Introduction
Species distribution models are one of the primary tools used by conservationists to investigate habitat suitability for species across landscapes (Guisan & Thuiller, Reference Guisan and Thuiller2005; Elith & Leathwick, Reference Elith and Leathwick2009). By linking habitat types and areas of human impact to species distributions, the models provide quantitative, spatially explicit information that can support conservation decision making (Guisan et al., Reference Guisan, Tingley, Baumgartner, Naujokaitis-Lewis, Sutcliffe and Tulloch2013). Species distribution models have been applied successfully to species conservation assessments (Engler et al., Reference Engler, Guisan and Rechsteiner2004), locating new populations (Fois et al., Reference Fois, Fenu, Lombrana, Cogoni and Bacchetta2015), identification of threats and evaluation of environmental change (Thuiller et al., Reference Thuiller, Richardson, Pyšek, Midgley, Hughes and Rouget2005), and identification of suitable areas for reintroduction (Rodríguez et al., Reference Rodríguez, Brotons, Bustamante and Seoane2007). The visual and geo-referenced qualities of species distribution models also make them relatively easy to interpret by non-specialists from government and industry, offering the potential to incorporate biodiversity within landscape development strategies. Considering the past application of species distribution models and their ability to predict distributions in unsampled sites, they have potential to support conservation management efforts for rare and little known species, for which data are often scarce (Guisan & Thuiller, Reference Guisan and Thuiller2005).
The Sultanate of Oman is renowned for its rich endemic flora and fauna, which arise from remarkable habitat diversity. One of these habitats is the Hajar Mountain range, an arid, subtropical mountain system that hosts unique communities of plants (Patzelt, Reference Patzelt2015), reptiles (Carranza & Arnold, Reference Carranza and Arnold2012), insects (Monks & Ross, Reference Monks and Ross2015), and mammals (Delany, Reference Delany1989). Of particular interest is the Arabian tahr Arabitragus jayakari (Plate 1), an endemic ungulate confined to the Hajar Mountain range. Categorized as Endangered on the IUCN Red List, the Arabian tahr is believed to be under threat from habitat loss and disturbance, illegal hunting, disease, and competition from livestock (Insall, Reference Insall2008). Yet because of its scarcity and the inaccessible nature of its