Introduction
Several species of the genus Contracaecum Railliet & Henry, 1912 have been reported around the world in many species of fish-eating birds, including those belonging to the family Phalacrocoracidae (Abollo et al., Reference Abollo, Gestal and Pascual2001). Their life cycle is heteroxenous, involving piscivorous birds or pinnipeds (definitive hosts), small crustaceans (first intermediate host) and fish (intermediate hosts). Birds are infected by eating fish containing third-stage larvae (L3), and the fish acquire them by ingesting other fish and/or crustaceans containing second-stage larvae or L3 (Anderson, Reference Anderson2000). Adults are found parasitizing the intestine and proventriculus of the definitive host, where they often cause pathological lesions (Garbin et al., Reference Garbin, Mattiucci, Paoletti, González-Acuña and Nascetti2011; Violante-González et al., Reference Violante-González, Monks, Gil-Guerrero, Rojas-Herrera, Flores-Garza and Larumbe-Morán2011).
The Neotropical cormorant Nannopterum brasilianus (Gmelin, 1789) (syn. Phalacrocorax brasilianus) (Suliformes: Phalacrocoracidae) occurs from Patagonia to the coast of Texas (Fedynich et al., Reference Fedynich, Pence and Bergan1997; Amato et al., Reference Amato, Monteiro and Amato2006; Conde-Tinco & Iannacone, Reference Conde-Tinco and Iannacone2013, Kennedy & Spencer, Reference Kennedy and Spencer2014). This bird inhabits freshwater and marine environments and is the only one within the family found throughout the Neotropical region (Kalmbach & Becker, Reference Kalmbach and Becker2005).
Five species of Contracaecum have been reported parasitizing N. brasilianus: Contracaecum rudolphii Hartwich, 1964 (syn. C. spiculigerum) in Brazil, Chile, Cuba and USA (Baruš, Reference Baruš1966; Fedynich et al., Reference Fedynich, Pence and Bergan1997; Torres et al., Reference Torres, Valdivieso, Schlatter, Montefusco, Revenga, Marín, Lamilla and Ramallo2000; Amato et al., Reference Amato, Monteiro and Amato2006); Contracaecum multipapillatum Drasche, 1882 in Mexico (Vidal-Martínez et al., Reference Vidal-Martínez, Osorio-Sarabia and Overstreet1994; Violante-González et al., Reference Violante-González, Monks, Gil-Guerrero, Rojas-Herrera, Flores-Garza and Larumbe-Morán2011); Contracaecum australe Garbin, Mattiucci, Paoletti, González-Acuña and Nascetti, 2011 in Chile and Argentina (Garbin et al., Reference Garbin, Mattiucci, Paoletti, González-Acuña and Nascetti2011; Biolé et al., Reference Biolé, Guagliardo, Mancini, Tanzola, Salinas and Morra2012); Contracaecum travassosi Gutierrez, 1943 in Uruguay (Lent & Freitas, Reference Lent and Freitas1948); and Contracaecum caballeroi Bravo-Hollis, 1939 in Uruguay (Lent & Freitas, Reference Lent and Freitas1948).
In this study, we describe a new species of Contracaecum using light and scanning electron microscopy (SEM) for adults and fourth-stage larvae (L4) found in N. brasilianus and L3 found in the freshwater fish Hoplias argentinensis Rosso, Mabragaña, González-Castro, Bogan, Cardoso, Mabragaña, Delpiani & Díaz de Astarloa, 2018, both from the province of Córdoba, Argentina. Additionally, molecular phylogenetic data were used to determine its relationships within the genus.
Material and methods
Parasite collection
Adult nematodes and L4 were collected from the intestine and proventriculus of two specimens of N. brasilianus, and L3 specimens were collected from the intestine of one specimen of H. argentinensis from a shallow Pampean lake in central Argentina (33°25′S, 62°54′W), between September and December 2017. Birds were transported to a field laboratory where they were weighed and necropsied. During necropsy, their entire digestive tracts were removed and analysed. The contents were washed with water over a sieve with mesh of 0.25 mm and the sediment was placed in Petri dishes to collect nematodes. The fish specimen was kept in an ice chest to ensure good morphological condition of the parasites and to protect them during transport to the Pharmacology Laboratory of Universidad Nacional de Río Cuarto, where the specimen was weighed, measured and subsequently necropsied, through a ventral incision from the anal opening to the operculum line to expose the body cavity. All internal organs were individualized and placed in Petri dishes containing saline solution (0.65% sodium chloride), and subsequently examined with the aid of a stereoscopic microscope to collect the parasites. The isolated nematodes were fixed and stored in 70% ethanol.
