Introduction
Common milkweed (Asclepias syriaca L.) is known to provide a wide range of ecological benefits. The most well-known ecological function of Asclepias spp. is that they are the required larval host plant for the monarch butterfly (Danaus plexippus) (Ackery and Vane-Wright Reference Ackery and Vane-Wright1984). Asclepias spp. are associated with a wide variety of insects beyond monarch butterflies, including some species that have been shown to be beneficial in controlling pest insects such as the European corn borer (Ostrinia nubilalis) (DiTommaso et al. Reference DiTommaso, Averill, Hoffmann, Fuchsberg and Losey2016). Conservationists have shown significant interest in planting A. syriaca to restore monarch butterfly habitats, and farmers may be interested in planting A. syriaca at low densities on agricultural land as part of an integrated pest management approach (Harris et al. Reference Harris, Hall and Finke2023; Mohler et al. Reference Mohler, Teasdale and DiTommaso2021).
The most common method of establishing A. syriaca populations for habitat restoration is by planting it from seed. However, this can be a time-consuming process due to stratification requirements, so vegetative propagation may be a desirable alternative (Luna Reference Luna, Dumroese, Luna and Landis2009). Asclepias syriaca is particularly suited to vegetative propagation, because it readily reproduces from lateral root buds. It spreads expansively, which has led to invasiveness outside its native range (Pauková et al. Reference Pauková, Káderová and Bakay2013).
The roots of A. syriaca survive for multiple years, and adventitious buds sprout in the spring when temperatures rise (Bhowmik and Bandeen Reference Bhowmik and Bandeen1976). In other weed species that propagate similarly from the roots, such as field bindweed (Convolvulus arvensis L.), vegetative propagation is the most common method of reproduction for certain populations (Gaskin et al. Reference Gaskin, Cortat and West2023). Roots have larger resource reserves than seeds, and plants arising from roots can be more competitive than those arising from seeds (Omezine and Harzallah-Skhiri Reference Omezine and Harzallah-Skhiri2010).
Most clonal propagation occurs from lateral root buds, which form on portions of the root system located mainly at shallow soil depths (Polowick and Raju Reference Polowick and Raju1982). However, propagation in A. syriaca can occur even from root segments without buds, and emergence can occur from as deep as 107 cm in the soil (Evetts and Burnside Reference Evetts and Burnside1974). Research conducted on butterfly milkweed (Asclepias tuberosa L.), a species closely related to A. syriaca, found that vegetative propagation was most successful when segments from portions of the root closer to the surface were used and that planting depth did not have much of an impact on plant growth (Ecker and Barzilay Reference Ecker and Barzilay1993). Propagation has been successful even from short root segments, with previous research indicating that the minimum length at which clonal reproduction can occur is 2.5 cm (Jeffery and Robinson Reference Jeffery and Robison1971).
Currently, the recommendations for vegetatively propagating A. syriaca are to collect segments from dormant plants, with segments taken from higher up on the roots and having at least one bud (Luna and Dumroese Reference Luna and Dumroese2013). It is also advisable to plant root segments by late fall and irrigate them in the first year (Stevens Reference Stevens2000). The objective of our research was to elaborate on these guidelines by determining an optimal root segment length and burial depth for the vegetative propagation of A. syriaca. We hypothesized that vegetative propagation from longer root segments will be more successful (i.e., increased emergence and growth) than propagation from shorter root segments and that root segment burial closer to the surface but within the top 30 cm of soil will result in more successful propagation, relative to root burial farther from the soil surface. The rationale for these hypotheses is that longer root segments have more stored resources than shorter segments and that burial closer to the surface reduces the distance plants need to cover before they emerge, resulting in lower energy expenditures before plants reach the soil surface.
Materials and Methods
Two runs of a greenhouse study were conducted in 2023 at Cornell University in Ithaca, NY, USA. The first run took place from February 2 to June 7, and the second run took place from July 3 to November 10. Asclepias syriaca was grown from seed (Ernst Conservation Seeds, Meadville, PA, USA) in 9.5-L pots filled with Cornell Mix (0.2 m3 peat, 0.2 m3 vermiculite, and 0.1 m3 perlite plus 1.8 kg CaSO4, 1.8 kg 10-5-10 N-P-K, and 2.3 kg lime). Seeds were cold moist stratified at −2 C for a month before planting, and germination occurred after approximately 2 wk. Four seeds were planted in each pot. Plants were grown under 400-W high-pressure sodium lamps on a 15/9-h day/night cycle in addition to natural light. In the first run, 60 pots were planted in this phase of the trial, and approximately 40 seedlings emerged and survived. In the second run, 45 pots were planted, and 17 seedlings emerged and survived. The temperature was kept between 20 and 25 C during the day and between 18 and 23 C at night. Pots were irrigated with tap water as needed daily or whenever the top few centimeters of soil felt dry.
