Butyrate is one of the SCFA produced by bacterial fermentation of carbohydrates (dietary fibre, resistant starch) in the colon of single-stomached species including swine and man(Reference Cummings and Macfarlane1). Butyrate is also naturally released from milk by pregastric lipases during suckling in young animals. Butyrate is known to inhibit the growth of both Gram-positive (for example, enterococci) and Gram-negative (for example, coliforms) bacteria(Reference Sun, O'Connor and Roberton2). Butyrate is quickly absorbed and can be utilised directly as an energy source by colonic epithelial cells(Reference Roediger3). In addition to being an important fuel for colonocytes, several studies have indicated that butyrate increases mucosal proliferation and improves epithelial cell differentiation and colonic barrier function(Reference Cook and Sellin4–Reference Kinoshita, Suzuki and Saito6). Furthermore, butyrate protects the intestine as shown in studies of total parenteral nutrition in rats where it retards the associated mucosal atrophy(Reference Koruda, Rolandelli and Bliss7). Butyrate is also involved in intestinal adaptation by increasing cell proliferation and decreasing apoptosis(Reference Scheppach and Weiler8). In other studies, butyrate has been reported to affect the immune system(Reference Weber and Kerr9) and to exert anti-inflammatory properties(Reference Segain, Raingeard de la Blètiere and Bourreille10, Reference Venkatraman, Ramakrishna and Shaji11). However, the effects of butyrate depend on the experimental model (in vitro or in vivo), the state of the cells (normal or cancerous(Reference Pender, Quinn and Sanderson12)), the degree of inflammation(Reference Inagaki and Sakata13) and the dose (proliferative at low dose, anti-proliferative at high dose(Reference Velazquez, Lederer and Rombeau14)) leading to the concept of the ‘butyrate paradox’(Reference Kien, Schmitz-Brown and Solley15).
The effects of butyrate on colonic tissues after stimulation of its production by butyrogenic dietary substrates(Reference Kien, Blauwiekel and Bunn16) or after caecal administration(Reference Kien, Blauwiekel and Bunn16) have been described extensively, whereas its effects on intestinal biology and function after oral administration are poorly documented. Due to anti-bacterial, anti-inflammatory and proliferative properties, butyrate is an attractive potential alternative to antibiotic growth promoters that have been banned from farms in the European Union since 1 January 2006. Therefore, several studies have been carried out to investigate the effects of sodium butyrate (SB) on growth, small intestine (SI) morphology and digestibility in weaned piglets. However, conflicting results have been reported. Gálfi & Bokori(Reference Gálfi and Bokori17) were the first to show higher growth performances in pigs weighing from 7 to 102 kg and receiving SB at 1·7 g/kg feed. Then, Piva et al. (Reference Piva, Morlacchini and Casadei18) showed that feeding SB at 8 g/kg to piglets in the first 8 weeks after weaning improved the average daily gain and the average daily feed intake during the first 2 weeks. In contrast, Manzanilla et al. (Reference Manzanilla, Nofrarias and Anguita19) reported improved feed efficiency but unchanged growth performance as well as deeper jejunal crypt depth, increased colonic goblet cells numbers and reduced digestibility of organic matter and starch in weaned pigs receiving SB (3 g/kg DM intake) for 14 d after weaning. Biagi et al. (Reference Biagi, Piva and Moschini20) did not observe any modification of intestinal mucosal morphology in piglets receiving SB at 1 to 4 g/kg during the 6 weeks after weaning. SB provided orally soon after birth was found to increase growth and stimulate intestinal maturation(Reference Kotunia, Woliński and Laubitz21).
To our knowledge, very few experiments have been conducted in milk-fed young mammals. With our preliminary results in piglets(Reference Kotunia, Woliński and Laubitz21) and calves(Reference Guilloteau, Zabielski and David22) which suggest that SB provided orally soon after birth could increase body growth and intestinal maturation, we undertook the present research to investigate the influence of the period of administration of SB (before and/or after weaning) on growth rate, feed intake and gastrointestinal tract (GIT) architecture and function in young pigs.
Materials and methods
Animals and experimental design
The experimental procedures were carried out according to the guidelines of the French Ministry for Animal Research. The present study involved two periods with young pigs: the suckling period from day 5 after birth until weaning at day 28, and the post-weaning period from day 29 to days 39–40 for the first batch and to days 40–41 for the second batch of pigs. Two factors were investigated: SB before weaning and SB after weaning. These factors were combined in order to get four treatments: pigs with no SB supplementation (CC group; control), pigs supplemented with SB only before weaning (BC group) or only after weaning (CB group) and pigs supplemented both before and after weaning (BB group).
Thirty-two crossbred (Pietrain × Large White × Landrace) piglets from the experimental herd of the National Institute for Agricultural Research (INRA; Saint-Gilles, France) were blocked within litters into quadruplicates homogenous for birth weights and growth rates over the first 4 d of life. One pig from each block was randomly assigned to one of the four experimental treatments. The study was conducted in two consecutive batches of four litters each.
During the suckling period, the SB solution or the saline solution used as the control treatment was carefully administered by a 5–10 ml plastic syringe fitted with an oesophageal tube twice daily (at 09.00 and 15.00 hours), as previously reported in detail(Reference Mazzoni, Le Gall and De Filippi23). SB was supplied at a concentration of 3 g/kg of the daily intake of milk DM as estimated during the suckling period from individual growth rates, using the equation of Noblet & Etienne(Reference Noblet and Etienne24). The piglets were kept with their littermates and the sow in the farrowing unit throughout the suckling period.
At day 28, the experimental pigs were transported to the weaning building where air temperature (27–28°C) and light (08.00–21.00 hours) were automatically controlled. They were housed in individual cages (0·6 × 0·8 m) with a mesh floor. The starter diets were made available from the day of weaning onward. Between day 28 and day 32, the amount of feed offered was progressively increased from 10 to 80 % of the INRA recommendation(25). This was made for minimising the risk of over-feeding and subsequent GIT disorders and scours often seen with ad libitum feeding immediately after weaning. Feed intake amounted to 70 g per kg metabolic body weight (BW0·75) at day 32. Amounts of feed covering 100 % of the requirements were provided from day 33 until the end of the trial. The weaning diets were offered twice daily (at 09.00 and 15.00 hours) as a mash with a feed:water ratio of 1:1 (w/w) during the first 3 d after weaning and 2:1 thereafter. Water was provided ad libitum.