habitat, little is known about the species, with past studies confined to descriptions based on local knowledge and animal sign (Harrison & Gallagher, Reference Harrison and Gallagher1974; Munton, Reference Munton1985; Al Majaini, Reference Al Majaini1999; Insall, Reference Insall, Fisher, Ghazanfar and Spalton1999). This lack of information has limited the development of strategies that could promote conservation of the species.
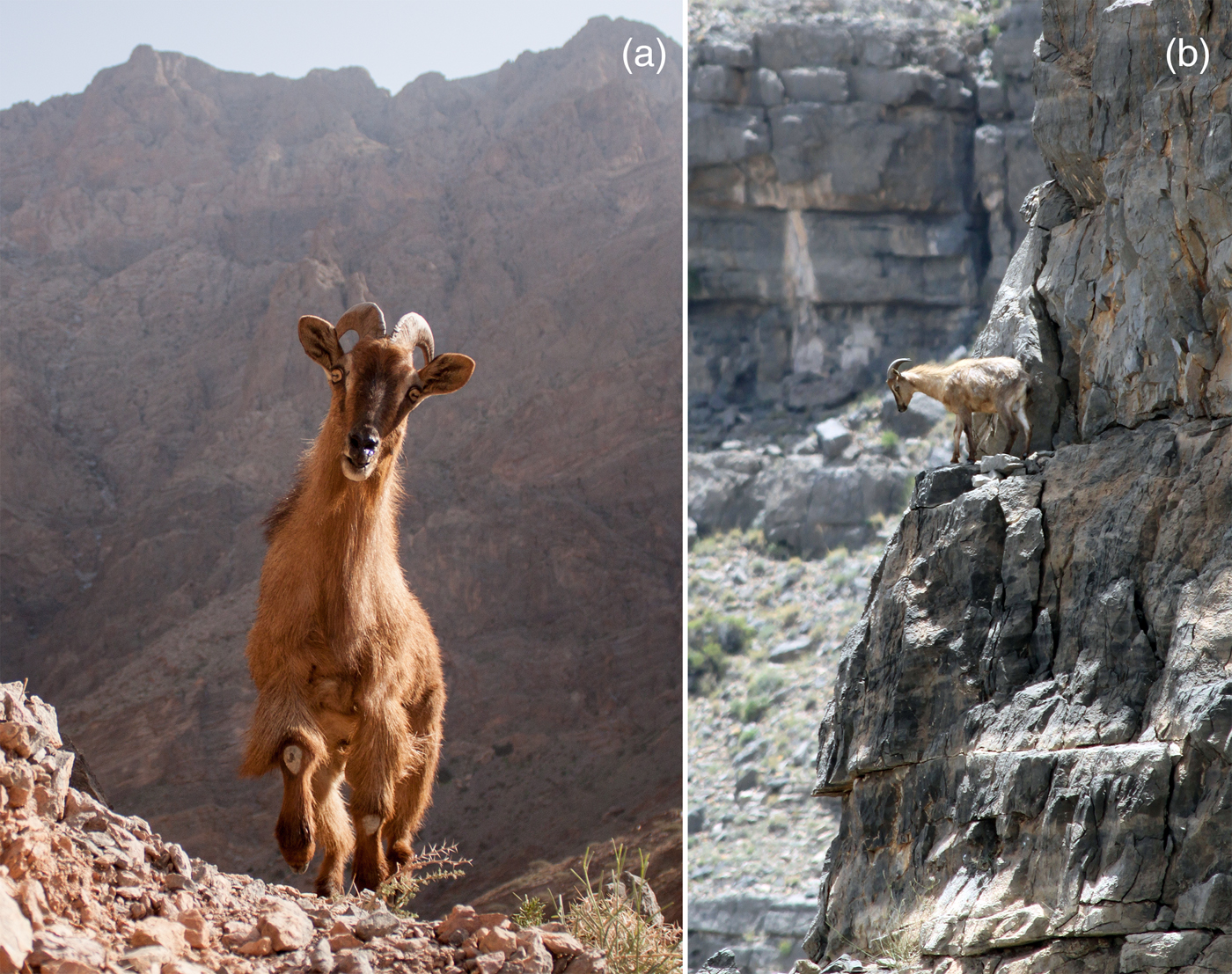
Plate 1 Photographs of a male Arabian tahr Arabitragus jayakari (a) in Wadi As Sareen Nature Reserve, with the core tahr conservation area of Jabal Aswad in the background, and (b) in typical rocky habitat of the Hajar Mountains.
As a result of the relatively recent impact of development in Oman compared to other countries, and favourable conservation policies such as the banning of hunting, the country hosts some of the largest extant populations of wildlife in the region (Fisher, Reference Fisher, Fisher, Ghazanfar and Spalton1999). However, recently there have been extensive losses of biodiversity and habitats as a result of poaching, habitat fragmentation and degradation (Fisher, Reference Fisher, Fisher, Ghazanfar and Spalton1999; Al Jahdhami et al., Reference Al Jahdhami, Al Mahdoury, Al Amri and Soorae2011; Al Hikmani et al., Reference Al Hikmani, Zabanoot, Al Shahari, Zabanoot, Al Hikmani and Spalton2015). In response, there has been a push by the government of Oman to build conservation capacity and integrate conservation with human development needs (Schrenk et al., Reference Schrenk, Neuschmid, Rathschüler and Kollarits2012; Dutton, Reference Dutton2016). Species distribution models are particularly suitable in the Omani context, given the spatial distribution and inaccessibility of wildlife populations. For little known species such as the Arabian tahr, the models have potential to make inferences from limited data sets, in a format that facilitates management decisions. We aimed to demonstrate the effectiveness of species distribution models built using camera-trapping data and occupancy models, to elucidate habitat relationships and threats, and to predict habitat occupancy of the Arabian tahr across the entire Hajar Mountain range. In so doing we provide the first landscape-scale conservation assessment of the Arabian tahr, outline its core habitats and the major threats to the species, and produce mapping outputs that identify important conservation management priorities for protection and restoration of the species.
