The legume family (Fabaceae) comprises more than 13 000 species, different in colour, size, shape and texture. They are mainly grown for human consumption, representing a staple food worldwide. Moreover, legumes play a prime role in human nutrition as they constitute rich sources of protein (15–45 %), high in lysine but limited in sulphur-containing amino acids, complex carbohydrates (24–68 %), starch (0·2–57·8 %), dietary fibre (1·2–25·6 %), PUFA (linolenic and linoleic), phytosterols, vitamins (niacin, pantothenic acid and folate), minerals (such as K, P, Cu, Fe, Ca and Mg), but also antinutritional factors including phytates, lectins, polyphenols, enzyme inhibitors and others(Reference Iqbal, Khalil and Ateeq1–Reference Garcia-Mora, Frias and Peñas4).
Edible legumes include bean (Phaseolus vulgaris), lima bean (Vigna lunatus), adzuki bean (Vigna angularis), mung bean (Vigna radiata), black gram (Vigna mungo), scarlet runner bean (Phaseolus coccineus), rice bean (Vigna umbellata), moth bean (Vigna acontifolia), tepary bean (Phaseolus acutifolius), broad bean (Vicia faba), garden pea (Pisum sativum var. sativum), chickpea (Cicer arietinum), dry cowpea (Vigna unguiculata), pigeon pea (Cajanus cajan), lentil (Lens culinaris), bambara groundnut (Vigna subterranea), vetch (Vicia sativa), lupins (Lupinus spp.), lablab (Lablab purpureus), sword bean (Canavalia ensiformis, gladiata), winged bean (Psophocarpus teragonolobus), velvet bean (Mucuna pruriens var. utilis), soyabean (Glycine max) and peanut (Arachis hypogaea)(Reference Duranti5,Reference Albuquerque, Nunes, Bessada and Pico6) .
In addition to their dietary contribution, legumes have bioactive compounds that exhibit diverse biological activities, for example, antioxidant, antihypertensive, immunomodulatory, hypolipidemic, antimicrobial, anticancer, hypoglycaemic and anti-inflammatory. The anti-inflammatory factors are related to obesity, cancer, rheumatoid arthritis, type 2 diabetes, inflammatory bowel disease, among others(Reference Serventi, Dsouza and Serventi7). Hence, bioactive compounds may be an alternative in the treatment. This review aims to summarise research on bioactive compounds present in legumes that exhibit anti-inflammatory effects.
Methods
A search was conducted in Science Direct, Google Scholar and PubMed databases, using the following key words, bioactive compounds in legumes, anti-inflammatory activity of legumes, biological activities of soyabean, bean, chickpea, pea, lupine, lentil and broad bean. Literature included was published in the last 15 years
Results
Inflammation
Inflammation is one of the first biological responses of the immune system; it occurs when body tissues are injured by physical trauma, intense heat, irradiation, irritating chemicals, or pathogenic infections and/or immune reactions. The word inflammation derives from the Latin word inflamo, which means I set fire. Inflammation encompasses symptoms such as redness, fever, swelling, pain and altered physiological functions to alleviate the infection and repair the damaged tissue. Depending on time and pathological characteristics, inflammation can be acute or chronic. Acute inflammation occurs immediately upon injury, lasting some seconds, minutes, hours or days; in contrast, chronic inflammation persists over time, if untreated, it could lead to tissue damage and chronic diseases, namely obesity, type 2 diabetes, inflammatory bowel disease, rheumatoid arthritis, chronic hepatitis, pulmonary fibrosis, tumour development, atherosclerosis and CVD(Reference Duodu, Apea-Bah, Taylor and Awika8–Reference Jayathilake, Visvanathan and Deen11).
The inflammatory process leads to the recruitment and activation of protective molecules, initiating a macrophage response, which serves as the first line of defence against harmful agents. Inflammatory stimuli such as lipopolysaccharides (LPS) activate macrophages to produce a variety of pro-inflammatory cytokines, including TNF-α, IL-1β, IL-6, and IL-8, interferon-γ (IFN-γ) and other inflammatory mediators such as PGE2 and nitric oxide (NO), synthesised by the enzymes cyclo-oxygenase (COX-2) and inducible nitric oxide synthase (iNOS), respectively(Reference García-Lafuente, Moro and Manchón3,Reference Galdino-Alves, Gonzalez de Mejía and Mileib-Vasconcelos12,Reference Guha and Majumder13) . Two primary signalling pathways maintain the defence system against harmful stimuli: the NF-ĸB and the mitogen-activated protein kinase (MAPK) pathway. The NF-ĸB pathway participates in cytokine production (IL-1β, IL-6, IL-8 and TNF-α), iNOS expression and COX-2 regulation. NF-ĸB proteins normally exist in the cytoplasm of inactive cells; nevertheless, they respond to stimulation, translocating into the nucleus and inducing the transcription of various pro-inflammatory genes. MAPK pathway triggers extracellular signal-regulated kinase (ERK), c-Jun N-terminal kinase (JNK) and phosphorylation of p38 MAPK, which promote inflammation(Reference García-Lafuente, Moro and Manchón3,Reference Guha and Majumder13–Reference Kim, Kawaguchi and Hayashi15) .
Common treatment of inflammation consists of steroidal anti-inflammatory drugs (EIA) and non-steroidal anti-inflammatory drugs; however, their prolonged use is associated with adverse side effects, evincing the necessity of new treatments for inflammatory diseases(Reference Huang, Lin and Lu14). Bioactive compounds, such as phenols, polysaccharides, proteins and peptides, have been identified in legumes (Tables 1 and 2), displaying anti-inflammatory effects in vivo and in vitro. The anti-inflammatory effect is generally regulated through NO, IL, TNF-α, IFN-γ and other mediators; however, their mechanisms of action have been only partially characterised(Reference Zhu, Du and Xu16) and reported in a few studies (Fig. 1).