Experimental solutions given orally and weaning diets
Solutions of saline (9 g NaCl/l) and SB (60 g/l, reference 303410; Sigma, St Quentin Fallavier, France) were prepared for oral administration to young pigs during the suckling period. The pH of both solutions was adjusted to 7·0 with sodium hydroxide. Two weaning starter diets were formulated (Table 1). Both diets were similar in composition, except that SB was introduced (3 g/kg DM) at the expense of maize starch in one of them. This dose was based on data available in the literature to use SB amounts in a physiological range. Titanium dioxide and chromic oxide were added to the diets as faecal and ileal digestibility markers(Reference Wilfart, Montagne and Simmins26), respectively. No antimicrobial agent was added to the diets.
Table 1 Composition of the control weaning diet*

* Calculated nutrient composition (as-fed basis, g/kg): DM, 888; crude protein, 191; crude fibre, 34; fat, 50; Ca, 7·2; Na, 2·5; P, digestible, 3·65; lysine, 12·5; methionine, 4·4; tryptophan, 2·5; threonine, 8·0; net energy, 10·0 MJ/kg.
† Soycomil® (ADM, LJ Koog aan de Zaan, The Netherlands).
‡ Maize starch (40 g/kg in the control diet) partially substituted by sodium butyrate (3 g/kg) in the experimental treatment.
§ Protastar® (AVEBE, Veendam, The Netherlands).
∥ Mixture of palm oil and soyabean oil (1:1, v/v).
¶ Supplying (per kg diet): retinol, 525 μg; cholecalciferol, 5 μg; α-tocopherol, 7·4 mg; phylloquinone, 0·5 mg; thiamin, 1 mg; riboflavin, 4 mg; d-pantothenic acid, 9 mg; niacin, 12·5 mg (available); biotin, 50 μg; cyanocobalamin, 15 μg; folic acid, 0·3 mg; pyridoxine, 1·5 mg; choline, 400 mg; Fe, 80 mg; Zn, 54 mg; Mn, 30 mg; Co, 0·15 mg; I, 0·14 mg; Se, 0·25 mg; antioxidants (E310, E320, R321), 50 mg; maize starch as a carrier.
** Used as an ileal digestibility marker.
†† Used as a faecal digestibility marker.
Measurements and sampling during the experiment
The piglets were weighed three times per week during the suckling period, the weaning day, twice per week from weaning to slaughter and, finally, on the slaughtering day. Fresh faeces were collected twice on day 37 and faecal samples were freeze-dried, milled and stored at +4°C until chemical analysis. Blood (10 ml) was collected from either of the jugular veins of piglets at day 5, at day 28 (weaning) and at day 37, in tubes with EDTA (VT-100 STK, 0·1 ml EDTA, 0·47 ml/l: 21 % (w/v); CML, Nemours, France) and aprotinin (10 000 IU/ml Trasylol; Bayer Pharma SAS, Puteaux, France). After blood centrifugation (2300 g; 10 min; +4°C), plasma was recovered and stored frozen at − 20°C until analysis.
Measurements and sampling at slaughter
Piglets from two litters were randomly slaughtered 4 h after the last meal on day 39 and those from the remaining two litters on day 40 after birth for the first batch of pigs. Slaughter took place on days 41 and 42 for the pigs of the second batch. The piglets were stunned by electric shock and then exsanguinated. After midline abdominal incision, the GIT was gently removed, weighed and placed on ice for digesta contents and tissue sampling. In brief, the stomach and the large intestine were separated from the SI. The stomach was weighed full and empty. The digesta were frozen and later freeze-dried, milled and stored at +4°C before chemical analysis.
The SI was separated from the mesentery, measured for its length and divided into five segments: the first one was from the pylorus to the ligament of Treitz (SI1) and corresponded to the duodenum. The distal 2 m were taken arbitrarily as the ileum (SI5) for getting enough digesta for ileal digestibility measurements. The remaining part of the SI corresponded (mostly) to the jejunum. It was divided into three parts equal in length (SI2, SI3, SI4). The total contents from the SI were collected frozen, freeze-dried, milled and stored dry at +4°C until analysis. The empty SI was flushed with cold saline, blotted dry with absorbing paper and the tissues weighed. The caecum and colon were not examined.
Chemical analysis of diets, gastric and intestinal digesta and faeces
DM was determined by drying at +105°C to constant weight, and ash by incineration at +550°C for 16 h. N was determined with a Leco FP 428 N analyser method (no. 990.03; Leco Corp., St Joseph, MI, USA) according to Association of Official Analytical Chemists(27). The concentration of chromium from chromic oxide in diets and digesta was determined spectrophotometrically (Cobas Mira; Horiba ABX, Montpellier, France) according to the method described by Poncet & Rayssiguier(Reference Poncet and Rayssiguier28). The concentration of titanium in diets and faeces was determined spectrophotometrically (Cobas Mira; Horiba ABX) according to the method described by Njaa(Reference Njaa29).
Histomorphometry
A small tissue sample (0·5 × 0·5 cm) was removed from the middle of each intestinal segment, fixed in buffered formalin and kept in ethanol–water (70:30, v/v) at +4°C until analysis. Villous–crypt architecture along the SI was determined using microdissection according to the technique of Goodlad et al. (Reference Goodlad, Levi and Lee30) and measured using image analysis as previously described(Reference Boudry, Peron and Le Huerou-Luron31). Mean values for villous height and crypt depth were determined for ten individual villi and crypts from each specimen, and individual means calculated. Mucosal thickness was estimated for each sample as the sum of mean villous height and mean crypt depth.