Roots were collected 6 wk after emergence, when the plants were an average of 21-cm tall. Plants were cut at the base of the stem before the root systems were removed from the pots, washed, and randomly arranged on a greenhouse bench. We cut segments 3-, 8-, or 15-cm long from this pool of root systems, taking care to perform this sampling without bias (examples of possible biases: taking 3-cm segments from smaller plants or from nearer the soil surface). All root segments were taken preferentially from the upper portions of the main root. Root segments of each length (3, 8, or 15 cm) were planted at a depth of 3, 8, or 15 cm, for a total of nine different treatment combinations. One root segment was planted per pot. There were five replicate pots per combination arranged in a completely randomized design. The pots were not rearranged during the experiment, meaning that plant growth might have been impacted by location on the bench. Pot size, soil, and growing conditions were as described earlier.
At the end of a 10-wk growing period, emergence from root segments was recorded, and the height of each plant was measured. All of the plants that emerged in both runs of this experiment established successfully, so the number of established plants reflects total emergence by the end of the experiment. Height measurements were taken at the natural highest point on the plant without straightening it. Plants were clipped at the soil surface to measure aboveground biomass. The soil was then removed from the pots, and the root systems were carefully removed before being rinsed with water over a sieve. Biomass samples were dried for 48 h at 55 C, sufficient to reach constant weight. One of the root samples in the first run of the experiment was damaged due to an error during the root-washing process, so that sample was removed from the data.
Data were analyzed in R (4.3.2; R Core Team 2023). Linear models were created using planting depth, root segment length, run of the experiment (first or second), and interactions as fixed effects. Models tested the impacts of these factors on above- and belowground biomass, height, and root:shoot ratio at the end of the 10-wk growing period. These models were evaluated using ANOVA. Square-root transformations were applied to all the models except for the root:shoot ratio models, which used logarithmic transformations. For all the biomass and height models, individual runs, but not the interaction between the run or either of the other factors, affected the results. In these cases, individual models were created for each run, using planting depth and root segment length as fixed effects, and were again evaluated using ANOVA. A generalized linear model was created to analyze the emergence data, using planting depth, root segment length, run of the experiment (first or second), and interactions as fixed effects. This model was also evaluated using ANOVA. For all the models, pairwise differences between treatments were evaluated with Tukey’s honest significant differences (HSD) test (α = 0.05). The estimated marginal means were used to generate figures.
Results and Discussion
The probability of emergence from root segments did not differ between runs of the experiment, and it was not affected by root segment length, planting depth, or the interaction between the two (Figure 1). However, the three treatments with the numerically lowest model-predicted likelihood of emergence were the 3-cm segments planted at depths of 15 or 8 cm (probability of emergence of 51% or 56%, respectively) and the 8-cm segments planted at a depth of 15 cm (probability of emergence of 56%; Figure 1). Plants grown from 15-cm segments planted at a 3-cm depth had the greatest chance of emergence, with a probability of 92% (Figure 1). This pattern in the emergence data supports the view that emergence from greater depths in the soil is more stressful for plants grown from smaller root segments. When a conservationist decides to use shorter root segments, it may be advantageous to plant them at soil depths less than 15 cm.