Table 1. Bioactive compounds with in vitro anti-inflammatory activity found in legumes
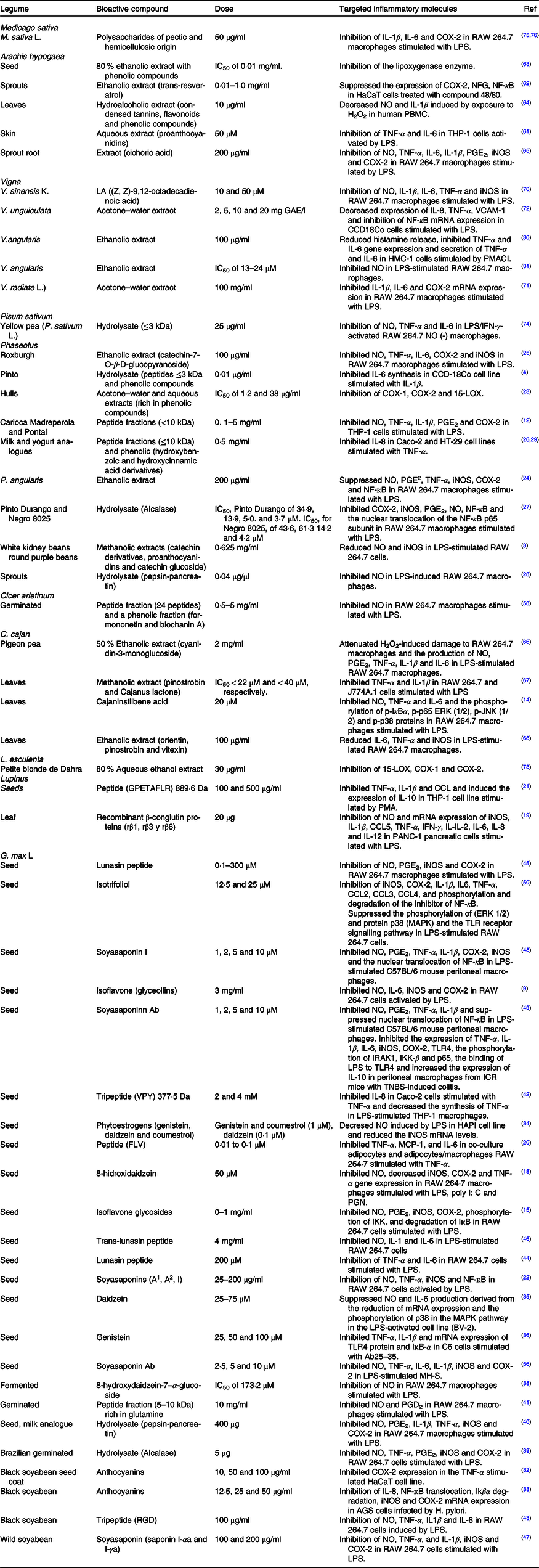
COX-2, cyclo-oxygenase; LPS, lipopolysaccharides; NFG, nerve growth factor; NO, nitric oxide; PBMC, peripheral blood mononuclear cells; iNOS, inducible nitric oxide synthase; HMC, human mast cells; PMACI, phorbol 12-myristate 13-acetate and calcium ionophore A23187; IFN-γ, interferon-γ; LOX, lipoxygenase; IĸBα, inhibitor kappa B α; ERK, extracellular signal-regulated kinase; JNK, c-Jun N-terminal kinase; TNBS, trinitrobenzene sulfonic acid; MAPK, mitogen-activated protein kinase; TLR, Toll-like receptors; IRAK, IL-1 receptor-associated kinases; IKK, IĸB kinase; MCP-1, monocyte chemoattractant protein-1; PGN, peptidoglycan; VCAM, vascular cell adhesion molecule.
Table 2. Bioactive compounds with in vivo anti-inflammatory activity found in legumes
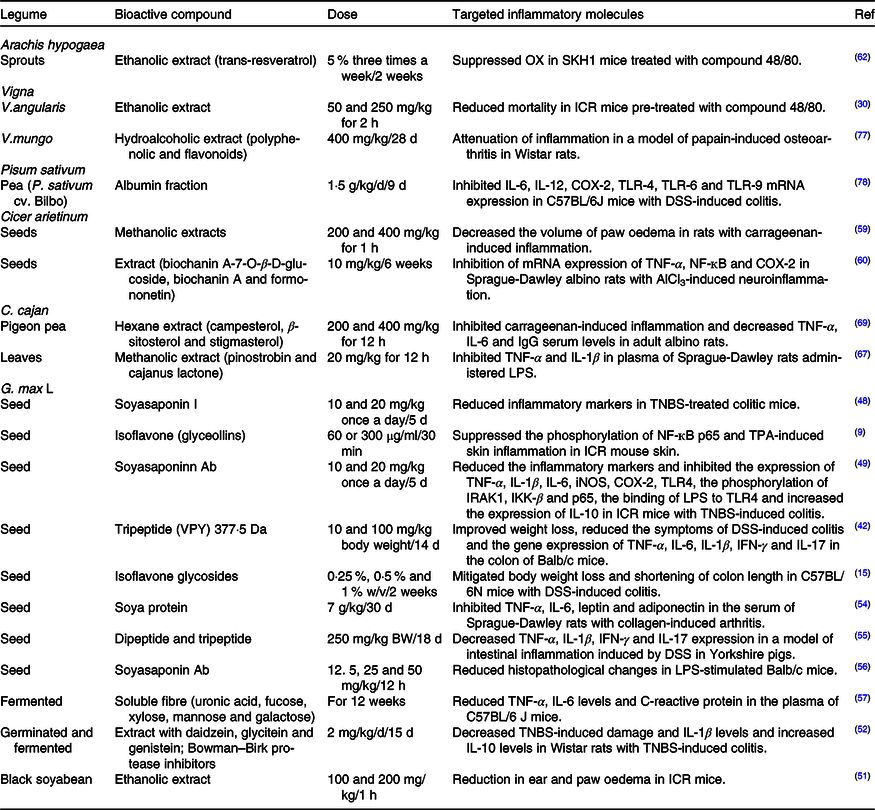
COX-2, cyclo-oxygenase; TLR, Toll-like receptors; DSS, dextran sulfate sodium; LPS, lipopolysaccharides; TNBS, trinitrobenzene sulfonic acid; iNOS, inducible nitric oxide synthase; IRAK1, IL-1 receptor-associated kinases; IKK-β, IĸB kinase; VPY, Val-Pro-Tyr; IFN, γ, interferon-γ; TPA, 12-O-tetradecanoylphorbol-13-acetate.