Intestinal histology for cell proliferation and apoptosis, and for densities of goblet cells and intra-epithelial lymphocytes
Whole tissue from the medium part of the SI (SI3) was collected quickly after slaughter, fixed in phosphate-buffered formalin (4 %), dehydrated and embedded in paraffin. Then, sections of 5 μm thickness were prepared and stained or immunostained for proliferation (PCNA antibody clone PC10; Sigma) and apoptosis (terminal deoxynucleotidyl transferase-mediated dUTP-biotin nick end-labelling (TUNEL); Promega, Madison, WI, USA) as described by Domeneghini et al. (Reference Domeneghini, Di Giancamillo and Bosi32). Other sections were stained with haematoxylin and eosin and counted for goblet cells and intra-epithelial lymphocytes in the villi. Staining was carried out on two serial sections each time and cell counts were conducted on between ten and twenty villi and crypts as previously reported(Reference Piel, Montagne and Sève33). The means were calculated for getting one average value per cell type and per pig.
Digestive enzyme activities
Just after slaughtering, a piece of pancreatic tissue was frozen in liquid N2 and stored at − 20°C. From the middle of each of the five SI segments (SI1 to SI5), a piece of mucosa was scraped with a glass slide from a portion (40 cm) of SI. The mucosa was weighed and homogenised. Samples were then frozen in liquid N2 and stored at − 20°C. After thawing of the samples coming from pancreas tissue and intestinal mucosa, protein content was measured as described by Lowry et al. (Reference Lowry, Rosebrough and Farr34). The digestive enzymes were analysed in the pancreas and in the small-intestinal mucosa as reported elsewhere(Reference Gestin, Le Huërou-Luron and Péniau35–Reference Marion, Petersen and Romé37) and are presented in Table 2. Enzyme activities were calculated as μmol hydrolysed substrate per min (IU) and expressed as specific (IU per g protein) or total (IU/kg piglet BW) activity.
Table 2 Pancreatic and intestinal enzymes analysed

Detection of pro-inflammatory cytokine mRNA
RNA from three segments (SI1, SI3 and SI5) was extracted as previously described(Reference Meissonnier, Pinton and Laffitte38). After assessment of concentration, purity and quality, 2 μg total RNA were reverse-transcribed(Reference Oswald, Dozois and Barlagne39). Real-time PCR assays were performed on 8 ng synthesised cDNA(Reference Meissonnier, Pinton and Laffitte38), with porcine-specific primers for cyclophilin A, IL-1β, IL-18 and TNFα (Table 3)(Reference Meissonnier, Pinton and Laffitte38, Reference Von der Hardt, Kandler and Fink40, Reference Royaee, Husmann and Dawson41). Special care was taken to design or choose sets of primers on different exons or spanning exon–exon conjunctions to differentiate cDNA from genomic DNA. A dissociation curve analysis was carried out to confirm the specificity of the target nucleic acid sequence amplified. Data were analysed using the method of Peirson et al. (Reference Peirson, Butler and Foster42) and individual values for cytokine expression were obtained after normalisation to cyclophilin A, a housekeeping gene used for relative quantification. The level of expression of genes encoding for cytokines is expressed in fold-change as compared with CC piglets (mean value of 1 in this group).
Table 3 Oligonucleotide sequences of forward and reverse primers used for real-time PCR

IGF, insulin-like growth factor.
Detection of insulin-like growth factor-I and insulin-like growth factor-I receptor mRNA
From a tissue sample collected in the middle of the SI (SI3), mRNA was extracted as previously described(Reference Marion, Petersen and Romé37). Total mRNA (3 μg) was reverse-transcribed(Reference Gardan, Gondret and Louveau43). Real-time PCR assays were performed on 50 ng synthesised cDNA(Reference Gardan, Gondret and Louveau43), with porcine-specific primers for insulin-like growth factor I (IGF-I) and IGF-I receptor (Table 3). Endogenous 18S ribosomal RNA amplifications were used for each sample to normalise the expression of the selected genes.
Plasma gut regulatory peptide concentrations
Plasma concentrations of cholecystokinin were measured in ethanol-extracted plasma by means of a commercially available RIA kit (reference RB 302; Euro-Diagnostica AB, Malmo, Sweden). Synthetic cholecystokinin 26-33 was used as a standard. The concentration of glucagon-like peptide-2 (GLP-2) in plasma was measured by RIA after extraction of plasma with 70 % ethanol (v/v, final concentration), as previously reported(Reference Hartmann, Johnsen and Ørskov44). The antiserum is directed against the common N-terminus of human and porcine GLP-2 and therefore measures only fully processed GLP-2 of intestinal origin.
Calculations and statistical analyses
The apparent digestibility coefficients of DM, organic matter and N at the ileal level and in the whole GIT were calculated according to the following equations(Reference Wilfart, Montagne and Simmins26):


where Zileum, Zfaeces and Zdiet are the DM, organic matter or N concentrations in ileum contents, faeces and in diet, respectively, Crileum and Crdiet are the concentrations of chromium in ileum contents and diets and Tifaeces and Tidiet are the concentrations of titanium in faeces and diet, respectively.
An ANOVA of the data was conducted using the general linear model procedure of SAS (version 8.1; SAS Institute Inc., Cary, NC, USA) with a two-levels full factorial design including SB administration before weaning (Be), SB administration after weaning (Af) and Be × Af interaction. Batch and litter within batch were also included in the model. The values presented are least square mean values with their standard errors and effects were considered significant at P < 0·05. Tendencies were reported when 0·05 < P ≤ 0·10. When the Be × Af interaction had a value of P ≤ 0·10, the following three pre-planned orthogonal contrasts were analysed: ‘SB all periods v. never SB’ ((CB+BC+BB) v. CC); ‘SB, one period v. two periods’ ((CB+BC) v. BB); ‘SB only before weaning v. only after weaning’ (BC v. CB). The values for cytokine gene expressions did not display a normal residue distribution. Therefore, a log base 10 transformation of the data was used.
Results
The experiment went well but two pigs (one in the BC and one in the BB group) had to be removed from the trial because they did not consume the weaning diets. The other animals remained clinically healthy during the entire study.
Growth performance, digestibility of feed components and transit along the gastrointestinal tract
Supplementing the pigs with SB before weaning had a positive effect on their final BW (P = 0·042) (Table 4). Before weaning, average daily gain did not differ between the four groups (P = 0·25 to P = 0·53), but after weaning, average daily gain was higher in pigs receiving SB (BC, CB and BB pigs) than in control pigs (P = 0·016 and P = 0·10 for SB supplied before and after weaning, respectively). The average daily feed intake in the post-weaning period was higher for the pigs consuming SB before weaning (P = 0·048).
