Methionine (Met) is considered to be the highest priority amino acid in poultry diets as it is the first limiting amino acid and has multiple biological functions in avian species( Reference Bunchasak 1 ). Dietary Met levels affect the secretion of hormones in animals, such as triiodothyronine, insulin growth factor-II, estradiol, follicle-stimulating hormone and luteinising hormone( Reference Carew, McMurtry and Alster 2 – Reference Meng, Song and Li 4 ). In avian species, Met deficiency impairs the laying performance, egg mass, feed conversion ratio (FCR)( Reference Xiao, Wang and Liu 5 – Reference Fouad, Ruan and Lin 7 ), antibody production( Reference Hosseini, Zaghari and Lotfollahian 8 ) and retards female reproductive organ development( Reference Barnes, Calvert and Klasing 9 – Reference Del Vesco, Gasparino and Grieser 11 ), including relative weight of the ovary, oviduct length, total number of follicles, large and small, and the growth rate of secondary follicles( Reference Meng, Song and Li 4 ). Met deficiency can lead to imbalanced lipid and protein metabolism, which reduces egg quality and increases the incidence of reproductive problems associated with fatty liver and obesity( Reference Corzo, Kidd and Dozier 10 – Reference Kikusato, Sudo and Toyomizu 13 ). The Met is involved in the biosynthesis of GSH, cysteine, taurine and carnitine( Reference Yin, Ren and Yang 14 ), which play vital roles as antioxidants( Reference Lee, Han and Nakamura 15 – Reference Jia, Bao and Zhang 17 ). Several studies have reported that dietary Met supplementation increased the content of cysteine, taurine and the activities of antioxidant enzymes, and reduced the lipid peroxidation in Chinese broiler breeders and commercial broiler chickens( Reference Xiao, Wang and Liu 5 , Reference Vesco, Gasparino and Grieser 18 ). However, it was reported that dietary Met levels ranging from 2·5 to 5·0 g/kg did not affect the plasma glutathione redox system in laying ducks, which was possibly due to not low enough Met level in the basal diet( Reference Fouad, Ruan and Lin 7 ). In this regard, it was hypothesised that a control diet containing less Met (2·0 g v. 2·5 Met/kg) might affect the activities of antioxidant enzymes and their mRNA expression levels in duck breeders and their offspring by modulating the expression of nuclear factor erythroid 2 like 2 (Nrf2), but this has not been investigated previously. Moreover, previous reports have shown that dietary Met levels increases the egg weight and albumen secretion in different strains of laying ducks and laying hens, which was reflected in egg weight( Reference Bunchasak, Ratchadapornvanitch and Thiengtham 3 , Reference He, Li and Gao 6 , Reference Fouad, Ruan and Lin 7 ), but the relationship between them was little referred. Thus, it might be possible that dietary Met can induce the same effect in duck breeders as laying ducks, which may lead to an increase in the weight of hatchings, by altering gene expression of ovalbumin (OVAL, the dominant protein in albumen) in duck breeders.
In poultry, Met improves the productivity, reproductive performance, egg quality, antioxidant defence system and humoral and cellular immune responses, and attenuates the adverse impacts of non-optimal temperature (cold or heat stress)( Reference Xiao, Wang and Liu 5 , Reference Fouad, Ruan and Lin 7 , Reference Vesco, Gasparino and Grieser 18 , Reference Yang, Zhang and Ding 19 ). Thus, the effects of Met on commercial and local strains of broiler breeders, laying hens, quails, turkeys, meat-type and egg-type ducks can be positive, but variable( Reference Bunchasak, Ratchadapornvanitch and Thiengtham 3 , Reference He, Li and Gao 6 , Reference Fouad, Ruan and Lin 7 , Reference Vesco, Gasparino and Grieser 18 , Reference Liu, Lin and Zhou 20 , Reference Zeng, Zhang and Chen 21 ). Further, few studies have reported the effect of dietary Met levels in laying duck breeders. The Longyan duck breeders are the most popular laying ducks for duckling production in South China, mainly because of their high productivity, adaption to high ambient temperature and resistance to diseases. Therefore, the current study sought to determine the effect of dietary Met levels on productivity, reproductive performance, antioxidant capacity, OVAL and antioxidant-related gene expression in laying duck breeders; it also examined whether dietary Met increases the secretion of albumen by upregulating expression of the OVAL gene in duck breeders and improving the activities of antioxidant enzymes and their gene expression levels in duck breeders and their offspring by modulating the expression of Nrf2.