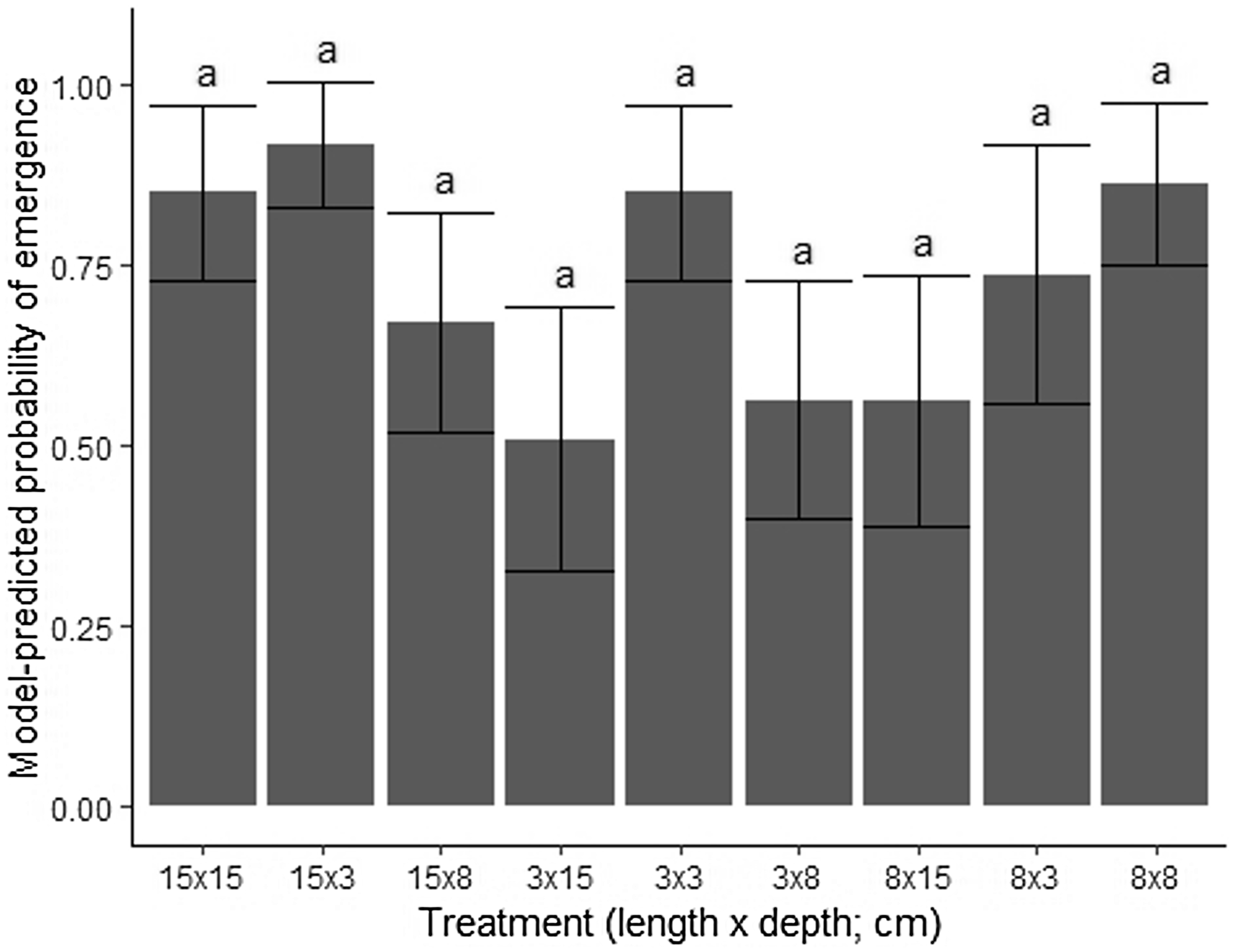
Figure 1. Effects of initial root segment length (cm) and planting depth (cm) on the model-predicted percent of Asclepias syriaca emergence having occurred at 10 wk postplanting. Error bars represent the standard errors. Treatments labeled with the same letter are not significantly different according to Tukey’s honest significant differences (HSD) test (α = 0.05).
In Run 1, aboveground biomass was affected by root segment length (P = 0.0005), but not the planting depth or the interaction between segment length and planting depth. Plants grown from a root segment that was 15-cm long had greater aboveground biomass, with a mean of 4.95 ± 0.60 (SE) g, compared with plants grown from 8- or 3-cm segments, which had average masses of 2.51 ± 0.40 (SE) and 1.69 ± 0.41 (SE) g, respectively (Figure 2). Similarly, belowground biomass (P < 0.0001) and height (P = 0.0004) were both only impacted by the length of the root segment that was planted. A root segment length of 15 cm was associated with greater biomass and height (Figures 3 and 4). Plants grown from 15-cm segments had an average belowground biomass of 5.97 ± 0.78 (SE) g and an average height of 30.60 ± 1.43 (SE) cm, plants grown from 8-cm segments had an average belowground biomass of 1.95 ± 0.29 (SE) g and an average height of 20.17 ± 1.87 (SE) cm, and plants grown from 3-cm segments had an average belowground biomass of 1.71 ± 0.50 (SE) g and an average height of 17.73 ± 3.05 (SE) cm. The root:shoot ratio was not impacted by either root segment length or planting depth in the first run of the experiment (Figure 5).