Study area
The Hajar Mountains are a 650 km mountain range in northern Oman, extending into the United Arab Emirates (Fig. 1). The range is composed of steep, jagged mountains, canyons and dry river beds, rising steeply from the Gulf of Oman to the highest point of 3,009 m, on Jabal Shams. As elevation increases, temperature and evapotranspiration decrease, with mean annual temperatures of 18°C at 2,000 m, 25°C at 1,070 m and 29°C at sea level (Luedeling et al., Reference Luedeling, Siebert and Buerkert2007). Mean annual precipitation ranges from 75 mm in the lowlands to 400 mm in the higher reaches of the mountains (Kwarteng et al., Reference Kwarteng, Dorvlo and Kumar2009). Given the hot, arid conditions, vegetation is generally sparse, but coverage increases in drainage lines and with altitude, with the highest species richness found at 1,000–1,500 m (Ghazanfar, Reference Ghazanfar1991). Species commonly found across the Hajar Mountains include Acacia tortilis, Ficus cordata subsp. salicifolia, bushes such as Aerva javanica, and grasses such as Sporobolus spicatus. Steep rocky slopes tend to be dominated by plants such as Euphorbia larica, Echinops erinaceus, Blepharis ciliaris, and bunch grasses Aristida adscensionis. Vegetation cover is also influenced by the underlying geology, which is mostly Cretaceous limestone and ophiolites. Vegetation tends to be more common in areas underlain by limestone, as the serpentine ophiolite rocks weather to produce soils with low macronutrient and high heavy metal concentrations adverse for plant growth (D'Amico & Previtali, Reference D'Amico and Previtali2012). As the Hajar Mountains are one of the main watersheds in Oman, they have traditionally been occupied by humans. Anthropogenic threats to the Arabian tahr include poaching, and grazing of sheep and goats (Insall, Reference Insall2008). Since the early 2000s infrastructural development and improvements to the road network have resulted in habitat loss and fragmentation.

Fig. 1 (a) Probability of occurrence of the Arabian tahr Arabitragus jayakari across its Extent of Occurrence in the Hajar Mountains of Oman and the United Arab Emirates, based on camera-trap surveys conducted during 2012–2015, and protected areas for the Arabian tahr in Oman (Wadi As Sareen, Jabal Qahwan). Number 1 marks an area suitable for an Arabian tahr protected area, which could protect a geographically separate population. (b) The 2008 IUCN Red List Area of Occupancy (AOO) for the Arabian tahr (shaded), with the camera-trap survey points used in this study (dots). (c) The predicted AOO of the Arabian tahr from camera-trap surveys, based on predicted occurrence probability values > 0.28.
Methods
Camera-trap surveys
Camera traps (Bushnell Trophy Cam HD 119577C and 119466, Kansas City, USA) were used to collect data on Arabian tahr occupancy across the Hajar Mountains. Cameras were attached to rocks, 30–40 cm above ground level, and set using normal trigger sensitivity, with three photographs per event and a 5-second delay between captures. To gain inference about the entire Arabian tahr population we sampled the Hajar Mountain range using a stratified random approach. Sampling sites were generated at random using ArcGIS 10.3 (ESRI, Redlands, USA), with a 10 km separation between sampling locations, which approximates the distance between individual mountains. The closest mountain to each random location was then used as the sampling site. At each site 2–3 cameras were placed at various altitudes and habitats, separated by at least 1 km (mean 2.5 km, max. 9.3 km) to ensure stratification. Cameras were set at altitudes of 17–2,768 m. Given the rocky nature of the Hajar Mountains there were generally no distinct paths; camera traps were placed on cliff ledges, passes and the easiest passageway across slopes.
The sampling unit for the study was defined as a single camera trap placement (site) that was at least 1 km from the nearest neighbouring camera. Camera traps were operational for various periods of time but to avoid possible violation of the population closure assumption we included only data from the winter season (October–April), spanning up to 90 days (mean 72 days) per camera trap. Each 24-hour trapping period was used as a unique sampling occasion for each camera trap placement.