Methods
Birds, diets and experimental design
All experimental procedures were approved by the Animal Care and Use Committee of Guangdong Academy of Agriculture Sciences, China. A total of 540 female Longyan laying duck breeders with the same genetic background in terms of the parental generation and with comparable body weight (BW) at 19 weeks of age were randomly assigned to six dietary treatments, each comprising six replicates of fifteen birds. The ducks were individually housed in galvanised steel battery cages (length 42 cm×width 30 cm×height 50 cm; Guangzhou Huanan Poultry Equipment Co., Ltd) with a nipple drinker and feeder; the ambient temperature varied from 15 to 32°C during the 24-week experimental period. Fresh drinking water was available ad libitum, and 80 g of feed per duck in pellet form was introduced twice daily at 07.00 and 15.00 hours. A 16 h light–8 h dark photoperiod was applied throughout the study.
The basal diet (Table 1) was formulated to satisfy the nutritional requirements for Longyan laying duck breeders with the exception of Met. The six groups were fed the basal diet supplemented with 0 (control), 0·75, 1·50, 2·25, 3·00 or 3·75 g Met/kg in the form of DL-Met (MetAMINO®; Evonik Industries AG), respectively, which resulted in total contents of 2·00, 2·75, 3·50, 4·25, 5·00 and 5·75 g/kg. Male Longyan duck breeders were fed a standard commercial diet containing 10·87 MJ/kg metabolisable energy/kg, 170 g/kg crude protein, 8·00 g/kg Ca, 3·80 g/kg available phosphorus and 4·50 g/kg Met. At 38 weeks of age, each breeder was artificially inseminated twice weekly with 100 µl of pooled semen( Reference Gvaryahu, Robinzon and Meltzer 22 ) to evaluate reproductive performance (fertility, hatchability and proportion of healthy ducklings). In total, 1800 eggs (fifty eggs from each replicate) were collected over 5 sequential days between 38 and 39 weeks from the 2nd day after the first artificial insemination, the eggs were weighed, labelled and stored in a dark controlled-temperature room (18°C and 75 to 80 % relative humidity), and then incubated in the incubator (JXB2000; Dezhou Jingxiang Technology Co., Ltd) for 28 d. On the first 5 d, the incubator temperature was 38·4°C with 45 % humidity; from 6 to 10 d, the temperature was 38·0°C with 50 % humidity; from 11 to 15 d, the temperature was 37·5°C with 50 % humidity; from 16 to 20 d, the temperature was 37·1°C with 55 % humidity; from 21 to 25 d, the temperature was 36·8°C with 60 % humidity; and from 26 to 28 d, the temperature was 36·5°C with 65 % humidity. The eggs were candled on days 6 and 18 to eliminate infertile eggs and dead embryos. The fertility and hatchability were determined along with hatchling weights of ducklings being recorded on the hatching day.
Table 1 Ingredients and nutrient composition of the basal diet for laying duck breeders, as-fed basis
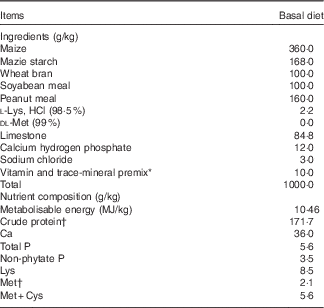
* The premix provided per kilogram diet: vitamin A, 3·96 mg; vitamin D3, 0·066 mg; vitamin E, 20 mg; vitamin K3, 2·75 mg; vitamin B1, 4·4 mg; vitamin B2, 9·0 mg; vitamin B6, 4·4 mg; vitamin B12, 0·02 mg; choline, 500 mg; nicotinic acid, 30 mg; pantothenic acid, 22 mg; folic acid, 1·1 mg; biotin, 0·22 mg; Fe from ferrous sulphate, 60 mg; Cu from copper sulphate, 8·0 mg; Mn from manganese oxide, 100 mg; Zn from zinc oxide, 90 mg; iodine from ethylenediamine dihydroidide, 0·5 mg; Se from sodium selenite, 0·2 mg; Co from cobaltous sulphate, 0·26 mg.
† Measured values of crude protein and methionine content. Each value is based on triplicate determinations. Other nutrient compositions are calculated values.
Sample collection
At the end of the experiment (after feeding for 24 weeks), twelve breeders per treatment were selected at random (excluding obvious outliers in terms of BW, two breeders in each replicate) for sampling. Heparinised blood was collected from the wing vein, centrifuged at 1000 g for 20 min at 4°C and the plasma was then collected and stored at −20°C until further analysis. The breeders were then stunned, exsanguinated and the ovaries were collected and weighed. Samples were collected from the liver, oviduct magnum and ovaries( Reference Zhao, Zhang and Jiao 23 , Reference Xia, Fouad and Chen 24 ), which were rinsed rapidly with PBS, snap-frozen in liquid N2 and stored at −80°C. After hatching, twelve ducklings from each treatment group (two ducklings from each replicate) were stunned, exsanguinated and samples of the liver and brain( Reference Tsunekage and Ricklefs 25 ) were harvested, rinsed quickly with PBS, snap-frozen in liquid N2 and stored at −80°C.