Table 4 Influence of oral supplementation with sodium butyrate (SB) before (Be) and/or after (Af) weaning on body weight, performance and post-weaning feed intake in pigs
(Mean values with residual standard errors of analysis of variance)

CC, control (no SB supplementation); BC, SB before weaning and control after weaning; CB, control before weaning and SB after weaning; BB, SB before and after weaning.
* Be × Af: interaction between SB supplementation before and after weaning.
† Gain:feed ratio.
When SB was provided after weaning (Table 5), SB treatment tended to decrease post-weaning apparent ileal DM digestibility (P = 0·076) and decreased the apparent digestibility of N (P = 0·009). The faecal apparent digestibility of DM, organic matter and N was increased with SB supplementation before weaning (P = 0·030 to P = 0·040) while it was decreased when SB was provided after weaning (P = 0·004 to P = 0·015). Finally, the post-weaning faecal apparent digestibility of ash was lower in pigs supplemented with SB before weaning (P = 0·032) but was not influenced when SB was provided after weaning (P = 0·60).
Table 5 Influence of oral supplementation with sodium butyrate (SB) before (Be) and/or after (Af) weaning on apparent ileal and faecal digestibility of feed components after weaning in pigs
(Mean values with residual standard errors of analysis of variance)
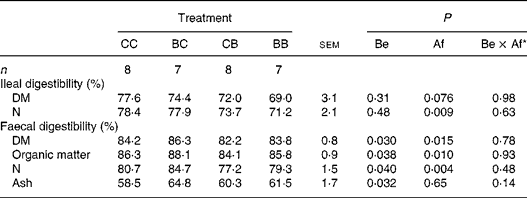
CC, control (no SB supplementation); BC, SB before weaning and control after weaning; CB, control before weaning and SB after weaning; BB, SB before and after weaning.
* Be × Af: interaction between SB supplementation before and after weaning.
At 4 h after the last meal, the amount of DM (expressed as a percentage of DM intake at the last meal) left in the stomach was higher in piglets receiving SB before weaning (P = 0·034), with no effect of SB administration after weaning (P = 0·14) (Fig. 1). This was confirmed for residual N in the stomach (data not shown). The amount of DM present in each of the first four segments of the SI (SI1 to SI4) was not influenced by SB supplementation (P = 0·25 to P = 0·91). SB supplementation had no effect on residual DM (or N; data not shown) present in the distal SI (SI5) (DM: P = 0·43; N: P = 0·55). However, there was a tendency (P = 0·09) for an interaction between SB supplementation before and after weaning. The amount of DM (and N; data not shown) in the distal SI (SI5) of pigs supplemented with SB strictly before or after weaning tended to be lower than in pigs supplemented at both periods (P = 0·073). The treatment had no effect on digesta pH along the GIT (data not shown).
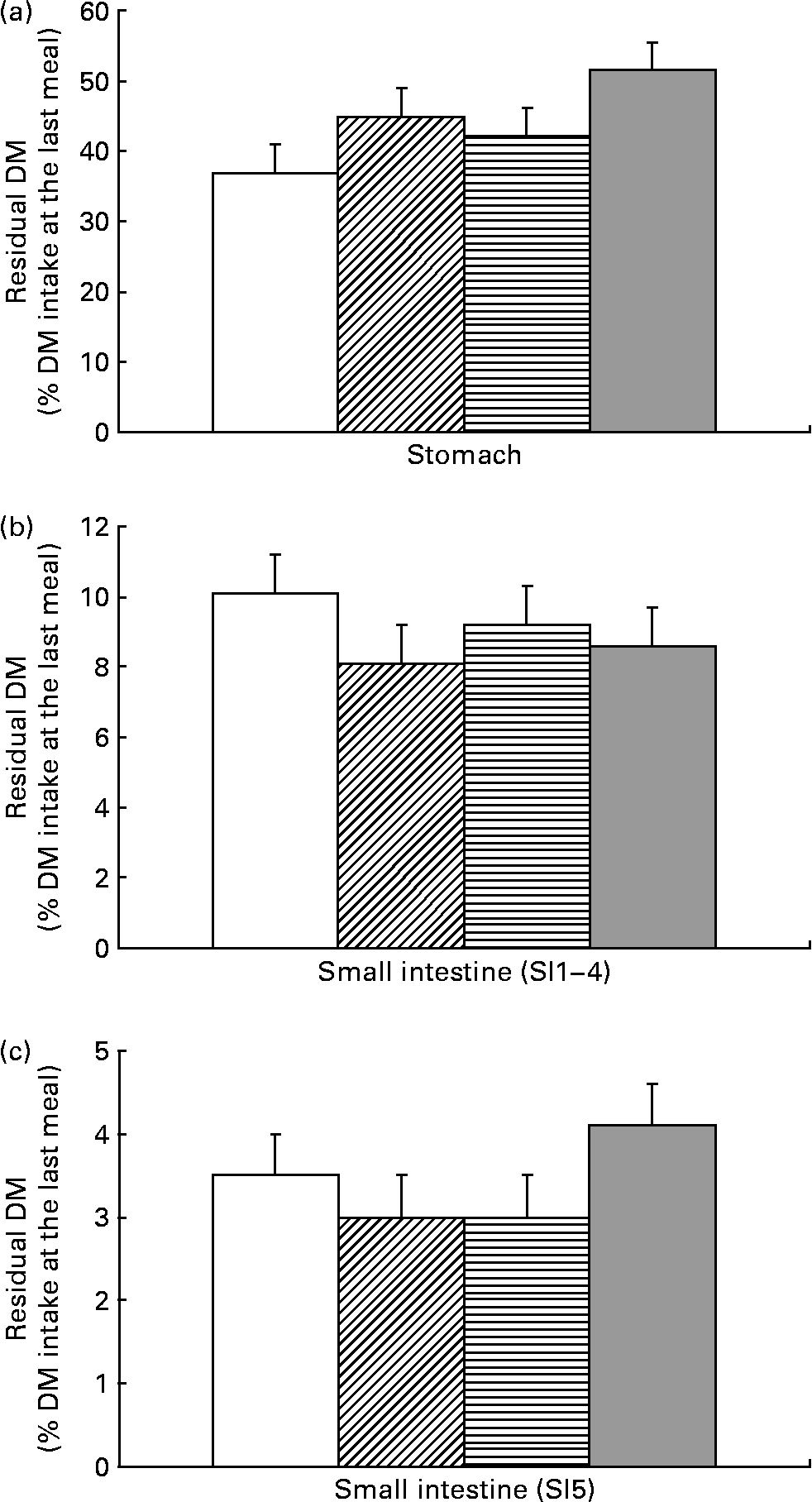
Fig. 1 Influence of sodium butyrate (SB) supplementation on residual digesta DM in the stomach (a), the proximal and medial small intestine (SI1 to SI4) (b) and the distal small intestine (SI5) (c) after slaughter 4 h after the last meal. (□), Control (no SB supplementation before or after weaning); (), SB before weaning and control after weaning (BC); (
), control before weaning and SB after weaning (CB); (
), SB before and after weaning (BB). Values are means (n 7–8 per group), with standard errors of the mean represented by vertical bars. Supplementation with SB before weaning increased the residual DM present in the stomach (P = 0·034). The treatments did not affect digesta DM present in the proximal plus mid-small intestine (SI1 to SI4) (P>0·10). The before × after supplementation interaction tended (P = 0·09) to be significant for the residual DM present in the distal small intestine. The pre-planned orthogonal contrast comparisons revealed a tendency (P = 0·07) for residual DM in the distal small intestine to be lower in the pigs supplemented with SB (for one period; BC or CB) as compared with those supplemented for both periods (BB).