Fig. 1. Possible in vitro anti-inflammatory mechanisms proposed for some legumes related to LPS-activated TLR receptor. LPS, lipopolysaccharide; TLR, Toll-like receptor; COX, cyclo-oxygenase; PGE2, prostanglandin E2; NO, nitric oxide; iNOS, inducible nitric oxide synthase; MAPK, mitogen-activated protein kinases; ERK, extracellular signal-regulated kinase; JNK, c-Jun NH2-terminal kinase. Created in BioRender.com.
Bioactive compounds with anti-inflammatory activity
Phenolic compounds
Phenolic compounds represent one of the most common plants secondary metabolites. In legumes, they are typically concentrated in the seed coat; when highly pigmented, they have a higher phenolic content than non-pigmented or less pigmented ones. Phenolic compounds have an aromatic structure with one or more hydroxyl groups. Classified as flavonoids (flavones, flavonols, flavanones, isoflavones, anthocyanins, chalcones, dihydrochalcones and catechins), phenolic acids (hydroxybenzoic, hydroxyphenyl acetic, hydroxyphenyl pentanoic and cinnamic hydroxyl acids), tannins, stilbenes and lignans(Reference Albuquerque, Nunes, Bessada and Pico6–Reference Duodu, Apea-Bah, Taylor and Awika8,Reference Kumar and Kumar17) . Over the last years, phenolic compounds have attracted significant attention due to the wide variety of biological activities they exhibit, in particular, antioxidant, antitumour and anti-inflammatory effects(Reference García-Lafuente, Moro and Manchón3).
Isoflavones
Isoflavones are natural phenolic compounds in legumes such as lupin, chickpea and soyabean(Reference Kim, Kang and Kim18), which exert diverse biological activities, for instance, inflammatory effects.
Peptides and proteins
Currently, there is a growing demand for plant-derived proteins as a component of functional foods and as an alternative to the environmentally expensive production of animal protein(Reference Lima-Cabello, Morales-Santana and Foley19). In this regard, grain legumes are important sources of high-quality dietary protein; in many regions, proteins are obtained only from legumes(Reference Duranti5). Furthermore, proteins can also play a bioactive role by themselves and can be precursors of biologically active peptides with various physiological effects(Reference Duranti5,Reference Kwak, Kim and Choi20) . Bioactive peptides are short sequences of amino acids (2–20 amino acids) that remain inactive within their parental protein, but when released – by enzymatic hydrolysis, fermentation or germination, during food processing or gastrointestinal digestion – they can become immunomodulatory, antihypertensive, antithrombotic, opioid, anti-inflammatory and antioxidant agents(Reference Garcia-Mora, Frias and Peñas4,Reference Guha and Majumder13,Reference Millán-Linares, Millán and Pedroche21) .
Saponins
Saponins are a group of compounds characterised by their structure containing a carbohydrate fraction and an aglycone. When S derived from legumes (particularly lentil or soyabean), they present hypocholesterolemic, anticancer, anti-inflammatory and antioxidant activity(Reference Serventi, Dsouza and Serventi7,Reference Zha, Mao and Lu22) . Several in vitro and in vivo studies have shown the anti-inflammatory potential of legume bioactive compounds as described hereunder.
Bean
In vitro studies
Oomah et al.(Reference Oomah, Corbé and Balasubramanian23) obtained extracts (rich in phenolic compounds) from bean hulls (P. vulgaris L.); the acetone–water extract inhibited COX-1 and COX-2 enzyme expression with IC50 of 1·2 µg/ml and 38 µg/ml, respectively, whereas the aqueous extract suppressed lipoxygenase (15-LOX) enzyme expression with IC50 of 15·6 µg/ml. Likewise, Yu et al.(Reference Yu, Ahn and Shen24) obtained an ethanolic extract (0–200 µg/ml) from adzuki bean (P. angularis Wight), which suppressed the release of NO, PGE2, TNF-α, the expression of iNOS and COX-2 and the activation of NF-ĸB in RAW 264.7 macrophages stimulated with LPS. In a similar study, Fang et al. (Reference Fang, Lee and Park25) obtained an ethanolic extract from Phaseolus calcaratus Roxburgh containing catechin-7-O-b-d-glucopyranoside as a bioactive compound(100 µg/ml), which inhibited the synthesis of NO, TNF-α, and IL-6, and COX-2 and iNOS enzymes expression in LPS-stimulated RAW 264.7 macrophages, potentially through the phosphorylation of ERK 1/2, p38 MAPK and inhibitor kappa B α (IĸBα) and their subsequent degradation. Similarly, García-Lafuente et al.(Reference García-Lafuente, Moro and Manchón3) reported two extracts, one of P. Vulgaris var. white kidney bean (phenolic acids as the main components) and P. Vulgaris var. round purple bean (catechin derivatives, proanthocyanidins and glycosylated catechin) that at a concentration of 0·625 mg/ml. These extracts reduced the production of NO and mRNA expression of iNOS, IL-1β, IL-6 and TNF-α in macrophage RAW 264.7 cells stimulated with LPS, suggesting that they inhibited genetic expression by inactivating the NF-ĸB pathway. In addition, Chen et al.(Reference Chen, Zhang and Liu26) tested a phenolic fraction. Using hydroxybenzoic and hydroxycinnamic acids derivatives from bean (P. Vulgaris var. navy bean and light red kidney bean), milk and yogurt analogues, later subjected to gastrointestinal digestion in vitro and using a concentration of 0·5 mg/ml. The authors observed inhibition of IL-8 synthesis in colon carcinoma (Caco-2) and human colorectal adenocarcinoma (HT-29) cell lines stimulated with TNF-α.