Determination of productive and reproductive performance
The feed intake was recorded daily on a per replicate basis. The numbers of total, broken and shell-less eggs were recorded daily by replicate. The eggs produced were individually weighed and graded daily. Egg production (%) (100×number of eggs/number of breeders), egg weight (g), egg mass (g/d), average daily feed intake and FCR (g feed/g egg) were calculated daily, on a per replicate basis, and the data were then pooled before analysis. The fertility and hatchability were also calculated, and the proportion of healthy ducklings was calculated as the number that were clean, dry, free of deformities and with bright eyes, as described by Tona et al. ( Reference Tona, Onagbesan and De Ketelaere 26 ), expressed as a percentage relative to the total number of hatchlings.
Egg quality
At the end of the experiment, four eggs were collected at random (excluding obvious outliers such as excessively large or small, rough, misshapen, cracked) from each replicate, and the average of these twenty-four eggs was calculated per treatment to determine the quality traits. Yolk colour score, albumen height and Haugh unit (albumen quality) were measured on the day of collection using an Egg Analyzer (model EA-01; ORKA Food Technology). The strength of the shell was determined on the vertical axis using an Egg Force Reader (model EFR-01; ORKA Food Technology). Eggshell thickness was measured using a digital micrometre, and egg shape index (SI) was determined with a digital caliper and calculated as SI=width×100/length, where the length was the distance between the blunt and pointed ends and the width was the diameter at the mid-length. The eggs were weighed, and then the yolks were separated, weighed and expressed as percentages of egg weight. The shells with membranes were weighed after drying at 105°C and expressed as percentages of egg weight. Finally, the albumen weight was calculated as egg weight minus the weight of yolk and eggshell, and expressed as percentages of egg weight.
Ovary and oviduct-related indices
The collected ovaries, small yellow follicles (SYF; 3 mm<diameter<8 mm), large yellow follicles (LYF; diameter≥8 mm) and oviduct were weighed using an electronic balance (AB-S/PH; Mettler Toledo). The numbers of total SYF and LYF were recorded. The weights of total SYF and LYF were calculated relative to the ovarian weight and expressed as ratios.
Biochemical assay of plasma and tissue samples
The plasma contents of GSH and malondialdehyde (MDA), activities of total superoxide dismutase (T-SOD) and glutathione peroxidase (GPX), and the total antioxidant capacity (T-AOC) were determined in duplicate colourimetrically using commercial kits according to the manufacturer’s protocols (Jiancheng Bioengineering Institute), similar to that described by Huang et al. ( Reference Huang, Jiao and Song 27 ).
A quantity of 40 mg of frozen liver or brain were homogenised on ice in 4 ml of homogenisation buffer (0·05 m TRIS-HCl, pH 7·4, 1 mm EDTA, 0·25 m sucrose) with an Ultra-Turrax (T8; IKA) for 5 s at 3000 g . The homogenate was centrifuged at 12 000 g for 10 min at 4°C, and the supernatant was stored at −80°C. The concentrations of GSH, MDA and activities of T-SOD, GPX and T-AOC in the livers of breeders, as well as in the livers and brains of ducklings, were measured with kits (Jiancheng Bioengineering Institute). The concentrations of protein carbonyl (PC) in the brains of ducklings were measured with kits (Jiancheng Bioengineering Institute), similar to that described by Huang et al. ( Reference Huang, Jiao and Song 27 ). All samples were measured in duplicate at appropriate dilutions in order to determine the concentrations of GSH, MDA, PC and activities of the enzymes in the linear range for standard curves constructed with pure enzymes. The protein contents of supernatants were determined using Coomassie Brilliant Blue G250 (Sigma Chemical) with bovine serum albumin as the standard.
Total RNA extraction and reverse transcription
Total RNA was extracted from the frozen tissues using TRIzol® (Invitrogen) and treated with DNase (Takara) according to the manufacturers’ protocols. RNA concentrations were determined by NanoDrop 1000 (ThermoFisher Scientific) and RNA integrity was verified by electrophoresis on 1 % agarose gels.
Total RNA (2·5 µg) from each sample was used to generate complementary DNA (cDNA) in a final volume of 20 µL according to the manufacturer’s instructions (Promega). The cDNA was then diluted 1:10 with nuclease-free water (Ambion) and stored at −20°C until use. Primers for the genes of interest were designed with Primer Premier 5.0 based on duck sequences in GenBank, as shown in Table 2; they were obtained from Shanghai ShengGong Biological Company. The cDNA was amplified by PCR under the optimal conditions, which consisted of an initial 5-min denaturation at 95°C; thirty-five cycles of 30 s at 95°C, 30 s at the annealing temperature (Ta) and extension for 30 s at 72°C; and a final extension at 72°C for 10 min. Aliquots of PCR products were evaluated by electrophoresis in 1·5 % agarose gels and excised products from the gels were sequenced to verify authenticity.