Environmental variables
We hypothesized that 14 covariates would predict Arabian tahr occurrence across the Hajar Mountains (Table 1), as these covariates have influenced other mountain ungulates, or have been hypothesized to influence the Arabian tahr (Munton, Reference Munton1985; Insall, Reference Insall, Fisher, Ghazanfar and Spalton1999; Gross et al., Reference Gross, Kneeland, Reed and Reich2002; Hamel & Côté, Reference Hamel and Côté2007). Other than two variables that were recorded on-site (water and goats per trap-night), variable values were compiled in a geographical information system database spanning the Hajar Mountains, using ArcGIS 10.3. The global positioning system coordinates of each camera site were recorded when the camera was placed, and the positions imported into the database. Elevation, aspect and slope maps were obtained from a 30 m resolution Advanced Spaceborne Thermal Emission and Reflection Radiometer (ASTER) digital elevation model. Elevation was also used as a surrogate for rainfall, as rainfall increases linearly with elevation across the Hajar Mountains (Kwarteng et al., Reference Kwarteng, Dorvlo and Kumar2009). We measured ruggedness (RUG) as a continuous measure of roughness and terrain complexity, derived from the surface ratio, using digital elevation model surface tools for ArcGIS (Jenness, Reference Jenness2013). We used the mean surface ratio within 50 and 750 m radius buffers from the camera location to derive the variables RUG50 and RUG750, representing different scales of influence. The Heat Load Index, indicating the influence of sunlight on surface temperature, was derived from slope, aspect and latitude at sites using the equation of McCune & Keon (Reference McCune and Keon2002). Climatic data were obtained from WorldClim (Hijmans et al., Reference Hijmans, Cameron and Parra2004) and included the mean diurnal temperature range (BIO2) and annual temperature range (BIO7) at 1 × 1 km resolution. Other climatic variables correlated with existing environmental variables and so were not included. A binary covariate was assigned to identify camera sites that were inside Arabian tahr protected areas in Wadi Sareen and Jabal Qahwan Nature Reserves, within 50 m of a water source (WAT) or within an area of ophiolite (OPH). We derived the normalized difference vegetation index from 30 m resolution Landsat 8 imagery from November 2014, and used a 150 m radius buffer from each camera to measure the mean value of the index as a measure of the influence of vegetation cover. To understand the influence of humans we measured the distance to the nearest road (RD) and village (VIL) from camera locations. The relative number of goats at each site was derived directly from camera data and standardized by counting individual goats photographed per trap-night at each site (GOAT).
Table 1 Variables used in modelling habitat occupancy of the Arabian tahr Arabitragus jayakari across its range in the Hajar Mountains (Fig. 1), and the units of the effects (for a one-unit step increase or decrease).

Occupancy modelling
During October 2012–May 2015 a total of 333 camera traps were deployed in mountainous habitat; of these we retrieved detection/non-detection data on the Arabian tahr from 300 cameras. Sampling produced encounter histories suitable for calculation of occupancy, using a method that calculates the proportion of an area occupied by a species while accounting for imperfect detection (MacKenzie et al., Reference MacKenzie, Nichols, Royle, Pollock, Bailey and Hines2006). Occupancy is one of the best means of surveying the distribution and habitat preferences of species without relying on individual markings. Following the requirements of single-season occupancy models, the occupancy status of camera sites was assumed to remain closed during the up to 90-day sample season. This means each site was either continuously occupied or unoccupied by Arabian tahr over the entire survey period (MacKenzie et al., Reference MacKenzie, Nichols, Royle, Pollock, Bailey and Hines2006). This was a reasonable assumption given the short winter survey period, and because Arabian tahr are non-migratory and maintain consistent home range areas (authors, unpubl. data). By separating cameras by habitat type and by at least 1 km we also assumed that camera sites and the detection of species at sites were independent of one another (MacKenzie et al., Reference MacKenzie, Nichols, Royle, Pollock, Bailey and Hines2006).
Models were fitted using PRESENCE 10.2 (Hines, Reference Hines2006), using a two-stage logistic regression that incorporated environmental covariates to explain differences in site occupancy and detection. The first stage described the true presence and absence of a species (ψ), and the second described detection and non-detection (ρ), conditional on the presence of a species (Kéry et al., Reference Kéry, Gardner and Monnerat2010). Only independent covariates were used in the same models, indicated by a Pearson's pair-wise correlation coefficient < 0.45. When two variables were highly correlated, we selected the one least correlated to others. Covariates were fitted with linear and quadratic effects and interactions to allow for the possibility of non-linear relationships.