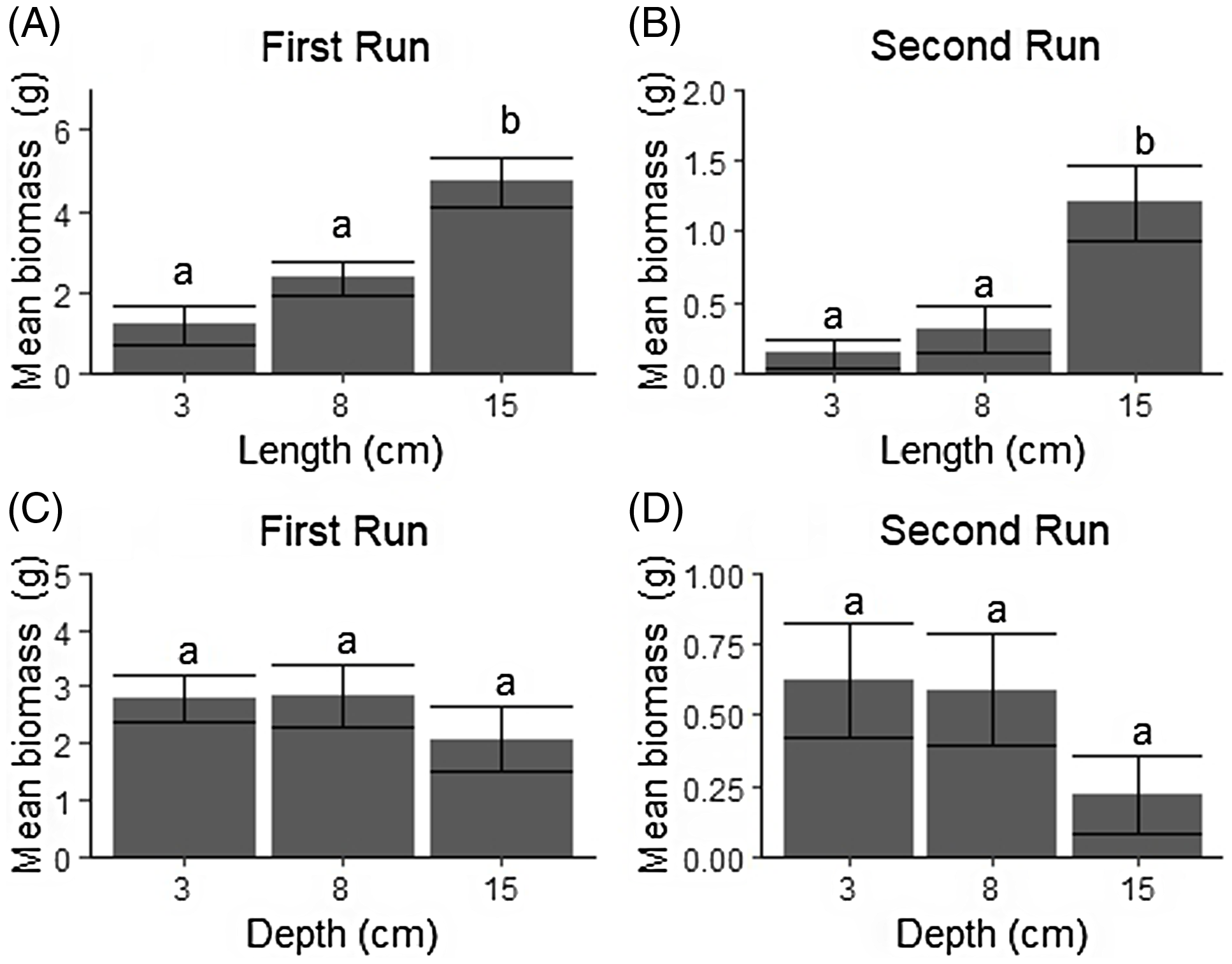
Figure 2. Effects of initial root segment length (cm) and planting depth (cm) on the model-predicted aboveground biomass (g) of Asclepias syriaca 10 wk postplanting. Data are presented for initial root segment length in the first (A) and second (B) runs, and planting depth in the first (C) and second (D) runs. Error bars represent the standard errors. Treatments labeled with the same letter are not significantly different according to Tukey’s honest significant differences (HSD) test (α = 0.05).