Correspondingly, alcalase-treated hydrolysates from common bean (P. vulgaris L. var. Pinto Durango and Negro 8025) inhibited the production of COX-2, PGE2, iNOS and NO with IC50 (Pinto Durango) of 34·9 µM, 13·9 µM, 5·0 µM and 3·7 µM, respectively, and with IC50 (Negro 8025) of 43·6 µM, 61·3 µM, 14·2 µM and 4·2 µM, respectively. Hydrolysates also suppressed NF-κB transactivation in the nuclear translocation subunit NF-κB p65 in LPS-stimulated RAW 264.7 macrophage cell line(Reference Oseguera-Toledo, Gonzalez de Mejia and Dia27). Comparatively, pepsin-pancreatin hydrolysates from germinated black bean protein (P. vulgaris L.) at 0·04 µg/µl restricted NO synthesis in LPS-stimulated RAW 264.7 macrophages(Reference López-Barrios, Antunes-Ricardo and Gutiérrez-Uribe28). Moreover, a bean alcalase hydrolysate (P. vulgaris L. var. Pinto; peptides ≤ 3 kDa and phenolic compounds) at 0·01 µg/ml inhibited IL-6 synthesis in the IL-1β-stimulated colon myofibroblast cell line CCD-18Co(Reference Garcia-Mora, Frias and Peñas4). In other studies, Galdino-Alves et al.(Reference Galdino-Alves, Gonzalez de Mejía and Mileib-Vasconcelos12) isolated peptide fractions (<10 kDa) from cooked beans (P. vulgaris var. carioca Madreperola and Pontal) and subjected them to pepsin-pancreatin (0. 1–5 mg/ml) simulated gastrointestinal digestion, decreasing TNF-α, IL-1β and PGE2 synthesis and COX-2 expression in human THP-1 macrophage cells stimulated with LPS. And Chen et al.(Reference Chen, Zhang and Liu26,Reference Chen, Zhang and Mats29) obtained peptide fractions (≤ 10 kDa) – with a concentration of 0·5 mg/ml – from common bean (P. vulgaris var. navy bean and light red kidney bean) milk and yogurt subjected to in vitro gastrointestinal digestion, which restrained IL-8 synthesis in TNF-α-stimulated Caco-2 and HT-29 cell lines. On the other hand, Kim et al.(Reference Kim, Kim and Kim30) reported that an ethanolic extract (100 µg/ml) derived from adzuki bean (V. angularis) reduced the release of histamine in human mast cells (HMC-1) stimulated with phorbol 12-myristate 13-acetate and calcium ionophore A23187 (PMACI). The decrease in histamine occurs when reducing the levels of intracellular Ca, whose mobilisation throughout the mast cell membrane mediates histamine release. Additionally, the extract suppressed the gene expression and secretion of TNF-α and IL-6 in HMC-1 cells stimulated by PMACI, as it may block the activation of NF-κB and MAPK pathways. Lastly, Jiang et al.(Reference Jiang, Zeng and David31) isolated nine compounds (including four new furanylmethyl glycosides, angularides A-D; one ent-kaurane diterpene glycoside, angularin A; and four new triterpenoid saponins, angulasaponins A-D) from an ethanolic extract of azuki bean (V. angularis). The compounds inhibited NO production with IC50 of 13–24 µM in RAW 264.7 macrophage cells stimulated with LPS.
In vivo studies
Kim et al.(Reference Kim, Kim and Kim30) used an ethanolic extract from azuki bean (V. angularis) in a ICR mice model of compound 48/80-induced systemic anaphylaxis. As a result, it reduced mortality in mice when pre-treated with the extract at 50 mg/kg and 250 mg/kg.
Soyabean (Glycine max L.)
In vitro studies
Kim et al.(Reference Kim, Xu and Chang32) evaluated the effects of anthocyanins from black soyabean seed coat at concentrations of 10 µg/ml, 50 µg/ml and 100 µg/ml, which inhibited COX-2 enzyme expression in the TNF-α-stimulated HaCaT cell line. Besides, Kim et al.(Reference Kim, Kim and Park33) assessed the effect of anthocyanins (cyanidin-3-glucoside, delphinidin-3-glucoside and petunidin-3-glucoside) from black soyabean on Helicobacter pylori-infected human gastric epithelium (AGS) cells. At concentrations of 12·5 µg/ml, 25 µg/ml and 50 µg/ml, anthocyanins decreased IL-8 secretion, iNOS, and COX-2 mRNA expression, ERK, JNK, and p38 MAPK phosphorylation, NF-κB translocation and degradation of Iĸβα.