Model selection
We used the Akaike Information Criterion (AIC) for model selection. All candidate models were ranked by comparison of AIC and Akaike weights (Burnham & Anderson, Reference Burnham and Anderson2002). Following the procedure of Kéry et al. (Reference Kéry, Gardner and Monnerat2010), we started with the most general model with all described effects, and kept the occurrence part of the model constant while modelling the detection probability using a backwards-elimination process. We selected parameters to drop based on indications of importance, such as overlap of the coefficients’ 95% confidence intervals with zero (Arnold, Reference Arnold2010). Following identification of the most parsimonious model structure for detection, detection was kept constant and we modelled occurrence probability.
Model fit was assessed using the goodness-of-fit test within PRESENCE 10.2 using 10,000 bootstraps (Mackenzie & Bailey, Reference MacKenzie and Bailey2004). The predictive performances of models were assessed using receiver operating characteristic curves, calculated using the application of Eng (Reference Eng2014). The receiver operating characteristic curve represents a plot of the rate of true positive predictions (sensitivity) against their equivalent false positive predictions (1, specificity) for all available occupancy values measured at sites (Fielding & Bell, Reference Fielding and Bell1997; Pearce & Ferrier, Reference Pearce and Ferrier2000). We tested the ability of each model to discriminate between sites that detected and failed to detect Arabian tahr by use of occupancy calculated at that site by the model under scrutiny. The predictive accuracy of models was assessed by comparing the area under the curve (AUC) of each receiver operating characteristic plot, which ranges from 0.5 for models with discrimination abilities expected by chance alone, to 1 for models with perfect discrimination; values of 0.7–0.9 were assumed to indicate good discrimination ability (Pearce & Ferrier, Reference Pearce and Ferrier2000).
We dealt with model selection uncertainty and the interpretation of the effects of parameters on Arabian tahr occupancy and detection by using model-averaging to calculate parameter estimates (β), occupancy (ψ) and detection (ρ) probabilities (Burnham & Anderson, Reference Burnham and Anderson2002). The relative importance of individual parameters was assessed through interpretation of their back-transformed effects and confidence intervals.
Model-averaged parameter estimates were used to construct a predictive occurrence probability map for the Arabian tahr using the ArcGIS raster calculator function. Variables measured on-site (goats per trap-night) were omitted from the calculation as we could not map their influence on occupancy in areas without these measurements. The distribution map was constructed using a pixel size of 50 m, the occupancy probability of each pixel was calculated using the equation for occupancy, equation values were obtained from geographical information system layers and the model parameter estimates. We followed the procedures of Guillera-Arroita et al. (Reference Guillera-Arroita, Lahoz-Monfort, Elith, Gordon, Kujala and Lentini2015) to estimate the area of occupancy (AOO) of the Arabian tahr, by summing site occupancy probabilities obtained from our species distribution model across the entire Hajar Mountains.
Results
For each camera site a mean of 72 trap-nights were used, with a combined sampling effort of 21,639 trap-nights. Arabian tahr were detected at 139 of the 300 camera-trap sites, giving a naïve occupancy estimate of 0.46 and a model-averaged site occupancy of 0.49 ± SE 0.29, and detection of 0.07 ± SE 0.07.
The tested occupancy models showed no lack of fit or overdispersion of data, with a c-hat value of 0.84 for our most complex model (Table 2; Burnham & Anderson, Reference Burnham and Anderson2002). Model-averaged site occupancy had a high discrimination capacity, indicated by a high AUC = 0.837 ± SE 0.023 for the full model and AUC = 0.831 ± SE 0.023 for the predictive distribution model, thus indicating correct discrimination between occupied and unoccupied sites 83.7 and 83.1% of the time (Pearce & Ferrier, Reference Pearce and Ferrier2000). Models adequately described and predicted occupancy probability at the landscape scale.
Table 2 The top-ranked models for Arabian tahr occupancy with detection kept constant, for detection with occupancy kept constant, and for models without covariates, with the number of parameters (K), the difference in Akaike Information Criterion relative to the top-ranked model (ΔAICc), the Akaike weight (Wi), the estimated over-dispersion parameter (Ĉ), and the area under the receiver operating curve (AUC).