Weight of the stomach and the small intestine, villous and crypt architecture and digestive enzyme activities of the small intestine
The fresh stomach weight was not influenced by SB supplementation (data not shown). By contrast, the SI weight was lower in the pigs supplemented with SB before weaning (P = 0·013), with no effect of SB supplementation after weaning (P = 0·66) (Table 6). This effect was (or tended to be) significant for all SI segments (P = 0·01 to P = 0·06), except the proximal (SI1) segment (P = 0·25 by SB supplementation; data not shown). SB provided before weaning to the pigs mainly affected the mucosa, the fresh weight of which was reduced for segments 2–4 of the SI (P ≤ 0·02). The weight of the SI muscle layers was not influenced by SB supplementation (P = 0·17 to P = 0·94).
Table 6 Weight of the whole tissue, mucosa and muscularis of the small intestine (SI)
(Mean values with residual standard errors of analysis of variance)
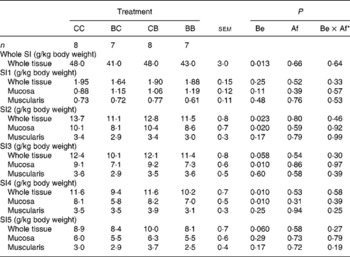
CC, control (no SB supplementation); BC, SB before weaning and control after weaning; CB, control before weaning and SB after weaning; BB, SB before and after weaning; Be, before weaning; Af, after weaning.
* Be × Af: interaction between SB supplementation before and after weaning.
Villous–crypt architecture analysis of the mucosa revealed no effect of SB supplementation in the proximal (SI1) and medial (SI3) segments (P = 0·14 to P = 0·97) (data not shown). However, the interaction of SB supplementation between before and after weaning was significant for villous height and mucosal thickness in the distal SI (SI5) (P = 0·03 and P = 0·05, respectively) (Fig. 2). Pigs supplemented with SB for one period (CB or BC groups) had villous height and mucosal thickness that were lower than those supplemented for both periods (BB) (P = 0·05 and P = 0·03, respectively). Cell proliferation as assessed in the mid-SI (SI3) tended to be increased by oral supplementation of SB after weaning (mean across CC and BC = 35·7 v. CB and BB = 39·6 (sem 1·6) cells per crypt; P = 0·10). Apoptosis was not influenced by the treatments (data not shown).
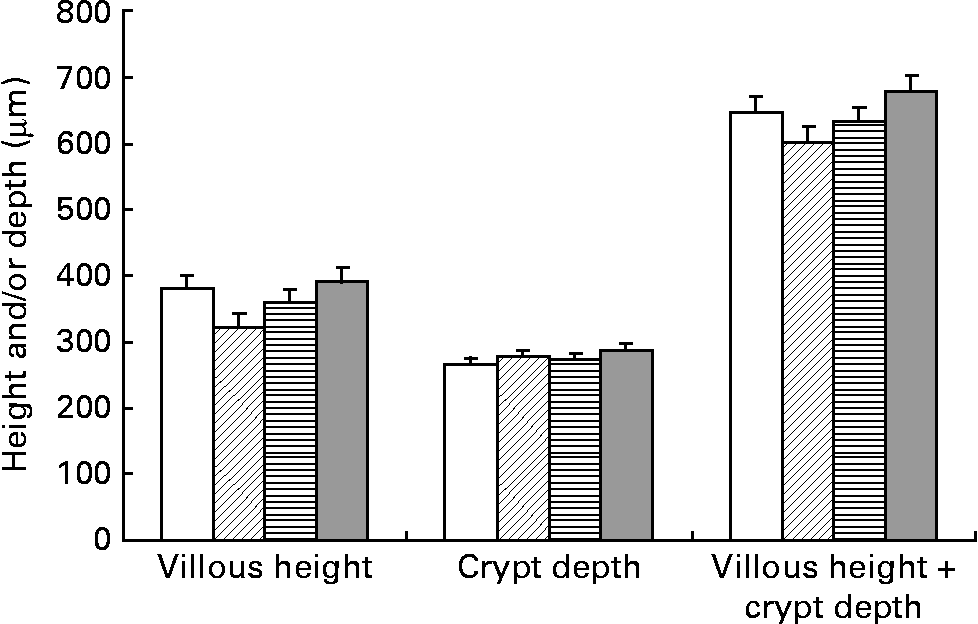
Fig. 2 Influence of sodium butyrate (SB) supplementation on villous height, crypt depth and villous height plus crypt depth of the distal small intestine (SI5). (□), Control (no SB supplementation before or after weaning); (), SB before weaning and control after weaning (BC); (
), control before weaning and SB after weaning (CB); (
), SB before and after weaning (BB). Values are means (n 7–8 per group), with standard errors of the mean represented by vertical bars. Supplementation with SB before or after weaning was not significant (P>0·10) but the before × after supplementation interaction was significant for villous height (P = 0·03) and villous height plus crypt depth (P = 0·05). The pre-planned orthogonal contrast comparisons indicated lower villous height and mucosal thickness in the pigs supplemented with SB for one period (BC or CB) as compared with those supplemented for both periods (BB) (P = 0·05 and P = 0·03, respectively). Crypt depth was influenced neither by the factors tested nor by their interaction (P = 0·18 to P = 0·89).