Morphological study
Adult nematodes and L4 collected from N. brasilianus and L3 collected from H. argentinensis were morphologically examined. Later, the anterior and posterior parts of the specimens were clarified in glycerine. Measurements were taken in millimetres (means followed by the range in parentheses). Morphological analyses were conducted using an Olympus BX51 light microscope (Tokyo, Japan). Nematode identification was made according to keys of Anderson (Reference Anderson2000) and Luque et al. (Reference Luque, Aguiar, Vieira, Gibson and Santos2011). Holotype, allotype and paratypes were deposited in the Helminthological Collection of the Instituto Oswaldo Cruz, Rio de Janeiro, Brazil (CHIOC).
For SEM analysis, one adult male and female, one L4 and one L3 were dehydrated through a graded ethanol series, dried in hexamethyldisilazane, coated with gold and examined in a JEOL JSM-740 1 F, at an accelerating voltage of 4 kV. The samples were mounted on aluminium stubs, coated with a 20 nm layer of gold and examined with a JEOL JSM 6390LV scanning electron microscope (operating at 15 kV) (JEOL, Akishima, Tokyo, Japan) at the Rudolf Barth Electron Microscopy Platform of Instituto Oswaldo Cruz, Rio de Janeiro.
Molecular and phylogenetic analyses
The middle part of two adult nematodes, one L4 and one L3 were separated from the rest of the body and used to characterize genetically the individual specimens by sequencing of the mitochondrial (mt) DNA cytochrome c oxidase subunit II (cox-2) gene. Genomic DNA was isolated using the phenol/chloroform protocol as in Billings et al. (Reference Billings, Yu, Teel and Walker1998). The cox-2 region of the mtDNA was amplified using the primers 211 (forward 5′ TTTTCTAGTTATATATAGATTGRTTTYAT 3′) and 210 (reverse 5′CACCAACTCTTAAAATTATC 3′) (Nadler & Hudspeth, Reference Nadler and Hudspeth2000). Polymerase chain reaction (PCR) reactions were performed in a volume of 25 μl with 20 mM of Tris hydrochloride (Tris-HCl) at pH 8.4; 50 mM of potassium chloride (KCl); 250 lM of each phosphated deoxyribonucleotides (dNTPs); 1.5 mM of magnesium chloride (MgCl2); 0.5 lM of each oligonucleotide primer; 1.25 U of Platinum Taq DNA polymerase (Invitrogen, Carlsbad, California, USA); and 2 μl of genomic DNA. PCR was performed using the cycling parameters specified in previous reports (Zhu et al., Reference Zhu, Gasser, Podolska and Chilton1998; Nadler & Hudspeth, Reference Nadler and Hudspeth2000). PCR products were visualized with Sybergreen (Invitrogen, Eugene, Oregon, USA) staining before electrophoresis on 1.5% agarose gels. The amplified products were purified with Exo-SAP-IT Kit (GE Healthcare Life Sciences, Grens, Switzerland) following the manufacturer's instructions, sequenced using an ABI PRISM BigDye Terminator Cycle Sequencing Ready Reaction Kit (Applied Biosystems-Perkin Elmer, Waltham, MA, USA) in a MegaBACE sequencer (GE Healthcare Life Sciences). All molecular analyses were performed at the Laboratory of Molecular Biology from the Universidade Federal Rural of Rio de Janeiro.