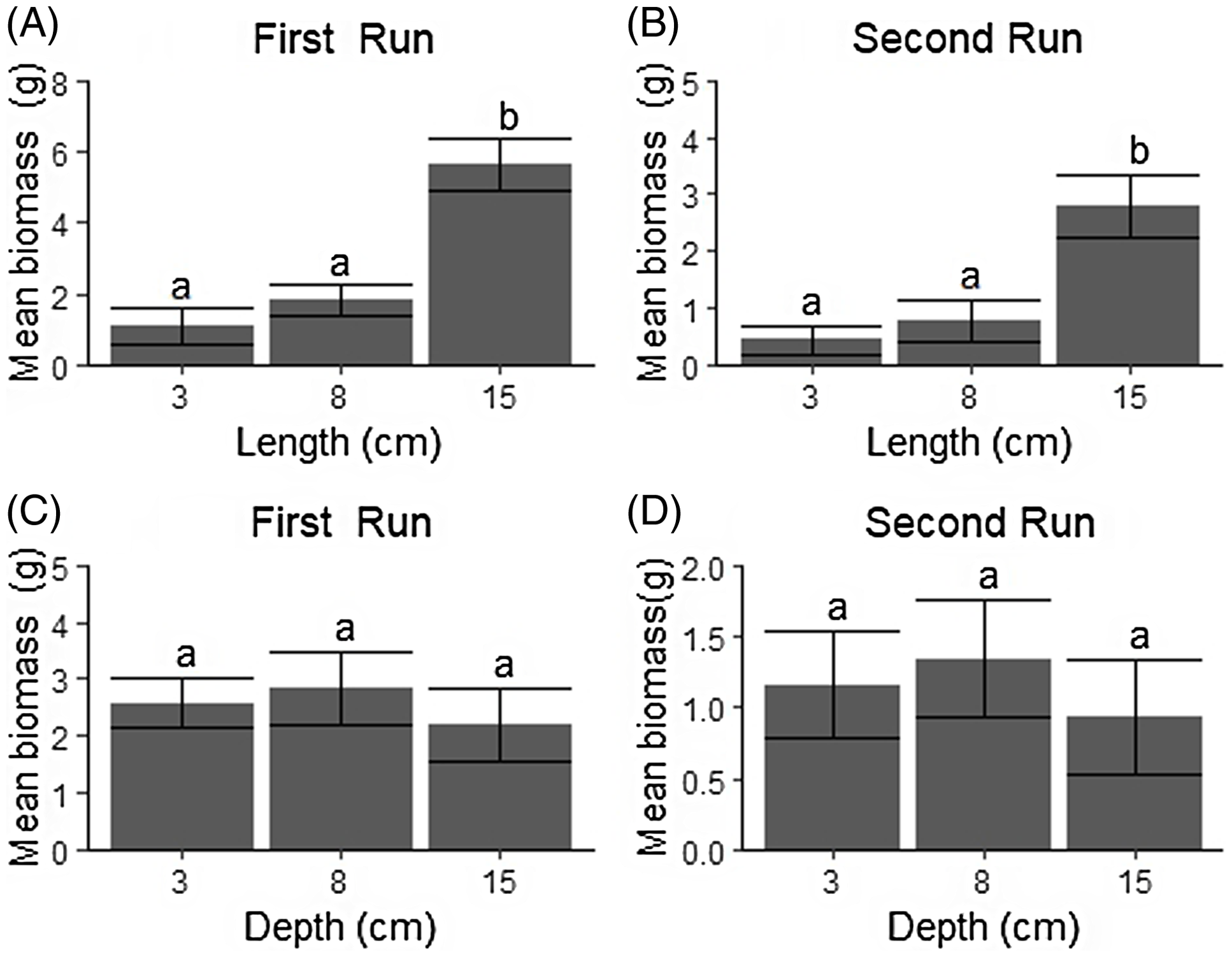
Figure 3. Effects of initial root segment length (cm) and planting depth (cm) on the model-predicted belowground biomass (g) of Asclepias syriaca 10 wk postplanting. Data are presented for initial root segment length in the first (A) and second (B) runs, and planting depth in the first (C) and second (D) runs. Error bars represent the standard errors. Treatments labeled with the same letter are not significantly different according to Tukey’s honest significant differences (HSD) test (α = 0.05).