The density of total and subtype goblet cells (neutral, acidic and acidic sulfated mucin-containing cells) and intra-epithelial lymphocytes in the mid SI were not influenced.
The total activities of maltase, sucrase, aminopeptidase A, aminopeptidase N and dipeptidyl peptidase IV, expressed per kg BW, were lower in the SI of pigs supplemented with SB after weaning (Table 7). The activity of lactase was not influenced by SB supplementation. The activity of maltase tended to be lower (P = 0·08) and that of sucrase was lower when pigs were supplemented with SB (whatever the period of supplementation; P = 0·01). However, the specific activities (IU/g protein) of all these enzymes were not influenced by SB supplementation (data not shown).
Table 7 Influence of oral supplementation with sodium butyrate (SB) before (Be) and/or after (Af) weaning on intestinal enzyme activities in pigs*
(Mean values with residual standard errors of analysis of variance)
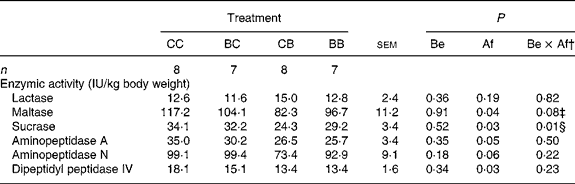
CC, control (no SB supplementation); BC, SB before weaning and control after weaning; CB, control before weaning and SB after weaning; BB, SB before and after weaning.
* Values are means of the enzymic activities determined in each intestinal segment. 1 IU = 1 μmol hydrolysed substrate per min.
† Be × Af: interaction between SB supplementation before and after weaning.
‡ The pre-planned orthogonal contrast comparisons revealed lower maltase activity in pigs supplemented with SB (whatever the period) as compared with controls (P = 0·001) and in pigs supplemented with SB after weaning as compared with those supplemented with SB before weaning (P = 0·044).
§ The pre-planned orthogonal contrast comparisons revealed lower sucrase activity in pigs supplemented with SB (whatever the period) as compared with controls (P = 0·008) and in pigs supplemented with SB after weaning as compared with those supplemented with SB before weaning (P = 0·011).
Pancreas weight and pancreatic digestive enzyme activities
SB supplementation influenced neither the weight nor the protein content of the pancreas (data not shown). The total chymotrypsin activity was reduced (P = 0·05) when SB supplementation was provided before weaning, with no effect of supplementation after weaning (P = 0·14) (Fig. 3). The total activities of trypsin and elastase II tended to be reduced by SB supplementation after weaning and before weaning, respectively (P = 0·10). The total activities of amylase, elastase I and lipase were not influenced by SB supplementation (data not shown). The specific activities of all these enzymes were not influenced by the dietary treatments (data not shown).
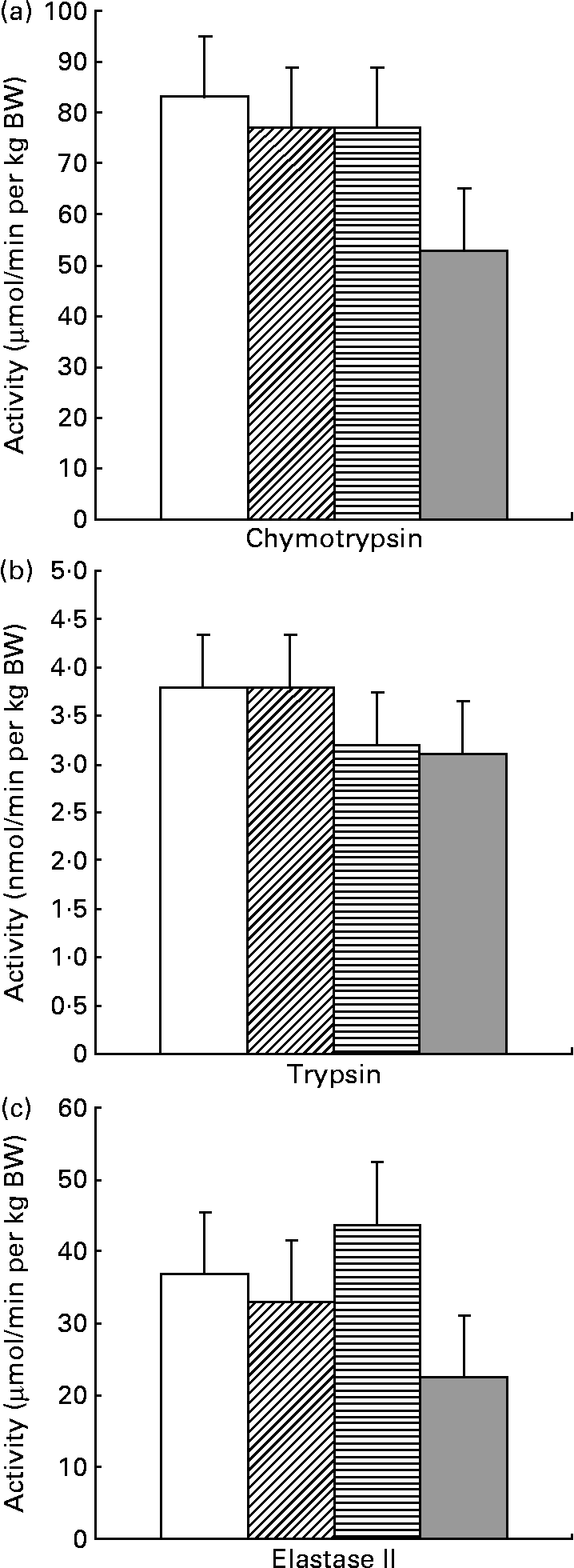
Fig. 3 Influence of sodium butyrate (SB) supplementation on chymotrypsin (a), trypsin (b) and elastase II (c) activities of the pancreas. (□), Control (no SB supplementation before or after weaning); (), SB before weaning and control after weaning; (
), control before weaning and SB after weaning; (
), SB before and after weaning. Values are means (n 7–8 per group), with standard errors of the mean represented by vertical bars. Chymotrypsin activity was reduced by SB supplementation before weaning (P = 0·05). Trypsin activity tended to be reduced by SB supplementation after weaning (P = 0·10). Elastase II activity tended to be reduced by SB supplementation before weaning (P = 0·10). BW, body weight.