Moreover, studies have reported the inhibitory activity of isoflavones isolated from soyabean against inflammatory mediators. Genistein (1 μM), coumestrol (1 μM) and daidzein (0·1 μM) decreased NO production, reduced iNOS mRNA levels, down-regulated the transcription factor interferon regulatory factor 1 (IRF-1) and phosphorylated STAT1, which modulate iNOS expression in LPS-activated HAPI rat microglial cells(Reference Jantaratnotai, Utaisincharoen and Sanvarinda34). Likewise, daidzein (25–75 μM) suppressed NO production, IL-6 mRNA expression and p38 phosphorylation in the MAPK pathway in the LPS-activated murine microglial cell line (BV-2)(Reference Chinta, Ganesan and Reis-Rodrigues35). Analogously, genistein (25 µM, 50 µM and 100 µM) prevented the release of TNF-α and IL-1β and inhibited the expression of Toll-like receptors 4 (TLR4) protein and Iĸβ-α mRNA in rat glioma cells (C6) stimulated by β-amyloid peptides 25–35 (Ab25–35)(Reference Ma, Ding and Yu36). Furthermore, a soyabean-derived compound consisting of genistein and Bowman–Birk trypsin inhibitor (100 mg/kg) reduced serum levels of TNF-α and IFN-γ and decreased TNF-α and IFN-γ mRNA expression in splenocytes of Balb/c mice with LPS-induced inflammation(Reference Sadeghalvad, Mohammadi-Motlagh and Karaji37). Another examination indicated that 8-hydroxidaidzein (50 µM) hindered NO synthesis, decreased iNOS, COX-2, and TNF-α gene expression, and constrained NF-κB and activator protein I (AP-1) transcriptional activities in RAW 264.7 macrophages stimulated with LPS, polyinosinic-polycytidylic acid (poly [I:C], a TLR3 inducer) and peptidoglycan (PGN, a TLR2 inducer)(Reference Kim, Kang and Kim18). Similarly, 8-hydroxydazidzein-7-α-glucoside (IC50 of 173·2 µM) prevented the synthesis of NO in LPS-stimulated RAW 264.7 macrophages(Reference Chang, Wang and Yang38). Also, isoflavone glycosides (3 mg/ml) limited the production of NO and PGE2, suppressing iNOS, COX-2 and inflammatory mediators involved in the NF-ĸB signalling pathway, through inhibition of IKKB phosphorylation and IĸB degradation in RAW 264.7 macrophages stimulated with LPS(Reference Kim, Kawaguchi and Hayashi15).
Comparably, an alcalase-treated hydrolysate from germinated soyabean protein (at 5 µg of protein) restricted the synthesis of NO, TNF-α, and PGE2 and the expression of iNOS and COX-2 in LPS-stimulated RAW 264.7 macrophages(Reference Vernaza, Dia and Gonzalez De Mejia39). In another study, Dia et al. (Reference Dia, Bringe and González De Mejia40) obtained pepsin-pancreatin hydrolysates from soya milk analogues with a concentration of 400 µg, which suppresses the production of NO, PGE2, IL-1β, and TNF-α and the expression of iNOS and COX-2 enzymes in RAW 264.7 macrophages stimulated with LPS. González-Montoya et al.(Reference González-Montoya, Hernández-Ledesma and Silván41) isolated peptide fractions from soyabean sprouts hydrolysed with pepsin-pancreatin enzymes and observed that in 5 kDa to 10 kDa peptide fraction (rich in glutamine) at a concentration of 10 mg/ml, they induced the highest inhibition of NO and PGD2 in RAW 264.7 macrophage cells stimulated with LPS, attributable to the ability of peptides to restrict the expression of iNOS and COX-2 enzymes. Besides, a Val-Pro-Tyr (VPY) tripeptide with a molecular mass of 377·5 Da isolated from soyabean at a concentration of 2 mM and 4mM hampered IL-8 secretion in Caco-2 cells stimulated with TNF-α, and at a concentration of 1 μM and 10 μM, and decreased the synthesis of TNF-α in human THP-1 macrophages stimulated with LPS(Reference Kovacs-Nolan, Zhang and Ibuki42). Similarly, a Phe-Leu-Val (FLV) peptide derived from soyabean β-conglycinin, at concentrations of 0·01 µM to 0·1µM blocked the release of TNF-α, monocyte chemoattractant protein-1 (MCP-1) and IL-6, from both TNF-α-stimulated adipocytes and co-cultured adipocytes/macrophages. An effect was mediated by the inactivation of the signalling molecules JNK and IĸB kinase (IKK) and the down-regulation of IĸBα in the adipocytes(Reference Kwak, Kim and Choi20). In addition, Arg-Gly-Asp tripeptide (RGD) was purified from germinated black soyabean and treated with high hydrostatic pressure (150 MPa) at 100 μg/ml. It repressed the expression of NO, TNF-α, IL-1β and IL-6 in LPS-induced RAW 264.7 macrophages(Reference Kim, Jang and Lee43). The lunasin peptide (43 amino acids) isolated from soyabean at a concentration of 200 µM hindered the synthesis of TNF-α and IL-6 in LPS-stimulated RAW 264.7 macrophages(Reference Hernández-Ledesma, Hsieh and de Lumen44), and at concentrations of 0·1–300 µM, restricted the production of NO, PGE2, and the expression of iNOS and COX-2 in the same cell line. These results suggest that lunasin could prevent inflammation by obstructing the COX-2/PGE2 and iNOS/NO pathways(Reference Dia, Wang and Oh45). Finally, the trans-lunasin peptide, at a concentration of 4 mg/ml, inhibits the synthesis of NO, IL-1 and IL-6 in RAW 264.7 macrophages stimulated with LPS(Reference Hao, Fan and Guo46).
Soyasaponins in soyabean are important bioactive secondary metabolites that shown anti-inflammatory activity(Reference Yang, Le and Androutsopoulos47). Lee et al.(Reference Lee, Park and Yeo48,Reference Lee, Park and Joh49) isolated soyasaponin I and soyasaponin Ab, which exhibited an inhibitory effect (1, 2, 5 and 10 µM) on the production of NO, PGE2, TNF-α, IL-1β and the phosphorylation of IκBα, and the nuclear translocation of NF-κB in LPS-stimulated peritoneal macrophages from C57BL/6 mice. Particularly, soyasaponin I attenuated the activation of the NF-κB transcription factor, and soyasaponin Ab decreased the expression of TLR4, the phosphorylation of IL-1 receptor-associated kinases (IRAK1), IKK-β, and p65 and increased IL-10 expression and blocked the binding of LPS to TLR4 in peritoneal macrophages. Similarly, soyasaponin structures (soyasaponin A1, A2, I) at a dose of 25–200 µg/ml restricted the production of NO and TNF-α, the enzymatic activity of iNOS and the activity of NF-ĸB in RAW 264.7 macrophages activated by LPS(Reference Zha, Mao and Lu22). In another study, Yang et al.(Reference Yang, Le and Androutsopoulos47) tested a crude extract of soyasaponins (saponin I-αa and I-γa), which at a concentration of 100 μg/ml and 200 μg/ml decreased NO production and regulated iNOS and COX-2 enzymes expression in RAW 264.7 macrophages stimulated with LPS. Soyasaponins I-α also constrained TNF-α and IL-1β expression; however, soyasaponin I-γa only inhibited TNF-α expression. The authors proposed that the anti-inflammatory effect of soyasaponins I was mainly mediated through the phosphorylation of p38 and JNK proteins.