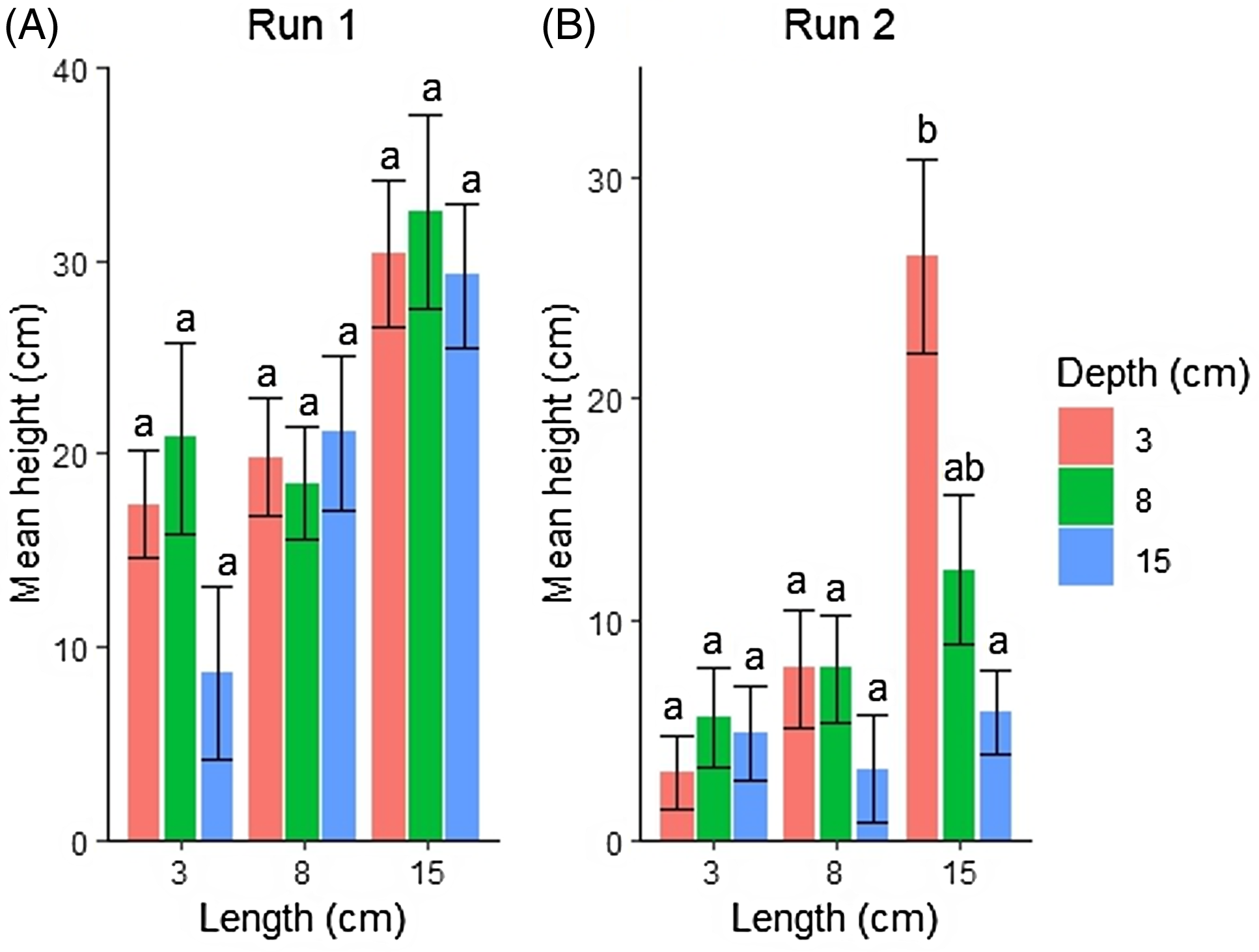
Figure 4. Effects of initial root segment length (cm) and planting depth (cm) on the model-predicted height (cm) of Asclepias syriaca 10 wk postplanting. Data are presented for the first (A) and second (B) runs. Error bars represent the standard errors. Treatments labeled with the same letter are not significantly different according to Tukey’s honest significant differences (HSD) test (α = 0.05).