To examine the phylogenetic relationships, the nucleotide sequences obtained in this study and those retrieved from GenBank were aligned with the CLUSTAL W algorithm of the Bioedit Package (Thompson et al., Reference Thompson, Higgins and Gibson1994; Hall, Reference Hall1999). Sequences of Toxascaris leonina (NC023504) and Ascaris lumbicoides (AF179907) were used as the outgroups. The best evolutionary model for cox-2 gene was inferred by the Bayesian inference (BI) criterion using MEGA 7.0 software for both analysis, maximum likelihood (ML) and BI as optimality criterion using the Hasegawa–Kishino–Yano model plus gamma distribution and invariable sites (HKY) + G + I (Tamura et al., Reference Tamura, Peterson, Peterson, Stecher and Nei2011). The same software was used for construction of genetic distances using Kimura two parameters (Kimura, Reference Kimura1980). The statistical support for the cox-2 tree was determined by a heuristic search with 1000 bootstrap replicates (Guindon & Gascuel, Reference Guindon and Gascuel2003) and posterior probability (PP) for BI run in MrBayes 3.1.2 (Huelsenbeck & Ronquist, Reference Huelsenbeck, Ronquist and Nielsen2005) with the uncorrelated relaxed clock (Drummond et al., Reference Drummond, Ho, Phillips and Rambaut2006) and the conditioned reconstructed process (Yule process; Gernhard, Reference Gernhard2008). The Markov Chain Monte Carlo simulations were run for 89 generations and sampled at every 85 generations, with a burn-in of 10%, in BEAST version 1.8.4 (Drummond & Rambaut, Reference Drummond and Rambaut2007). The nodal support of the topology of each gene was calculated by bootstrap replication and the PP with a cut-off of 50%.
The phylogenetic analyses of the ML and BI trees were done with the mtDNA cox-2 of larvae (L3 and L4) and adults of Contracaecum sp. Sequences obtained were deposited at GenBank under the accession numbers MT304462 (adult), MT304463 (L4) and MT304464 (L3).
Results
Contracaecum jorgei n. sp.
Description