Factors affecting occupancy and detection
As there was no effect of year on Arabian tahr occupancy or detection, the variable was excluded from final models. Model-averaged parameter estimates and odds ratios suggested that ruggedness within a 750 m radius of the site (RUG750) had the greatest effect on Arabian tahr occupancy. For every 0.1 step increase in ruggedness there was a 182% increase in the odds of occupancy (Table 3). The trend remained consistent across all measured values of ruggedness (Fig. 2). Being located inside an Arabian tahr protected area also had a strong positive influence on tahr occurrence. Whereas 83.6% of camera traps inside Arabian tahr protected areas recorded Arabian tahr, only 36.5% of cameras outside of these areas recorded the species. This corresponded to a 669% increase in the odds of tahr occupancy inside compared with outside the protected areas (Table 2). Increases in altitude had an overall negative effect on tahr occupancy despite the relationship being positive at lower altitudes. Tahr occupied areas at altitudes of 81–2,315 m. Occupancy was highest at 400–1,200 m, peaked at 800–1,000 m, and then gradually declined above 1,000 m (Fig. 2). The proximity to villages and the relative abundance of goats, which indicated increased human disturbance, both had strong negative influences on tahr occupancy. On average, each additional goat recorded per trap-night resulted in a 50% reduction in the odds of tahr occupancy. Villages had a similar impact, with each 1,000 m further from a village resulting in a 49% increase in the odds of tahr occupancy (Fig. 2).

Fig. 2 Predicted occupancy probability and observed occupancy (based on camera-trap data) of the Arabian tahr across its range (Fig. 1) in relation to four influential variables: (a) elevation, (b) ruggedness within 750 m, (c) distance to nearest village, and (d) goats per trap-night at sampling sites. Camera-trap data were collected from the Hajar Mountains of Oman during October 2012–May 2015.
Table 3 Model-averaged logistic parameter estimates (β) for Arabian tahr occupancy and detection, with 95% confidence intervals.

The annual range in temperature (BIO7) had a small effect in comparison to other parameters, with a 1°C rise in annual temperature range resulting in a 7% decrease in the odds of tahr occupancy. Several variables predicted to influence tahr occupancy showed no effect. These included the dominant rock type in the area (ophiolite or limestone) and proximity to water. Despite previous literature suggesting the Arabian tahr's preference for north-facing aspects, models showed no effect of aspect or heat load, and the proportion of camera traps detecting tahr on south-facing slopes (44.6%) was similar to those on north-facing slopes (45.2%).
The detection of Arabian tahr at camera-trapping sites was most dependent on the characteristics of the area immediately surrounding the camera. The model-averaged detection probability for a 24-hour period was 0.07 ± SE 0.07. Variables with positive influences on Arabian tahr detection included the presence of water, ruggedness within 50 m, being inside a tahr reserve, and heat load, whereas roads, increases in altitude, normalized difference vegetation index and diurnal temperature range (BIO2) contributed towards lower detection probabilities (Table 3). Increases in the number of goats encountered at the site had a strong positive influence on tahr detection, which was opposite to the effect of goats on tahr occupancy probability.
Based on the most parsimonious occupancy model, the mean site occupancy (AOO) of Arabian tahr across the 29,153 km2 Hajar Mountains was 28.1 ± SE 0.07%. However, survey data and available literature (Insall, Reference Insall, Fisher, Ghazanfar and Spalton1999; M. Chreiki, pers. comm.) suggested that several areas included within our distribution map in Oman and the United Arab Emirates are now unoccupied by Arabian tahr. Omitting these areas where tahr have been extirpated leads to an estimated AOO of 23.9 ± SE 0.08%, equivalent to 6,986 ± SE 558 km2 (Fig. 1). Our estimate is substantially lower than the current IUCN Red List map of AOO, which shows an extant area of 31,541 km2 (Insall, Reference Insall2008; Fig. 1).
Discussion
We assessed the distribution and habitat preferences of the Arabian tahr across its entire range, clarifying the species’ status and identifying conservation issues and opportunities. Tahr distribution was positively influenced by ruggedness and the protection provided by reserves, but elevation, human disturbances, including village proximity and the presence of livestock, negatively influenced Arabian tahr occupancy.
The high occupancies found in tahr protected areas demonstrated a clear positive effect of government-led protection of the species. This has been accomplished through patrolling ranger forces and restrictions on human activity in the Wadi Sareen and Jabal Qahwan nature reserves.