On the other hand, Li et al.(Reference Li, Yoon and Won50) obtained coumestans (coumestrol, isotrifoliol and phaseol) from soyabean leaf. At concentrations of 12·5 μM and 25 μM, reduced NO and PGE2 production in RAW 264.7 macrophages was activated by LPS. Isotrifoliol (at 12. 5 μM) or 25 μM) showed the greatest reduction of mRNA expression of iNOS, COX-2, IL-1β, IL6, TNFα, CCL2, CCL3 and CCL4. Besides, isotrifoliol diminished phosphorylation and degradation of the NF-κB inhibitor, suppressed ERK1/2 and p38 MAPK phosphorylation, and restrained the TLR signalling pathway.
In vivo studies
In vivo, pharmacological evaluation studies have shown the soyabean capacity to attenuate inflammation. Yim et al.(Reference Yim, Lee and Choi51) reported that an ethanolic extract (200 mg/kg) from black soyabean reduced arachidonic acid-induced ear oedema and carrageenan-induced paw oedema in ICR mice models. Moreover, daidzein, glycitein, genistein and Bowman–Birk protease inhibitors administered for 15 d (2 mg/kg/d) decreased microscopic damage, enhanced intestinal permeability, visceral hypersensitivity, and intestinal proteolytic activity, and increased IL-1β and IL-10 levels in Wistar rats with colitis induced by 2, 4, 6 trinitrobenzene sulfonic acid (TNBS)(Reference Moussa, Bézirard and Salvador-Cartier52).Comparably, apigenin administration to C57BL/6 mice with ulcerative colitis induced by dextran sulfate sodium attenuates clinical signs of the disease and down-regulated the expression of microsomal prostaglandins (mPGES), COX-2, iNOS and serum matrix metalloproteinase-3 (MMP-3). Authors demonstrated that apigenin action is linked with the inhibition of canonical and non-canonical inflammasome pathways(Reference Márquez-Flores, Villegas and Cárdeno53). In the same model, isoflavone glycosides (0·25 %, 0·5 % and 1 % [w/v]) mitigated body and colon weight loss(Reference Kim, Kawaguchi and Hayashi15). In another study, soya protein (7 g/kg) limited TNF-α, IL-6, leptin and adiponectin production in the serum of Sprague-Dawley rats with collagen-induced arthritis(Reference Shahi, Rashidi and Mahboob54).Consistent results were reported for 377·5-Da VPY tripeptide isolated from soyabean. When administered at concentrations of 10 mg/kg and 100 mg/kg body weight for 14 d to Balb/c mice, it reduced dextran sulfate sodium-induced colitis symptoms and decreased weight loss and gene expression of TNF-α, IL-6, IL-1β, IFN-γ and IL-17 in the colon(Reference Kovacs-Nolan, Zhang and Ibuki42). Similarly, soya-derived di- and tripeptides (150–500 Da) decreased the expression of TNF-α, IL-1β, IFN-γ and IL-17 in a model of dextran sulfate sodium-induced intestinal inflammation in Yorkshire pigs(Reference Young, Ibuki and Nakamori55). Soyasaponin I and soyasaponin Ab (10 and 20 mg/kg) orally administered to ICR mice with TNBS-induced colitis improved body weight reduction and prevented colon shortening, myeloperoxidase catalytic activity, TNF-α, IL-1β, IL-6, iNOS and COX-2 expression(Reference Lee, Park and Yeo48,Reference Lee, Park and Joh49) . Furthermore, soyasaponin Ab (12·5 mg/kg, 25 mg/kg and 50 mg/kg) diminished lung histopathological changes (interstitial oedema, hyperaemia, thickening of the alveolar wall and diffuse interstitial infiltration by inflammatory cells) in Balb/c mice stimulated with LPS to induce acute lung injury. It can also decreased the production of NO, TNF-α, IL-6, and IL-1β and the expression of iNOS and COX-2 in murine alveolar macrophages (MH-S) stimulated with LPS(Reference Lin, Cheng and Wang56). Lastly, Kim et al.(Reference Kim, Jia and Lee57) reported that soluble fibre – consisting of uronic acid, fucose, xylose, mannose and galactose – obtained from fermented soyabeans reduced TNF-α, IL-6 and C-reactive protein levels in the plasma of C57BL/6J mice.
Chickpea (C. arietinum L.)
In vitro studies
Chickpea isoflavones such as formononetin and biochanin A (0·5–5 mg/ml) show inhibitory activity on NO synthesis in RAW 264.7 macrophages stimulated with LPS(Reference Milán-Noris, Gutiérrez-Uribe and Santacruz58). Besides, a peptide fraction (24 peptides) with molecular weight between 1·1 kDa and 2·3 kDa isolated from a germinated and digested chickpea protein concentrate (0·5–5 mg/ml) lowered NO synthesis in RAW 264.7 macrophages stimulated with LPS(Reference Milán-Noris, Gutiérrez-Uribe and Santacruz58).
In vivo studies
Masroor et al.(Reference Masroor, Baig and Ahmed59) evaluated methanolic extracts of chickpea (var. Desi and Kabuli) at doses of 200 mg/kg and 400 mg/kg, which decreased paw oedema volume in rats with carrageenan-induced inflammation. Moreover, isoflavones were isolated from chickpea (C. arietinum L.) – that is, biochanin A-7-O-β-D-glucoside, biochanin A and formononetin – administered at a dose of 10 mg/kg to Sprague-Dawley albino rats with aluminium chloride (AlCl3)-induced neuroinflammation, inhibiting TNF-α, NF-ĸB and COX-2 mRNA expression(Reference Wahby, Mohammed and Newairy60).