Table 2 Primer sequences used for quantitative real-time PCR

Ta, annealing temperature; SOD1, superoxide dismutase 1; GPX1, glutathione peroxidase 1; CAT, catalase; HO-1, haem oxygenase 1; Nrf2, nuclear factor erythroid 2 like 2; OVAL, ovalbumin.
Quantitative real-time PCR analysis
Quantitative real-time PCR was performed using the same primers to quantify mRNA contents. Each 20 µl PCR mixture contained 10 µl of 2X iQTM SYBR Green Supermix, 0·8 µl (10 mm) of each primer and 2 µl of cDNA. The mixtures were incubated using an iCycler iQ Real-time Detection system (Bio-Rad) for thirty-nine cycles (95°C for 15 s and at the Ta for 30 s). Quantification of the transcripts was performed using a standard curve with 10-fold serial dilutions of cDNA. A melting curve was constructed to verify that only a single PCR product was amplified. Samples were assayed in triplicate with standard deviations of threshold cycle (C
t
) values not exceeding 0·5. The
$$2^{{{\minus}\Delta \Delta C_{t} }} $$
method was used with β-actin as the reference transcript for relative gene expression and then expressed relative to the transcript abundance in birds fed the control diet(
Reference Livak and Schmittgen
28
).
Statistical analysis
Replicate (n 6) served as the experimental unit, where two breeders and ducklings were sampled per replicate unless stated otherwise. The experimental data were analysed by one-way ANOVA using the general linear model procedure in SAS (version 9.2; SAS Institute Inc.). Differences between means were assessed using Duncan’s multiple range tests at P<0·05 probability level. Results are expressed as means with their standard errors, derived from the ANOVA error mean square. Only when the main effect was shown to be significant (P<0·05), orthogonal comparisons were used to estimate the linear and quadratic effects of Met supplementation. Quadratic regressions (Y=c+bX+aX 2) were fitted to the responses of the dependent variables to Met supplementation. The dietary concentration of Met at which the response first reached 95 % of the maximum was used to estimate the requirement( Reference Dozier, Corzo and Kidd 29 ).
The sample size (n) was estimated using the following equation:
$n\,{\equals}\,\psi ^{2} \left( {\mathop{\sum}{\left( {S_{i}^{2} } \right)} \,/\,K} \right)/\left[ {\mathop{\sum}{\left( {X_{{i\,{\rm average}}} {\minus}X_{{{\rm average}}} } \right)} ^{2} \!/\left( {K{\minus}1} \right)} \right]$
(
Reference Desu and Raghavarao
30
), where n is the sample size, Ψ is ψ value, K is the group count, α is the probability of a type I error occurring at 5 %, β is the probability of a type II error occurring at 10 %, S
i
and X
i average are the standard deviation and the average value of corresponding ith group, respectively. For example, for the most variable index, Ψ=3·08, α=0·05, β=0·10, X
average=2·00 and the sample size needed to detect a significant main effect between groups was at least n 6 ducks per group.
Results
Productive and reproductive performance
Dietary Met significantly affected (P<0·05) the egg weight, egg mass, FCR, hatchability, healthy ducklings and weight of hatchlings (Table 3). The egg weight and 1 d duckling weight increased in a linear (P<0·001) manner, with a lesser quadratic (P<0·05) component as Met concentration increased. The egg mass increased, and then decreased quadratically (P<0·05) as dietary Met concentration increased. The hatchability and proportion of healthy ducklings increased in a linear (P<0·05) and quadratic (P<0·05) manner as Met concentration increased. There were no significant effects (P>0·05) of dietary Met levels on daily egg production and fertility.
Table 3 Effects of the dietary methionine (Met) level on the productivity (19–43 weeks) and reproductive performance (38–39 weeks) of duck breeders in the peak laying period (Mean values with their standard errors, n 6)
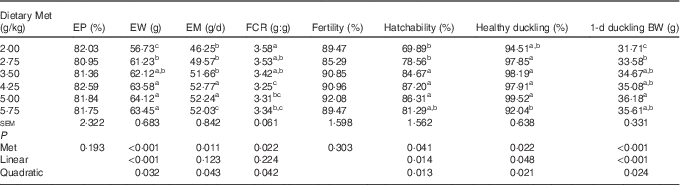
EP, egg production; EW, egg weight; EM, egg mass; FCR, feed conversion ratio; BW, body weight.
a,b,c Mean values within a column with unlike superscript letters were significantly different (P<0·05).