Plasma gut regulatory peptide concentrations and insulin-like growth factor-I and insulin-like growth factor-I receptor gene expression
SB supplementation had no effect on plasma cholecystokinin and GLP-2 concentrations (data not shown).
SB supplementation neither influenced the IGF-I nor IGF-I receptor expression in the mid-SI (SI3) of piglets (data not shown).
Cytokine gene expression profile
In the proximal segment (SI1) and distal segment (SI5), all examined cytokines showed similar levels of expression whatever the treatment (data not shown). However, for IL-18 in the mid-segment (SI3), there was a tendency for an interaction between treatments before and after the weaning (mean per group expressed in fold-change as compared with the CC group: for BC, 0·85; for CB, 0·81; for BB, 1·53 (sem 0·26); P = 0·06). IL-18 was less expressed (P = 0·03) in the mid-SI (SI3) of pigs supplemented with SB for one period (whatever the period of supplementation) as compared with the control group (CC) and with the SB supplemented before and after weaning (BB group).
Discussion
In the present investigation, we provide evidence that SB, especially when provided early after birth, had positive effects on growth performance and on feed intake in young pigs. The new findings are that the effects of SB involved delayed gastric emptying, reduced intestinal mucosa weight and enhanced feed digestibility. Compared with data available in the literature, our work is the first to study the long-term impact, i.e. after weaning, of an oral butyrate administration during the neonatal period in piglets.
Digestion
Many mineral and organic acids, including SB, are known to slow down gastric emptying(Reference Hunt and Knox45–Reference Manzanilla, Perez and Martin47) but the underlying mechanisms are still poorly understood(Reference Manzanilla, Perez and Martin47). In agreement with these results, we observed an important gastric retention of DM when pigs were supplemented with SB before weaning. However, post-weaning SB supplementation does not seem to influence DM contents in the stomach of pigs(Reference Manzanilla, Nofrarias and Anguita19), as confirmed in the present study. We have no clear explanation for the observed impact of pre-weaning SB on gastric retention post-weaning, but the present data suggest a long-lasting action of SB on the developmental physiology of the stomach. Pre-weaning supplementation of SB was found to increase the densities of gastric parietal cells and somatostatin-positive cells in the present study as previously reported(Reference Mazzoni, Le Gall and De Filippi23). However, an opposite effect on the number of both parietal and endocrine cells was seen with calcium formate, another salt of organic acid, used at a similar dose(Reference Bosi, Mazzoni and De Filippi48). Similar to our observation, Manzanilla et al. (Reference Manzanilla, Perez and Martin47) reported increased stomach DM content in weaned pigs supplemented with formic acid or a mixture of plant extracts. They hypothesised that an increased gastric retention, as observed in the present study with SB, may be beneficial to the pig due to the importance of gastric pre-digestion of the diet and the known role of the stomach in preventing the entry of pathogens into the GIT.
One interesting point of the present study is that SB fed to pigs during the suckling period had no influence (ileal level) or improved (faeces) the apparent digestibility of feed components after weaning. By contrast, SB provided after weaning strongly decreased the ileal digestibility of both DM and N ( − 5·5 percentage points), with less marked effects at the faecal level. To our knowledge, no data are available in the literature on the effect of pre-weaning SB supplementation on digestibility after weaning. The present results are partly in agreement with those of Manzanilla et al. (Reference Manzanilla, Nofrarias and Anguita19) who reported reduced ileal digestibility of starch in pigs supplemented with SB after weaning. Our data suggest that supplementing pigs with SB before weaning influenced GIT microbiota colonisation in such a way that fermentation was more efficient after weaning. Although the underlying mechanisms are not understood yet, Castillo et al. (Reference Castillo, Martin-Orue and Roca49) reported that oral SB supplementation promoted the largest changes in the composition of the jejunal microbiota and in the ecological structure and metabolic activity of the microbial ecosystem of the large intestine, in comparison with the control treatment. These authors suggested that such changes in the extent of digestion and absorption of nutrients in the upper GIT could potentially modify the fermentable material reaching the large intestine and, therefore, modify the microbial activity at the faecal level. Work is in progress to explore the effects of SB supplementation on the microbiota.
Changes in ileal digestibility due to SB supplementation as observed in the present study are in agreement with the decreases (or tendencies) in the enzyme activities. Since the activity of pancreatic chymotrypsin and trypsin, and that of the intestinal mucosa, were lower in pigs supplemented with SB after weaning, it may be assumed that the digestion was lower. In contrast with the present results, acute administration of SB was shown to stimulate pancreatic fluid and amylase secretion dose-dependently in various animal models in vivo and ex vivo (Reference Katoh and Tsuda50, Reference Ohbo, Katoh and Sasaki51). In pigs, intra-ileal administration of SB caused an acute increase of trypsin and protein outputs at low doses (5 mm), with no effects at higher doses (10 mm)(Reference Sileikiene, Mosenthin and Tafaj52).