Peanut (A. hypogaea L.)
In vitro studies
Tatsuno et al.(Reference Tatsuno, Jinno and Arima61) obtained an aqueous extract (proanthocyanidins) from peanut skin at a concentration of 50 µM, and it attenuated TNF-α and IL-6 synthesis in human monocytic cells (THP-1) activated by LPS. Likewise, Choi et al.(Reference Choi, Choi and Kim62) reported that an ethanolic extract (trans-resveratrol) from peanut sprouts suppressed COX-2 and nerve growth factor expression. It also obstructed transcription factor NF-κB activity in a human epidermal keratinocyte cell line (HaCaT) treated with a polymer compound 48/80. Additionally, Limmongkon et al.(Reference Limmongkon, Nopprang and Chaikeandee63) observed that a peanut seed-derived ethanolic extract inhibited the LOX enzyme, with an IC50 of 0·01 mg/ml. Cossetin et al.(Reference Cossetin, da Silva-Brum and Casoti64) obtained a peanut leaf extract using 70 % ethanol, which contained condensed tannins, flavonoids and phenolic compounds (10–100 μg/ml), and detected that the extract decreased NO production and IL-1β levels (10 μg/ml) induced by previous exposure to H2O2 in human peripheral blood mononuclear cells. In a recent study, Lee et al.(Reference Lee, Son and Kim65) analysed an extract (cichoric acid) from peanut sprout root, which at a dose of 200 µg/ml reduced NO, TNF-α, IL-6, IL-1β, and PGE2, and restrained iNOS and COX-2 expression in LPS-stimulated RAW 264.7 macrophage cells. The anti-inflammatory effect of the extract was explained through inhibition of MAPK (ERK, JNK and p38) phosphorylation and activation of NF-ĸB.
In vivo studies
A peanut sprout ethanolic extract rich in trans-resveratrol at 5 % concentration suppressed the oxazolone (OX)-induced effects of oedema, scaling and thickening in a model of OX-induced contact dermatitis in SKH1 mice(Reference Choi, Choi and Kim62).
Pigeon pea (C. cajan L.)
In vitro studies
Lai et al.(Reference Lai, Hsu and Huang66) obtained an ethanol (cyanidin-3-monoglycoside) extract from pigeon pea. At a concentration of 2 mg/ml attenuated H2O2-induced DNA damage in RAW 264.7 macrophage cells and reduced NO, PGE2, TNF-α, IL-1β and IL-6 levels in RAW 264.7 LPS-stimulated macrophages. In a similar examination, a pinostrobin and cajanus lactone isolate extracted with methanol hampered the synthesis of TNF-α (IC50 < 22 µM) and IL-1β (IC50 < 40 µM) in LPS-stimulated RAW 264.7 and J774A.1 cells(Reference Patel and Bhutani67). Correspondingly, an ethanolic extract (100 µg/ml) from pigeon pea leaves containing orientin, pinostrobin, and vitexin reduced IL-6 and TNF-α secretion, and iNOS expression in RAW 264.7 macrophages cell line activated by LPS(Reference Schuster, Holzer and Doerfler68). In another study, Huang et al. (Reference Huang, Lin and Lu14) isolated cajan instilbene acid from pigeon pea leaves, which at a concentration of 20 μM obstructed the release of NO, TNF-α and IL-6 in RAW 264.7 murine macrophages stimulated with LPS. Also, this compound blocked the phosphorylation of IκBα, p65, ERK (1/2), JNK (1/2), and p38 proteins, involved in NF-κB and MAPK pathways. These results suggest that the anti-inflammatory activity is due, at least in part, to the suppression of NF-κB and the activation of the MAPK pathway in LPS-stimulated macrophages.
In vivo studies
Patel et al.(Reference Patel and Bhutani67) obtained a methanolic extract from pigeon pea leaves, which at a dose of 20 mg/kg reduced TNF-α and IL-1β levels in the plasma of Sprague-Dawley rats treated with LPS. The authors ascribed the effect of pinostrobin and cajanus lactone content on the extract. Finally, Hassan et al.(Reference Hassan, Matloub and Aboutabl69) tested a pigeon pea hexane extract, composed of unsaponifiable matter, that is, campesterol, β-sitosterol and stigmasterol at concentrations of 200 mg/kg and 400 mg/kg. It reduced carrageenan-induced inflammation and decreased TNF-α, IL-6 and IgG serum levels in adult albino rats.
Other legumes
In vitro studies
Although other legumes species contain bioactive compounds, literature remains scarce. Nevertheless, this section outlines the existing research. Lee et al.(Reference Lee, Lee and Cui70) obtained n-butanol fractions (oleanolic acid, LnA ((Z, Z, Z) −9,12,15-octadecatrienoic acid) and LA ((Z, Z) −9,12 -octadecadienoic acid)) from a methanolic extract of cowpea (V. sinensis K.). LA compound at concentrations of 10 μM and 50 μM inhibited the production of NO, IL-1β, IL-6, and TNF-α and the expression of iNOS, IL-1β, IL-6, and TNF-α mRNA in LPS-stimulated RAW 264.7 macrophage cells.