Egg quality
Among the egg quality indices (Table 4), the albumen weight, albumen proportion and Haugh unit increased in a linear (P<0·05) and quadratic (P<0·05) manner with dietary Met concentration, whereas the yolk proportion decreased in a linear (P<0·001) and quadratic (P<0·05) manner. There were no significant effects (P>0·05) of increasing Met on the eggshell thickness, egg SI, eggshell strength, yolk colour and eggshell weight and proportion.
Table 4 Effects of the dietary methionine (Met) level on the egg composition and quality of duck breeders during the laying period from 19 to 43 weeks of age (Mean values with their standard errors, n 6)
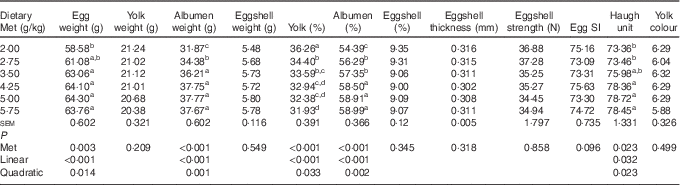
SI, shape index.
a,b,c,d Mean values within a column with unlike superscript letters were significantly different (P<0·05).
Ovary and oviduct-related indices
As shown in Table 5, the weight of total LYF increased linearly (P<0·001) with a minor quadratic effect (P<0·05), and the ratio of total LYF weight/ovarian weight was also affected in a linear (P=0·002) manner by dietary Met concentration. The ovarian weight (g/kg BW) increased in a linear (P<0·05) and quadratic (P<0·05) manner with dietary Met concentration. There were no significant effects (P>0·05) of increasing Met on the number of total SYF and LYF, nor the weight of total SYF and oviduct.
Table 5 Effects of the dietary methionine (Met) level on ovary and oviduct-related indices for duck breeders at 43 weeks of age (Mean values with their standard errors mean, n 6)

LYF, large yellow follicles, follicles with mean diameter>8 mm; SYF, small yellow follicles, follicles with mean diameter of 3 to 8 mm; BW, body weight.
a,b,c Mean values within a column with unlike superscript letters were significantly different (P<0·05).
Antioxidative indices and related gene expression in the liver of laying duck breeders
Table 6 shows that the hepatic content of GSH, and the hepatic activities of GPX and T-AOC increased quadratically (P<0·01) as dietary Met concentration increased; hepatic content of MDA showed reciprocal changes, with only a trend towards a quadratic (P=0·062) effect. Dietary Met levels increased the hepatic expression of GPX1 in a linear (P<0·05) and quadratic (P<0·001) manner in laying duck breeders, and the expression of catalase (CAT) changed linearly (P<0·001) with the Met levels in diet. There was a linear (P<0·01) and quadratic (P<0·01) effect of dietary Met levels on the hepatic expression of haem oxygenase 1 (HO-1), whereas Nrf2 changed quadratically (P<0·001) with dietary Met levels (Table 7). There were no significant effects (P>0·05) on the plasma contents of GSH or MDA, nor on the activities of T-SOD, GPX or T-AOC (data not shown).
Table 6 Effects of the dietary methionine (Met) level on antioxidative indices in livers of duck breeders at 43 weeks of age (Mean values with their standard errors, n 6)

prot, Protein; T-SOD, total superoxide dismutase; GPX, glutathione peroxidase; T-AOC, total antioxidant capacity; MDA, malondialdehyde.
a,b Mean values within a column with unlike superscript letters were significantly different (P<0·05).
Table 7 Effects of the dietary methionine (Met) level on the relative hepatic expression of genes related to antioxidation and ovalbumin gene expression in oviduct magnum in duck breeders at 43 weeks of age (Mean values with their standard errors, n 6)

SOD1, superoxide dismutase 1; GPX1, glutathione peroxidase 1; CAT, catalase; HO-1, haem oxygenase 1; Nrf2, nuclear factor erythroid 2 like 2; OVAL, ovalbumin; a.u., arbitrary units.
a,b,c Mean values within a column with unlike superscript letters were significantly different (P<0·05).
Ovalbumin gene expression in the oviduct magnum of duck breeders
There was an obvious linear (P<0·001) and quadratic (P<0·001) effect of dietary Met on the relative abundance of OVAL transcripts in the oviduct magnum (Table 7).
Antioxidative indices and related genes expression in the liver and brain of hatchlings
As shown in Table 8, the cerebral but not hepatic activities of T-SOD, GPX and T-AOC in newly hatched ducklings increased quadratically (P<0·01) as Met content of the breeders’ diet increased; reciprocal quadratic (P<0·05) changes in PC were evident. There were no effects on GSH or MDA contents of either tissue. Dietary Met for the breeders had quadratic (P<0·05) effects on the cerebral expression of GPX1, HO-1 and Nrf2 and hepatic expression of HO-1 in the hatchlings (Table 9).