General morphology. Body robust, filiform with fine transversal cuticular striations along the body, more evident on the anterior and posterior region. Interlabia subtriangular in shape, distal tip not bifurcate and the same length as the lips with one shallow apical notch (figs 1B and 2C). Lips with two conspicuous and lobed auricles, each with two prominent sensory pits at external end (fig. 2C). Dorsal lip larger than ventrolateral lips with two sublateral double papillae, each ventrolateral lip with one small externo-lateral papilla with irregular cuticular ornamentation and separate pore-like opening of amphid in addition to large double papilla (fig. 2C, D). Conspicuous cephalic collar with V-shaped lateral region without striations (fig. 2A, C). Oesophagus with reduced globular ventriculus (fig. 1A). Excretory pore at base of lips. Deirids slightly anterior to the nervous ring (figs 1A and 2A, B), rounded shape, not visible on all specimens. Ventriculus with posterior appendix (fig. 1A), intestinal caecum well developed, longer than ventricular appendix with final portion rounded (fig. 1A). Male and female presenting conical tail with pointed tip (figs 1C, D and 2E, F).
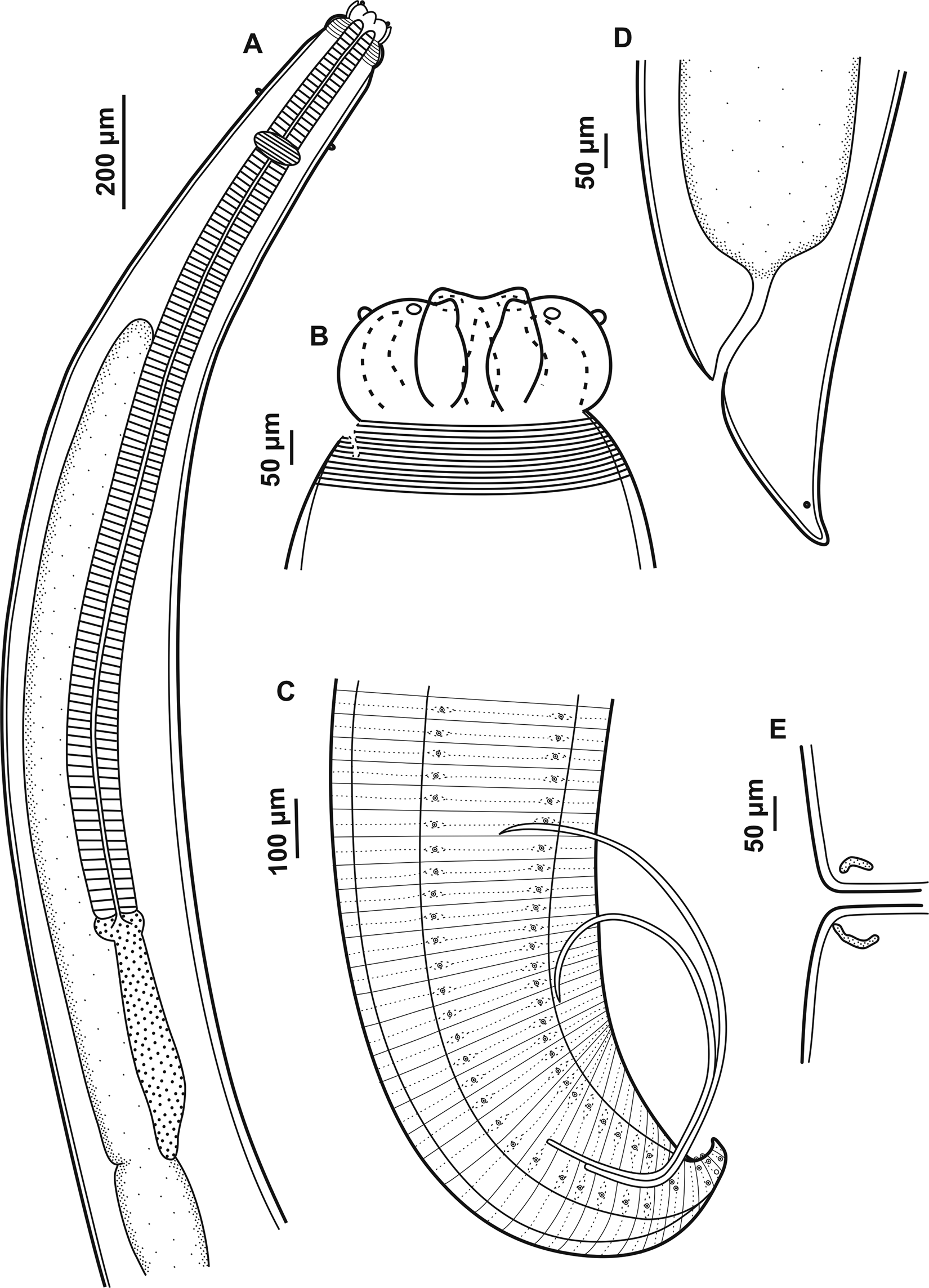
Fig. 1. Adults of Contracaecum jorgei n. sp. (A) Anterior end, dorsoventral view showing lips, cephalic collar, cephalic papilla, nerve ring, oesophagus, intestinal cecum, ventriculus and ventricular appendix. (B) Dorsal and ventrolateral lips, interlabia and cephalic collar. (C) Male, posterior end showing pre- and postcloacal papilla distribution, spicules and cloaca. (D) Female, posterior end showing cloaca and phasmid. (E) Female, median region of the body, vulva.
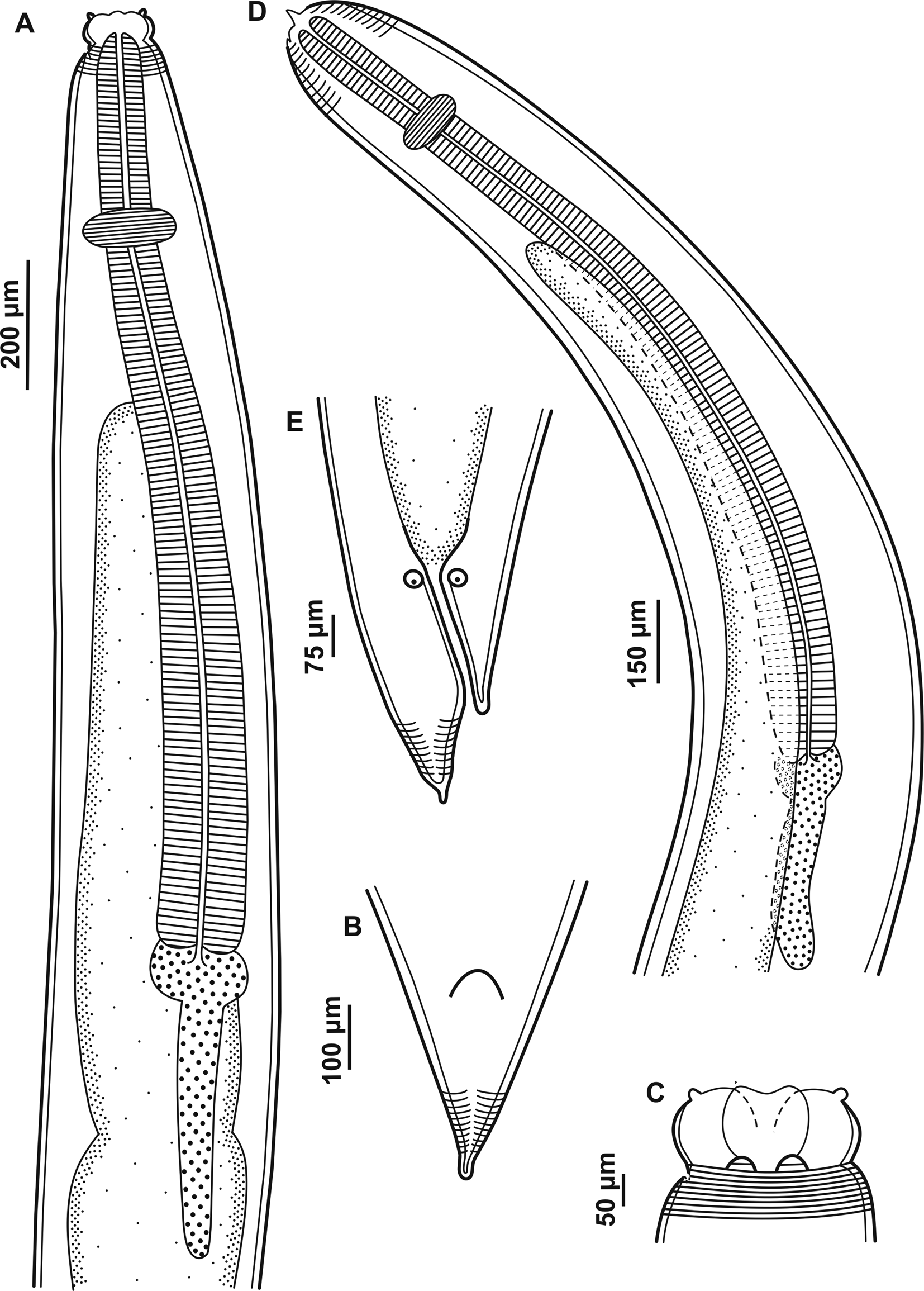