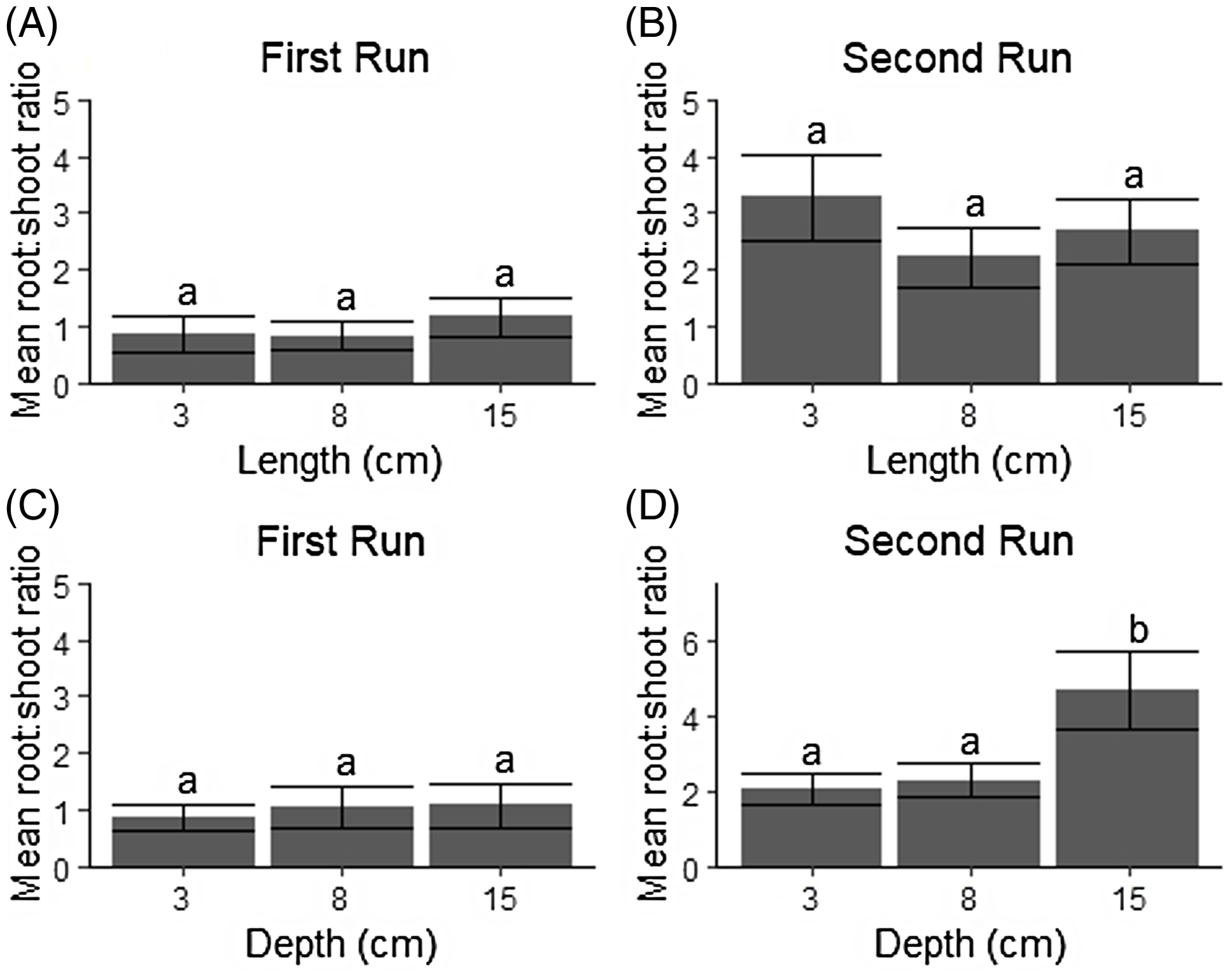
Figure 5. Effects of initial root segment length (cm) and planting depth (cm) on the model-predicted root:shoot ratio of Asclepias syriaca 10 wk postplanting. Data are presented for initial root segment length in the first (A) and second (B) runs, and planting depth in the first (C) and second (D) runs. Error bars represent the standard errors. Treatments labeled with the same letter are not significantly different according to Tukey’s honest significant differences (HSD) test (α = 0.05).