Table 8 Effects of the maternal dietary methionine (Met) level of duck breeders at 38 weeks of age on antioxidant indices in the liver and brain of hatchlings (Mean values with their pooled standard errors, n 6)

prot, Protein; T-SOD, total superoxide dismutase; GPX, glutathione peroxidase; T-AOC, total antioxidant capacity; MDA, malondialdehyde; PC, protein carbonyl.
a,b Mean values within a column with unlike superscript letters were significantly different (P<0·05).
Table 9 Effects of the maternal dietary methionine (Met) level of duck breeders at 38 weeks of age on the relative hepatic and cerebral expression levels of genes related to antioxidation in hatchlings (Mean values with their pooled standard errors, n 6)
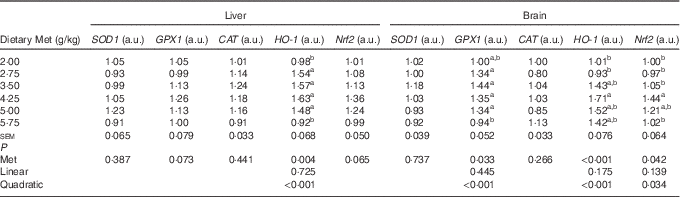
SOD1, superoxide dismutase 1; GPX1, glutathione peroxidase 1; CAT, catalase; HO-1, haem oxygenase 1; Nrf2, nuclear factor erythroid 2 like 2; a.u., arbitrary units.
a,b Mean values within a column with unlike superscript letters were significantly different (P<0·05).
Estimations of the dietary methionine requirements of laying duck breeders
Results of dietary Met requirements of laying duck breeders as estimated by the quadratic regression analysis are shown in Table 10. The dietary Met requirements for Longyan duck breeders from 19 to 43 weeks of age for optimising egg weight, albumen weight, egg mass, FCR, hatchability, maximal expression of hepatic GPX1 and Nrf2 and its activities were 4·6, 4·9, 4·4, 4·8, 4·0, 4·0, 4·0 and 4·0 g/kg, respectively.
Table 10 Estimations of the dietary methionine (Met) requirements based on quadratic regressions of egg weight, albumen weight, egg mass and hatchability, glutathione peroxidase 1 (GPX1), nuclear factor erythroid 2 like 2 (Nrf2) mRNA and GPX activity on dietary Met concentrations
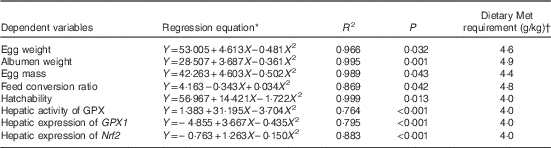
* Y is the dependent variable and X is the dietary Met concentration (g/kg).
† Dietary Met requirement=the optimal dietary Met concentration (g/kg).
Discussion
In this study, dietary Met levels affected the egg weight, egg mass, FCR, hatchability, proportion of healthy ducklings and their weight, but did not affect egg production and fertility. Previously, Xiao et al. ( Reference Xiao, Wang and Liu 5 ) found improvements in egg production, FCR, fertility and hatchability after increasing the Met level from 0·25 to 0·35 % in Chinese broiler breeders, whereas Hosseini et al. ( Reference Hosseini, Zaghari and Lotfollahian 8 ) showed that increasing the Met concentrations from 0·20 to 0·45 % for 8 weeks during the peak laying period in Iranian broiler breeders impaired egg production, egg mass and FCR, but did not affect hatchability, quality of chicks or their weight. In the previous study of Longyan laying ducks from 19 to 47 weeks of age, improvements in egg weight were found after increasing Met level from 0·25 to 0·50 %, but egg production, egg mass and FCR were not affected( Reference Fouad, Ruan and Lin 7 ). In contrast, in Shaoxing laying ducks, He et al. ( Reference He, Li and Gao 6 ) recorded improved egg production, egg weight, egg mass and FCR from increasing the Met concentration from 0·26 to 0·54 % for 6 weeks during the late laying period. A similar improvement in egg production was obtained after doubling the amount of Met in the basal diets (0·26 %) of Tsaiya laying ducks for 4 weeks( Reference Lien and Jan 31 ). Feeding laying hens diets containing 0·26 % Met for 12 weeks starting from 34 weeks of age impaired egg production, egg weight, egg mass and FCR, but adding 0·10 % Met normalised these effects( Reference Bunchasak, Ratchadapornvanitch and Thiengtham 3 ). In White Leghorn laying hens, Panda et al. ( Reference Panda, Rao, Raju and Bhanja 32 ) showed that egg production, egg weight, egg mass and FCR were not affected by increasing the Met level from 0·30 to 0·42 % in different feed sources for 16 weeks during the peak laying period. Thus, previous studies using different bird ages, strains and/or Met concentrations in the basal diet varied in the responses to increasing dietary levels of Met. The Longyan breed used here is the prevalent duck laying breed in southern China and is of great consequence as more than 80 billion eggs are produced annually. Optimising nutrient provision for the layers and breeders is of economic importance, so the present findings are commercially relevant.