The Arabian tahr showed a strong affinity for rugged landscapes, which may be related to a number of advantages conferred by rugged terrain. Firstly, rugged terrain was characterized by steep, fractured mountain slopes and ravines; thus it provides excellent cover. As the need to escape and take cover from predation is known to be a strong determinant of habitat selection by ungulates (Ripple & Beschta, Reference Ripple and Beschta2004), it is likely that Arabian tahr use rugged habitats as escape terrain to evade predation (Gross et al., Reference Gross, Kneeland, Reed and Reich2002; Hamel & Côté, Reference Hamel and Côté2007). Secondly, the steep and complex nature of rugged habitat creates areas of shade. As the tahr occurs in a region that experiences high temperatures, shade is likely to be important for temperature regulation by the species. Thirdly, the diversity of climatic and habitat conditions in rugged terrain promotes plant species richness (Al Rawahi & MacClaren, Reference Al Rawahi and MacClaren2015), and thus the use of rugged habitat may also provide nutritional advantages.
The negative impact of elevation on tahr occupancy suggests an upper limit to their current distribution. Low occupancy at higher elevations may be attributable to avoidance of colder temperatures, higher rainfall, or the vegetative composition dictated by the change in environmental conditions (Ghazanfar, Reference Ghazanfar1991; Patzelt, Reference Patzelt2015). Although tahr activity was focused at elevations of c. 1,000 m, a zone which contains many of the tahr's favoured forage species (Al Majaini, Reference Al Majaini1999), the range of elevations at which tahr occur, and differences in vegetation composition found in these zones, suggests that tahr do not have highly specialized dietary requirements. Their use of lower elevations in summer and winter also suggests they are resilient to high ambient temperatures. Considering the rate of temperature increase as a result of climate change across Arabia, these broad tolerances may equip tahr with some resilience to the climatic changes forecast for the Hajar Mountain region (Al Charaabi & Al Yahyai, Reference Al Charaabi and Al Yahyai2013).
The decline in tahr occupancy in relation to the presence of villages and goats indicates negative anthropogenic impacts on the species and its current distribution. However, we found mountainous areas next to the largest cities in Oman had relatively high levels of tahr occupancy, suggesting differences in the practices of rural and city dwellers. In Oman these include partial dependency on the local environment by rural populations, most notably through livestock husbandry, whereas city dwellers generally have income-based livelihoods with little associated use/disturbance of natural habitat, resulting in a higher availability of vegetation in mountains surrounding cities. That Arabian tahr occur close to cities suggests that they can tolerate noise and other disturbances associated with urban areas as long as their habitat remains unexploited.
Goats, which are ubiquitous across the Hajar Mountains, were found to have a significant impact on tahr occupancy. This had been suspected for some time (Insall, Reference Insall, Fisher, Ghazanfar and Spalton1999), but our results provide proof of this impact. The positive relationship between goat numbers and tahr detection probability indicated overlapping habitat preferences of the two species, while lower tahr occupancy suggests that goats reduce patch quality. The mechanisms of this relationship merit further attention, but it seems likely that the combination of dietary overlap (Al Majaini, Reference Al Majaini1999) and slow recovery of vegetation in arid conditions following grazing reduced patch quality by depletion of forage (e.g. Mishra et al., Reference Mishra, Van Wieren, Ketner, Heitkönig and Prins2004).
Several of the modelled variables showed no effect on tahr occupancy. One was north-facing aspects, which are commonly cited as a critical habitat of tahr, given the influence of aspect on temperature and vegetation availability (Munton, Reference Munton1985; Insall, Reference Insall, Fisher, Ghazanfar and Spalton1999). Our data indicated no relationship between Arabian tahr and northern slopes, but rather suggested that Arabian tahr gain access to shade and the associated forage through their selection of rugged terrain.
Distribution and conservation status
Our predictive distribution map showed a substantial decrease in Arabian tahr distribution, in comparison to the current IUCN Red List. The reduction in the species’ distribution is of great concern and reflects population extirpation since the early 2000s, but also an improvement in methodology providing a more accurate picture of the species’ distribution.