In another study, Zhang et al.(Reference Zhang, Shang and Qin71) obtained an acetone–water extract (100 mg/ml) from mung bean (V. radiate L.), which suppressed IL-1β, IL-6 and COX-2 mRNA expression in LPS-stimulated RAW 264.7 macrophages. Analogously, an acetone–water extract from cowpea (V. unguiculata) at concentrations of 2 mg GAE/l, 5 mg GAE/l, 10 mg GAE/l and 20 mg GAE/l decreased IL-8, TNF-α, vascular cell adhesion molecule (VCAM)-1 and NF-κB mRNA expression in non-malignant colon myofibroblast cells (CCD18Co) stimulated with LPS(Reference Ojwang, Banerjee and Noratto72). Moreover, Boudjou et al.(Reference Boudjou, Oomah and Zaidi73) evaluated an 80 % ethanolic extract of lentil hulls (Lens esculenta var. Petite blonde de Dahra), which at a concentration of 30 µg/ml inhibited 15-LOX, COX-1 and COX-2 enzymes with an IC50 of 54·6 µg/ml, 65·8 µg/ml and 119 µg/ml, respectively. The activity is attributed to the phenolic acids in the extract, such as syringic acid, trans-p-coumaric acid, ferulic acid, trans-cinnamic acid and epicatechin.
On the other hand, recombinant β-conglutin proteins (20 µg) (rβ1, rβ3 and rβ6) purified from narrow-leafed lupin (L. angustifolius) inhibited NO production and iNOS, IL-1β, CCL5, TNF-α, IFN-γ, IL-2, IL-6, IL-8 and IL-12 mRNA expression in pancreatic cells (PANC-1) stimulated with LPS(Reference Lima-Cabello, Morales-Santana and Foley19). Likewise, peptide Gly-Pro-Glu-Thr-Ala-Phe-Leu-Arg (GPETAFLR) – with a molecular mass of 889·6 Da, obtained by hydrolysis (alcalase) of lupine protein (L. angustifolius L.) and subsequently synthesised (100 and 500 µg/ml) – suppressed the expression of TNF-α, IL-1β, and CCL2 and induced the expression of IL-10 in human monocytic THP-1 cell line stimulated with phorbol 12-myristate 13-acetate (PMA)(Reference Millán-Linares, Millán and Pedroche21).
In another study, a hydrolysate (thermolysin, ≤3 kDa) of yellow pea protein (Pisum sativum L.) at a concentration of 25 µg/ml hindered the synthesis of NO, TNF-α and IL-6 in RAW 264.7 macrophage cells activated with LPS/IFN-γ (Reference Ndiaye, Vuong and Duarte74). Lastly, Chen et al.(Reference Chen, Liu and Zhang75,Reference Chen, Liu and Zhang76) purified two polysaccharides. One pectic (rhamnose, galactose, galacturonic acid and arabinose) and one hemicellulosic (xylose, glucuronic acid and arabinose) from an alkaline extract of alfalfa (Medicago sativa L.), which at a concentration of 50 μg/ml obstructed mRNA expression of IL-1β, IL-6 and COX-2 genes in RAW 264.7 mouse macrophage cells stimulated with LPS.
In vivo studies
Patel et al.(Reference Patel, Sawant and Kaur77) evaluated a hydroalcoholic extract containing polyphenols and flavonoids from black gram (V. mungo) in a model of papain-induced osteoarthritis in Wistar rats. The extract at a dose of 400 mg/kg attenuated the inflammatory process by reducing the diameter of the knee joint and improving grip strength and locomotion activity, with slight muscle degeneration and cartilage erosion. On the other hand, Utrilla et al.(Reference Utrilla, Peinado and Ruiz78) assessed a pea (Pisum sativum cv. Bilbo) albumin fraction, administered at a dose of 1·5 g/kg/d to C57BL/6J mice with dextran sulfate sodium-induced colitis, which repressed the expression of IL-6, IL-12, COX-2 mRNA and TLR (TLR-4, TLR-6 and TLR-9).
Discussion
The anti-inflammatory activity of soyabean is highly documented in both in vivo and in vitro studies. Purified bioactive compounds from soyabean, for example, isoflavones, saponins and peptides, indicate promising results against inflammation. In addition, bean extracts and protein hydrolysates have shown anti-inflammatory activity (most examinations are in vitro). Few studies have analysed the exact compound from the extract that may induce the anti-inflammatory activity. Regarding protein hydrolysates, most research solely reports peptide fractions of certain molecular weights but not the peptide sequence that may exert biological activity. Therefore, it is necessary to purify the bean bioactive compounds that show anti-inflammatory activity and evaluate them via in vivo models. Research on chickpea, peanut, pea, lentil, and other legumes have identified extracts (phenolic compounds), carbohydrates, and hydrolysates with anti-inflammatory activity. Nevertheless, there are few in vivo and in vitro studies concerning bioactive compounds derived from these legumes. Therefore, it represents an area of opportunity for further research, especially on how bioactive compounds induce anti-inflammatory activity and testing such effects in vivo.
Conclusions
Legumes are a source of protein, carbohydrates, vitamins and minerals; however, their health benefits go beyond their nutritional value. To date, studies focus on bioactive compounds from legumes as alternative therapies to the pharmacological treatment of various chronic inflammatory diseases. Most research on the anti-inflammatory activity of bioactive compounds isolated from legumes is limited to soyabean and bean. In contrast, legumes such as lupin, alfalfa, lentil, pea and chickpea have been less studied, and a limited number of them have dealt with the possible mechanism of their anti-inflammatory action. Their activity could be explained because of inactivation of the signalling pathways of NF-KB and MAPK, through inhibition of the nuclear translocation of NF-ĸB and suppression of IĸBα, ERK, JNK, and p38 phosphorylation, reducing the synthesis of inflammatory markers. These compounds may restrain the expression of enzymes, such as COX-2 and iNOS, decreasing the synthesis of NO and PGE2. Due to the scarce information about how these bioactive compounds produce anti-inflammatory effects, a change in the research approach in this area is essential, especially, regarding the use and application of less studied legumes that represent a good alternative as a source of compounds with anti-inflammatory activity.
Acknowledgements
Authors thank the Instituto Politécnico Nacional for the financial support through grant SIP-20210017.
J.-C.M.F.: conceptualisation, investigation and writing-original draft. M.-M.O.G.: investigation and formal analysis. M.F.Y.K.: methodology and validation. J.-M.C.: conceptualisation, investigation and writing – review and editing.
The authors declare no conflicts of interest.