Albumen weight, albumen proportion and Haugh unit increased significantly, and the yolk proportion decreased with increasing dietary Met in the present study; other indices of egg quality were unchanged. In Longyan laying ducks, egg SI, Haugh unit, yolk colour, yolk weight and eggshell weight did not change but the albumen weight increased, and thickness, relative weight and strength of eggshell decreased by increasing dietary Met from 0·25 to 0·50 %( Reference Fouad, Ruan and Lin 7 ). In Shaoxing laying ducks, the Haugh unit and yolk colour were not affected, but the albumen height and eggshell thickness increased after the Met levels changed from 0·26 to 0·54 %( Reference He, Li and Gao 6 ). Supplementing the diets of laying hens with various formulations of Met had inconsistent effects on the egg quality traits that were affected in the present study with Longyan breeders( Reference Bunchasak, Ratchadapornvanitch and Thiengtham 3 , Reference Liu, Lin and Zhou 20 , Reference Panda, Rao, Raju and Bhanja 32 ). In the current study, increasing dietary Met led to linear increases in the albumen weight, together explaining the increase in egg weight and likely accounting for the weight of hatchlings( Reference Williams 33 ).
Albumen (egg white) consists mainly (54 %) of the major protein OVAL( Reference Williams 33 ), lysozyme and ovotransferrin proteins. The expression of OVAL mRNA in the oviduct magnum (the main location for albumen secretion) increased linearly and quadratically as the Met concentration increased. To the best of our knowledge, this is the first report of enhanced expression of OVAL mRNA owing to increasing dietary Met in any avian species. In laying ducks, dietary Met supplementation increased the plasma levels of Met, lysine, arginine, isoleucine and histidine( Reference He, Li and Gao 6 ), all of which are essential components of OVAL( Reference Smith and Back 34 ), which may explain why dietary Met enhanced the mRNA expression level of OVAL here. This finding may explain why increasing the Met level increased the albumen weight and hatchling weight because albumen has multiple physiological functions during different phases of embryo development( Reference Moran 35 ). Exactly the same reasoning could explain the lowered hatchability of eggs from duck breeders fed low dietary Met (2·00 or 2·75 g/kg).
Ovarian-related indices( Reference Fouad, Chen and Ruan 36 ) were measured to evaluate the effects of Met on development of the reproductive system. Dietary Met influences secretion of follicle-stimulating hormone and luteinising hormone, which stimulate the growth and development of follicles( Reference Meng, Song and Li 4 ). The weight of total LYF and the fraction of ovarian weight they accounted for were the only variables affected by dietary Met fed to the breeder ducks. The present results were consistent with the basal diet, with 2·00 g/kg, being deficient in Met for breeder ducks. Met-deficient diets in laying hens depressed protein synthesis in the liver (one function being to produce and export egg yolk proteins), magnum and other segments of the oviduct( Reference Hiramoto, Muramatsu and Okumura 37 ). Other effects of Met deficiency include a decrease in breast meat yield in broilers( Reference Barnes, Calvert and Klasing 9 ) and influences on expression of genes related to protein turnover( Reference Del Vesco, Gasparino and Grieser 11 ).