In Run 2, both aboveground (P = 0.0011) and belowground (P = 0.0009) biomass were affected by root segment length, but not by the planting depth or the interaction between the two. In both cases, a root segment length of 15 cm was associated with greater biomass than shorter root segment lengths (Figures 2 and 3). The aboveground and belowground biomass means were 1.56 ± 0.44 (SE) and 3.28 ± 0.79 (SE) g, respectively, for 15-cm segments; 0.54 ± 0.28 (SE) and 1.09 ± 0.42 (SE) g, respectively, for 8-cm segments; and 0.21 ± 0.09 (SE) and 0.57 ± 0.22 (SE) g, respectively, for the 3-cm segments. Plant height was most impacted by the initial length of the root segment (P = 0.0005), but planting depth (P = 0.03906) and the interaction between length and depth (P = 0.0200) were also significant. In this run, the shallowest planting depth (3 cm) was associated with a greater mean height (27.24 ± 4.53 [SE] cm) than the deepest planting depth of 15 cm when the root segment that was planted was 15 cm in length (6.17± 1.29 [SE] cm; Figure 4). The root:shoot ratio was impacted by planting depth (P = 0.0175) in the second run of the experiment. A deep planting depth of 15 cm in this run resulted in a greater mean root:shoot ratio (9.22) than either of the other planting depths (2.52 for the 8-cm segments and 2.38 for the 3-cm segments) (Figure 5).
Multiple factors might explain the discrepancy between runs regarding the effect of planting depth on A. syriaca height and biomass. The second run occurred during a time of year with a shorter photoperiod than the first run, which likely impacted overall plant growth. Another potential factor is the quality of the root segments used in each run. During the second run, fewer plants emerged when A. syriaca was grown from seed to produce roots, suggesting that a greater number of smaller segments from lower sections of the roots were collected out of necessity. These smaller segments likely had fewer resources available and were therefore more negatively impacted when planted at greater soil depths. This explanation aligns with previous research indicating that more successful vegetative propagation in Asclepias spp. is associated with the specific location from which root segments are collected. For example, in A. tuberosa, the likelihood of emergence is generally greater when segments are collected from the upper portion of the root (Ecker and Barzilay Reference Ecker and Barzilay1993).
There are also multiple factors that influence why certain parts of the roots are more suitable for vegetative propagation. In A. syriaca, root buds begin developing earlier and in greater numbers on parts of the root that are close to the soil surface (Polowick and Raju Reference Polowick and Raju1982). Additionally, the greatest proportion of carbohydrates by weight in A. syriaca roots occurs at soil depths of 15 to 30 cm, with the second-greatest amount occurring at depths of 0 to 15 cm (Evetts and Burnside Reference Evetts and Burnside1974). It is possible that the impact of root length on total biomass in this study was partially due to the 15-cm root segments benefiting from both greater reproductive capacity of the upper portion of roots and increased carbohydrate content of the lower portion of the roots.
Plants grown from 15-cm root segments were generally larger than plants grown from shorter root segments at 10 wk after planting. For those interested in planting A. syriaca for conservation purposes, these results indicate that propagating from root segments of 15 cm or longer may lead to greater growth than using shorter segments. However, if this is not possible, shorter segments may be used, as total emergence rates did not differ by root segment length. Any planting depth within the top 15 cm of soil is likely to produce similar results, and the planting depth is not as important a consideration as the initial root segment length.
Future studies could investigate how initial root segment length impacts propagation under field conditions and over the course of multiple growing seasons. A longer root segment appears to be beneficial for plants during early growth, but it may not be as important after the first growing season. Additionally, plants grown under field conditions face various pressures that do not occur in a greenhouse study. For example, herbivory decreases clonal reproduction and slows population growth in A. syriaca (Dalgleish et al. Reference Dalgleish, Kula, Yair, Munkres, Mutterperl, Struckman and LaMar2024). It is possible that an increased root segment length might contribute to increased tolerance of such biotic or abiotic stressors during initial establishment under field conditions.
Overall, our study suggests that land managers who plan to vegetatively propagate A. syriaca should consider starting with root segments cut to 15 cm or longer. This approach could be beneficial even if plants are being propagated as a seed source, because plants grown from large rhizomes have been known to flower in the first year (Landis Reference Landis2014). Vegetative propagation also allows the large-scale production of unique genotypes with desirable traits (Luna Reference Luna, Dumroese, Luna and Landis2009). At the same time, it is important to note that vegetative propagation is not the best approach in all situations. For example, vegetative propagation is quicker than planting from seed but results in populations with less genetic diversity (Luna Reference Luna, Dumroese, Luna and Landis2009). Further research on all methods of establishing A. syriaca will support restoration of this beneficial native species.
Acknowledgments
The authors gratefully acknowledge the assistance of Scott Anthony, Ryleigh Coffey, and Rosa Xia.
Funding statement
This work was supported by a grant awarded to RSS by the Weed Science Society of America’s John Jachetta Undergraduate Research Program (2023).
Competing interests
The authors declare no competing interests.