Intestinal mucosa weight, villous–crypt architecture and cellularity and cytokine gene expression
Oral SB supplementation reduced the SI mucosal mass. This recalls the reported effect of dietary plasma protein on this organ(Reference Jiang, Chang and Stoll53). The fact that SB reduced SI mucosal weight would suggest that SB may interfere with intestinal protein metabolism(Reference Jiang, Chang and Stoll53, Reference Pirman, Mosoni and Remond54), following a reduced bacterial load and possibly a lower inflammation locally. Indeed, SB is known for its antibacterial(Reference Sun, O'Connor and Turner55) and anti-inflammatory(Reference Weber and Kerr9, Reference Segain, Raingeard de la Blètiere and Bourreille10) properties. Butyrate was shown to decrease intestinal expression levels of TNFα, IL-1β and IL-6 in patients with Crohn's disease(Reference Weber and Kerr9). In the present experiment, only the expression of the gene encoding for IL-18 tended to be lower in piglets fed SB either before or after weaning in the jejunum. A transient gut inflammation has been reported in piglets a few days after weaning(Reference McCracken, Spurlock and Roos56, Reference Pié, Lallès and Blazy57). In the present study, the observations made fairly late (12 d) after weaning may explain the low changes induced by SB in inflammatory cytokine gene expression. Besides, oral SB is quickly absorbed in rodents(Reference Egorin, Yuan and Sentz58) and disappears before the lower SI in pigs(Reference Manzanilla, Nofrarias and Anguita19), which makes a direct luminal effect of SB on the SI unlikely.
Manzanilla et al. (Reference Manzanilla, Nofrarias and Anguita19) reported a large increase (+40 %) in jejunal crypt depth of SB-supplemented weaned pigs aged 40 d, with no effect on the crypts of the other intestinal segments or on the SI villi. In the present study, we noted only a moderate reduction in jejunal villous height in SB-supplemented pigs, regardless of the period of supplementation. This observation is consistent with that of Biagi et al. (Reference Biagi, Piva and Moschini20) who reported a lack of effect of SB on villous–crypt architecture of weaned pigs, aged 60 d, supplemented with varying doses of SB (from 0·1 to 0·4 % of DM intake).
In the study by Manzanilla et al. (Reference Manzanilla, Nofrarias and Anguita19), SB ingestion led to a doubled density of goblet cells in the colon but failed to influence goblet cells in other parts of the GIT, enterocyte proliferation or intra-epithelial lymphocytes. In the present study, we noted a tendency for increased proliferation of enterocytes in villi of the mid-jejunum of pig supplemented with SB after weaning only. This agrees with earlier investigations demonstrating the proliferative properties of SB on the healthy GIT in vivo (Reference Kien, Schmitz-Brown and Solley15, Reference Sakata59). However, this effect may depend on the dose of n-butyric acid, low doses being stimulatory while higher doses inhibiting cell proliferation(Reference Inagaki and Sakata60).
Manzanilla et al. (Reference Manzanilla, Nofrarias and Anguita19) suggested that the increase in body average daily gain:average daily feed intake ratio found in pigs fed SB may be due to activation of the IGF-I axis because insulin-like growth factors exert multiple physiological functions including tissue growth and cell differentiation and survival(Reference Stewart and Rotwein61). The present data do not support this hypothesis.
Feed intake and growth
The preceding observations that oral SB administered to pigs during the suckling period increased gastric retention of digesta, reduced intestinal mucosa weight and finally maintained or improved the apparent digestibility of starter feed components after weaning may probably contribute to explain the better growth rate and post-weaning feed intake as observed in the present study.
Studies with SB supplementation have provided conflicting results regarding growth performance, feed intake and feed efficiency. Postnatal supplementation of 3 g SB/kg milk replacer increased BW gain in neonatal pigs fed a milk replacer(Reference Kotunia, Woliński and Laubitz21). In weaned pigs, a dose of 3 g SB/kg was reported to increase feed efficiency without altering feed intake(Reference Manzanilla, Nofrarias and Anguita19). By contrast, increasing levels of oral SB in the diet had no effect on growth performance of weaned pigs(Reference Biagi, Piva and Moschini20, Reference Weber and Kerr62). In the latter study(Reference Weber and Kerr62) a negative relationship between BW gain or feed intake and the dose of SB (0 to 4 g/kg) could be established, contrary to previous investigations(Reference Biagi, Piva and Moschini20). Many differences exist between these studies, including the period of supplementation, the dose of SB, the feed composition (pre- and post-weaning), the breed of pigs and finally the experimental environment. Thus, it is currently difficult to draw clear conclusions.
The present investigations suggest that SB provided before weaning has an effect on gastric retention and intestinal mucosa thinning that is more marked when the supplementation occurs before weaning, the final result being a more positive impact on growth rate with pre-weaning administration of SB. The opposite is true for feed component digestibility: it was reduced by post-weaning supplementation with SB. Combining SB supplementation before and after weaning seems to have contrasted effects on these GIT indices. Gastric retention of digesta tended to be more marked, intestinal mucosa thinning less pronounced and digestibility more reduced than with SB provided after weaning alone. This situation seemed to be more favourable to starter feed intake as compared with SB supplementation post-weaning only. Thus, the modes of action of oral SB are suggestively complex and the outcomes difficult to predict.
In conclusion, oral butyrate supplementation stimulates growth performance and starter feed intake in young pigs, especially when provided early after birth. This is accounted for by delayed gastric emptying, reduced intestinal mucosa weight and increased feed component digestibility. Further work is needed to elucidate the effects of oral SB on microbial colonisation, protein metabolism and barrier properties in the SI in order to understand the modes of action and the functional outcomes in terms of its effects on intestinal protection.
Acknowledgements
The authors thank R. Vilboux and G. Guillemois for diet manufacturing, D. Boutin, L. Gaillard, C. Homo, Y. Surel and P. Touanel for the care of the animals, and M. Formal, L. Lenormand, C. Perrier, V. Romé, G. Savary, S. Chever, M. Leborgne and A. Sahar for technical assistance. Professor C. Domeneghini is acknowledged for her advice in conducting proliferation and apoptosis histology. The financial support of the European Union for the project ‘Feed for Pig Health’ (contract number FOOD-CT-2004-506144) is greatly acknowledged. The authors are solely responsible for the work described in this article, and their opinions are not necessarily those of the European Union.
J.-P. L. and P. G. were responsible for the project development. M. L. G. conducted the nutritional experiments in pigs. M. L. G., J.-P. L. and P. G. were responsible for the analyses. I. P. O. and M. G. were responsible for cytokine analyses. J. J. H. was responsible for the determination of the plasma GLP-2 concentration. I. L. was responsible for the determination of the expression of IGF-I and its receptor. B. S. and M. L. G. were responsible for generating statistical analyses. M. L. G., and J.-P. L. were responsible for drafting the manuscript and M. L. G. is the corresponding author.
There are no conflicts of interest.