In the present study, dietary Met supplementation enhanced the hepatic levels of GSH, and activities of GPX and T-AOC, as well as the expression of HO-1and Nrf2 genes in laying duck breeders. Supplemental Met reduced levels of MDA with a trend towards a quadratic effect, while not changing T-SOD activity or SOD1 gene expression. The transcription factor Nrf2 plays a crucial role in maintaining stable liver functioning by regulating the expression of antioxidants (GPX, HO-1, CAT and SOD)( Reference Li and Kong 38 , Reference Lee, Lin and Yu 39 ). The HO-1 has a crucial role in generating biliverdin and bilirubin, which are involved in the antioxidant defence system, and nutritional factors modulate Nrf2 in order to increase antioxidant defences( Reference Ryter, Alam and Choi 40 , Reference Alam and Cook 41 ). One previous study in young grass carp fish( Reference Pan, Wu and Feng 42 ) investigated whether an adequate level of Met improved the antioxidant status by upregulating the expression of Nrf2 mRNA. Our previous study in Longyan laying ducks showed that increasing dietary Met from the 2·5 to 5·5 g/kg did not affect GSH, GPX or MDA in plasma( Reference Fouad, Ruan and Lin 7 ). In 16-week-old turkey hens, increasing Met from the levels recommended( 43 ) had no effect on MDA, GPX and SOD activity in plasma or breast muscles, but hepatic levels of SOD and GSH declined and MDA increased significantly( Reference Jankowski, Kubińska and Juśkiewicz 44 , Reference Jankowski, Kubińska and Juśkiewicz 45 ). There are numerous studies in various avian species and types( Reference Gvaryahu, Robinzon and Meltzer 22 , Reference Park, Pasquetti and Malheiros 46 – Reference Chen, Chen and Zhang 50 ) on effects of dietary Met at below and above recommended levels on antioxidant indices in laying duck breeders. As noted earlier, inconsistencies in results and differences to what has been observed here mainly reflect the differences in the animal models, Met deficiency level and tissue selected for evaluating the antioxidant status. In addition to the effects of graded dietary Met on the antioxidant status of breeders, and the effects measured on their eggs, some ‘carryover’ effects were obvious on the hatchlings in the present study. For the most part, these were noted in brain rather than liver and included brain carbonylated protein. The effects of maternal dietary Met on the antioxidant variables measured in hatchlings did not exactly follow the effects noted earlier on maternal traits, nor the hatchability and proportion of healthy ducklings. Previous studies have investigated the effects of dietary Met on breeders, but they generally did not consider its effects on their offspring( Reference Xiao, Wang and Liu 5 , Reference Hosseini, Zaghari and Lotfollahian 8 ). Indeed, only Brun et al. ( Reference Brun, Bernadet and Cornuez 51 ) showed that maternal dietary Met affects the growth performance and lipid metabolism in the offspring of Muscovy duck breeders. Similarly, in sows fed a diet supplemented with Met in the form of dl-2-hydroxy-4-methylthiobutanoic acid, reductions in oxidative stress occurred in the intestines of their piglets( Reference Zhong, Li and Liu 52 ).
The laying performance and reproductive performance of duck breeders are mainly concerns in duck industry. In the current study, the dietary Met levels for maximising the egg weight, albumen weight, egg mass and FCR were 4·6, 4·9, 4·4 and 4·8 g/kg, respectively. In addition, diet containing 4.0 g Met/kg could attain optimal hatchability, hepatic GPX1 and Nrf2 expression, and the hepatic GPX activity in duck breeders. These results indicate that dietary Met level at 4·0–5·0 g/feed is necessary in laying duck breeders. Previous studies used quadratic regression models as a fitted model with lower P values and higher R 2 values to evaluate nutritional requirements of poultry( Reference Lu, Chang and Liao 53 ). Thus, the albumen weight and Nrf2 mRNA expression level are supposed to be more reliable indicators for the evaluation of Met requirements of laying duck breeders.
In conclusion, the present study showed that the egg weight, albumen weight, egg mass, FCR, hatchability and 1-d duckling BW of laying ducks breeders were improved by increasing dietary Met to approximately 4·0–5·0 g/kg. The increases in the egg weight and albumen weight may have reflected up-regulated expression of OVAL in the oviduct magnum of breeders by increasing dietary Met. A diet containing 4·0 g Met/kg would achieve optimal hepatic GPX1 and Nrf2 expression, maximise the activity of GPX and minimise lipid peroxidation. There was an obvious carryover effect to the embryos as the maternal dietary Met enhanced HO-1 and Nrf2 transcripts and antioxidant status, and reduced carbonylated protein in the brain of hatchlings.
Acknowledgements
The authors sincerely thank W. Bruce Currie (Emeritus Professor, Cornell University, Ithaca, NY) for his help in presentation of this manuscript.
This study was supported by the Earmarked Fund for Modern Agri-industry Technology Research System (grant no. CARS-42-13), Supporting Program for the Research of State Key Laboratory of Livestock and Poultry Breeding, Science & Technology Program of Guangdong Province (grant no. 2013A061401020), Operating Funds for Guangdong Provincial Key Laboratory of Animal Breeding and Nutrition (grant no. 2014B030301054), Supporting Program for Guangdong Agricultural Research & Development Center of Livestock and Poultry Healthy Breeding, and President Foundation of Guangdong Academy of Agricultural Sciences (grant no. 201619).
The authors’ contributions are as follows: D. R., A. M. F., L. Y. and C. Z. were responsible for the study design; D. R. conducted the animal trial, performed the sample analyses and prepared the manuscript; C. Z. and A. M. F. participated in editing of the manuscript; Q. F., C. L. and Y. W. contributed to the sample analyses. W. X., S. W. and W. C. assisted with the duck trial. All authors read and approved the final manuscript.
None of the authors has any conflicts of interest to declare.