The notable absence of tahr in several areas with high predicted occurrence probability also suggests the existence of other characteristics or impacts not considered in our models. Our omission of illegal hunting of Arabian tahr, past and present, is probably the most important factor explaining absence in apparently suitable habitats. In the past, Arabian tahr were hunted as an important protein source for local people. Since 1976 it has been illegal to kill or capture Arabian tahr in Oman, but the steep terrain associated with the species makes this difficult to enforce. Poaching still occurs in Oman, as indicated by the periodic prosecution of poachers. However, given its illegality, it is difficult to accurately estimate the scale or effect of poaching on the species’ distribution.
Conservation and management recommendations
We now have an improved understanding of factors affecting the distribution of the Arabian tahr. The baseline information provided will facilitate better conservation planning to improve the long-term prospects and recovery of the species. Negative impacts caused by anthropogenic disturbances are the main cause of tahr absence in areas that otherwise appear to be suitable, and of these the displacement of tahr by domestic goats is the most serious. Domestic goats are reared across the Hajar Mountains, and as goats and tahr have similar habitat preferences, any future attempts to protect or restore Arabian tahr habitat should first consider how livestock populations can be reduced or controlled. The removal of feral goats is complicated by the difficulty in differentiating domestic from feral populations, and therefore community engagement will be an important aspect of any removal plan. For owned, herded livestock, the initiation of local incentives to restrict goats to less rugged, flatter areas to partition goats from core Arabian tahr habitats may be the most effective approach.
The high tahr occupancies found within Arabian tahr protected areas demonstrate the positive impacts of government-led protection in Oman. Considering these benefits, creation of more protected areas could bolster tahr conservation in the region. As the south-eastern Hajar mountains are already well protected, suitable areas identified by our surveys lying between Al Batinah North and Al Buraimi Governates (Fig. 1), northern Oman, should be assessed for their potential role in protecting a geographically separate population. This strategy could establish a regional source population of Arabian tahr that could reinvigorate the surrounding landscape through dispersal.
Several areas with high occurrence probabilities currently lack viable tahr populations. If disturbances can be identified and removed from these areas, they are potential sites for restoration and captive-bred reinforcement of the Arabian tahr population. In areas of high suitability in the eastern United Arab Emirates, which have poor connectivity with viable Arabian tahr populations, reinforcement through the release of captive-bred tahr may be the only means to restore populations. However, surveys of these areas are warranted, to investigate whether small remnant populations still exist. In Oman, primary efforts should focus on threat removal and maintenance of habitat connectivity to encourage natural dispersal, but where this is impossible reinforcement using captive tahr is a viable alternative.
Our findings regarding the status and requirements of the Arabian tahr will inform conservation of the species in the wild. Continued population extirpation and the limited, fragmented distribution of the Arabian tahr suggest that its Endangered status should be retained, and that conservation planning and action is required to prevent its extinction in the wild. As a first step our species distribution modelling is a useful spatial planning tool that can identify conservation opportunities, and potential conflicts between human development and tahr conservation.
Acknowledgements
This study was funded and facilitated by the National Field Research Centre for Environmental Conservation, the Office for Conservation of the Environment, Diwan of Royal Court, Oman, and by the Oman Earthwatch Programme. We thank Yasser Al Salami, Dr Saif Al Shaksi and Dr Mohammed Al Balushi for providing permissions and support for the project, and Dr Roderic Dutton and Nigel Winser for their support. We thank Steve Sharland, Ryan Clarke, Huw Goodall, Joshua Neve, Herve Nuttall, Chris Jones and Gareth Whittington-Jones for assistance in the field.
Author contributions
SR designed, managed and undertook camera-trap data collection and collation, conceptualized the article, undertook the data analysis and co-wrote the article. MHAJ and HAR undertook camera-trap data collection and co-wrote the article.
Biographical sketches
Steve Ross works on wildlife ecology, monitoring, behaviour, habitat selection and distribution related to wildlife management and conservation. He also works in support of conservation education, ecological training and mentoring, and is a member of the IUCN Cat Specialist and Caprinae Specialist Groups. Mansoor Al Jahdhami works on protected area management, research supervision and administration. He has broad interests in wildlife ecology related to wildlife management, captive management and reintroduction, monitoring and field data collection technologies, and is a member of the IUCN Conservation Breeding Specialist Group. Haitham Al Rawahi’s work is currently focused on the Arabian tahr. His interests include the use of camera trapping and satellite tagging techniques to investigate wildlife behaviour and distribution, and for biodiversity surveying and monitoring.