Fig. 2. Larvae of Contracaecum jorgei n. sp. (A) Fourth larval stage, anterior end, lateral view showing cephalic collar, cephalic papilla, nerve ring, oesophagus, intestinal cecum, ventriculus and ventricular appendix. (B) Fourth larval stage, posterior end showing a small projection with cuticle striated transversely. (C) Fourth larval stage, dorsal and ventrolateral lips, cephalic collar and cephalic papilla. (D) Third larval stage, anterior end, lateral view showing larval tooth, cuticle striated transversely, nerve ring, oesophagus, intestinal cecum, ventriculus and ventricular appendix. (E) Third larval stage, posterior end showing a small projection with cuticle striated transversely.
Male. Based on holotype and eight paratypes. Body length 14.31–20.98 (15.95), width at the intestinal caecum level 0.29–0.59 (0.38). Distance from anterior end to nerve ring 0.29–0.46 (0.37). Distance from anterior end to deirids 0.29 (n = 1). Oesophagus length 2.01–3.88 (2.62) and width 0.09–0.19 (0.12). Intestinal caecum length 1.19–3.32 (1.94) and width 0.07–0.15 (0.09). Length of intestinal caecum to body length ratio 8.00–15.83%. Ventriculus length 0.03–0.09 (0.05) and width 0.04–0.07 (0.06). Ventricular appendix length 0.29–0.57 (0.40) and width 0.04–0.09 (0.06). Ventricular appendix length representing 1.82–3.41% of body length. Tail length 0.15–0.21 (0.17). Caudal extremity presenting 26 precloacal pairs of papillae equidistant, forming two subventral lines extending anteriorly (figs 1C and 2F). Six postcloacal pairs of papillae: the first pair of subventral proximal papillae posterior to cloaca, the second pair of large paracloacal papillae (the pair of paracloacal papillae are asymmetrically displaced to the first pair proximal, first pair more anterior than the second pair) and four distal pairs of papillae (two subventral pairs and two sublateral pairs) (fig. 2I). One pair of phasmids situated between the sublateral pairs of papillae. Median plaque (median papilla) barely visible on anterior cloaca rim (fig. 2H). Spicules are subequal, distal tip pointed (figs 1C and 2G). Right and left spicule length 2.28–3.63 (2.81) (n = 4) and 2.03–3.18 (2.61) (n = 4), representing 13.47–19.72% and 13.17–17.28% of total body length, respectively.
