global value chains, although the speed of consolidation has been rapid in some sectors. The striped catfish sector in the Mekong Delta, Vietnam was transformed in less than a decade from a smallholder system dependent on local inputs (wild seed, human and pig manure, together with home-made feeds) supporting local demand, to a global producer of white fish, highly dependent on imported feed ingredients. In general, research suggests that employment generated by commercially orientated, family farms is likely to generate the greatest overall opportunities for rural communities to escape poverty( Reference Belton, Ahmed and Murshed-e-Jahan 25 ). The trend towards the global seafood trade, both wild capture and aquaculture, being controlled by large integrated corporate entities is therefore an issue. The resilience of the family farm, its decline much lamented but still dominant in overall food production( Reference Graeub, Chappell and Wittman 102 ), suggests that the mosaic of contemporary aquaculture systems found throughout both the richer and poorer world will persist.
Conclusions
The expected growth in both human population and per capita consumption of farmed seafood, is linked to both the decline in availability of wild stocks and growth in urban-driven purchasing power. These drivers necessitate an increase in both the scale and productivity of aquaculture. Already characterised by a huge diversity of farmed species, consolidation around fewer, genetically improved strains and species with greater scientific investment is likely in the decades ahead. Life cycle assessments indicate even current stocks and systems are comparable with, or better than most terrestrial livestock in terms of greenhouse gas, fresh water, land use and other impacts( Reference Roberts, Newton and Bostock 37 , Reference Nijdam, Rood and Westhoek 77 ). This suggests the untapped potential of aquatic animals has only just begun to be realised. The first steps, with selective breeding of farmed Atlantic salmon, shrimp and tilapias, are well underway and demonstrating potential, as is consideration of the benefits of the basic efficiencies of farming coldblooded animals. A review of change in basic feeding efficiencies of the key aquaculture species (Table 1) in the past few years suggests the rapid improvements made, on the basis of feed, breed and management. This could be expected to follow similar lines to broiler chicken development( Reference Willems, Miller and Wood 103 ). A key question is where are these major efficiencies most likely to be realised in a constantly moving food production landscape and the degree to which the three major pillars of sustainability evolve and impact on one another?
Table 1. Improvements in feed efficiencies for major aquaculture species 1995–2015

*Data from source( Reference Henriksson, Zhang and Nahid 106 ); all other data from source( Reference Tacon and Metian 62 ).
Current trajectories suggest that international trade in farmed seafood will remain a key characteristic of the sector given the advantages that tropical countries have in terms of species and environments and the trend towards consumption of processed, value-added products worldwide. Well designed and managed ponds, where environmental impacts are minimised, have a large competitive edge over more intensive technological solutions such as tank-based recirculation systems that have been developed for higher value species in OECD countries. However, the species–farm environment interaction is also dependent on consumers’ likely choices going forward and different visions of food futures( Reference Garnett 90 ). The role of technological innovation in meeting the challenges facing the sustainable intensification of aquaculture have been considered earlier, conventionally categorised within the fields of ‘feeds, genes and disease’ but increasingly advances are being made at their interface and in the context of limitations imposed by the water–nutrient–energy nexus( Reference Mo and Zhang 104 ).
Financial Support
David Little was supported by the Nutrition Society to present an oral version of this paper at their Summer Meeting 2015 at the University of Nottingham. Much of the article derives from outcomes of the Sustaining Ethical Aquaculture Trade project (no. 222889) co-founded by the European Commission within the Seventh Framework467 Programme—Sustainable Development Global Change and Ecosystem for which consortium members are acknowledged for their participation. The paper also serves as a contribution to the FAO Committee on Fisheries Sub-Committee on Aquaculture, which is working to determine the contribution of aquaculture to food security and nutrition.
Conflicts of Interest
None.
Authorship
The concept and major contribution to this article was prepared by D. L. Contributions to environment sections and figures were prepared by R. N., while major contributions to development sections were prepared by M. B. All authors had oversight of the final document regarding key messages and conclusions.