Female. Based on allotype and five paratypes. Body length 15.25–18.77 (16.64) and width at intestinal caecum level 0.32–0.50 (0.40). Distance from anterior end to nerve ring 0.30–0.39 (0.36). Deirids were not visualized. Oesophagus length 2.50–3.81 (2.96) and width 0.08–0.12 (0.10). Intestinal caecum length 1.60–2.10 (1.90) and width 0.07–0.15 (0.10). Length of intestinal caecum to body length ratio 10.53–11.18%. Ventriculus length 0.02–0.07 (0.04) and width 0.02–0.07 (0.05). Ventricular appendix length 0.40–0.61 (0.49) and width 0.07–0.09 (0.07).Ventricular appendix length representing 2.63–3.26% of body length. Vulva in anterior half of body (fig. 1E). Distance from anterior end to vulva 6.57–8.48 (7.86). Ratio of vulva to body length 43.11–45.17%. Tail length 0.17–0.25 (0.22). One pair of phasmids on the tail end (figs 1D and 2E).
L4. Based on six paratypes. Large-sized nematodes white or yellow-white in colour. Cuticle striated transversely and more distinct at the extremities of the body (fig. 3A–C). Cephalic extremity rounded with three well-developed lips (one dorsal and two ventrolateral) (figs 3C and 4A). Lips with two conspicuous and lobed auricles, absence of interlabia (fig. 4A). Lips smooth, without cuticular and auricular striations, presenting cephalic papillae on each ventrolateral lip, with a single oval papilla and lateral amphid; well-developed cephalic collar. Body length 14.01–16.67 (15.17) and width at the caecum level 0.21–0.52 (0.30). Excretory pore located between the ventrolateral lips, difficult to visualize. Deirids not visible. Distance from anterior end to nerve ring 0.28–0.42 (0.32). Oesophagus length 1.99–2.91 (2.24) and width 0.08–0.12 (0.09) (fig. 3A). Intestinal caecum length 1.31–2.26 (1.56) and width 0.08–0.17 (0.11) (fig. 3A). Length of intestinal caecum to body length ratio 9.35–13.57%. Oval ventriculus length 0.05–0.10 (0.08) and width 0.05–0.13 (0.10) (fig. 3A). Ventricular appendix length 0.31–0.50 (0.42) and width 0.05–0.12 (0.07) (fig. 3A). Length of ventricular appendix representing 2.27–2.99% of body length. Conical tail with pointed tip with 0.10–0.18 (0.14) in length (figs 3B and 4B).

Fig. 3. Scanning electron microscopy of adults of Contracaecum jorgei n. sp. (A) Male, anterior end, lateral view showing cephalic collar and deirid. (B) Detail showing deirid. (C) Female, anterior end showing dorsal and ventrolateral lips, interlabia (arrow) and sensory pit (*). (D) Female, ventrolateral lip showing one large double papilla (arrow), one externo-lateral papilla (*) and amphid (a). (E) Female, posterior end showing conical tail with pointed tip. (F) Male, posterior end showing precloacal and postcloacal papillae, spicules and conical tail with pointed tip. (G) Distal spicule end, lateral view. (H) Male, ventral view posterior end showing precloacal papillae, postcloacal papillae and median papillae (arrow). (I) Male, ventral view showing postcloacal papillae. Abbreviations: d, deirid; p, phasmid; a1, proximal papilla pair; a2, paracloacal papilla pair; a3 and a4, distal subventral papillae; a5 and a6, distal sublateral papillae.
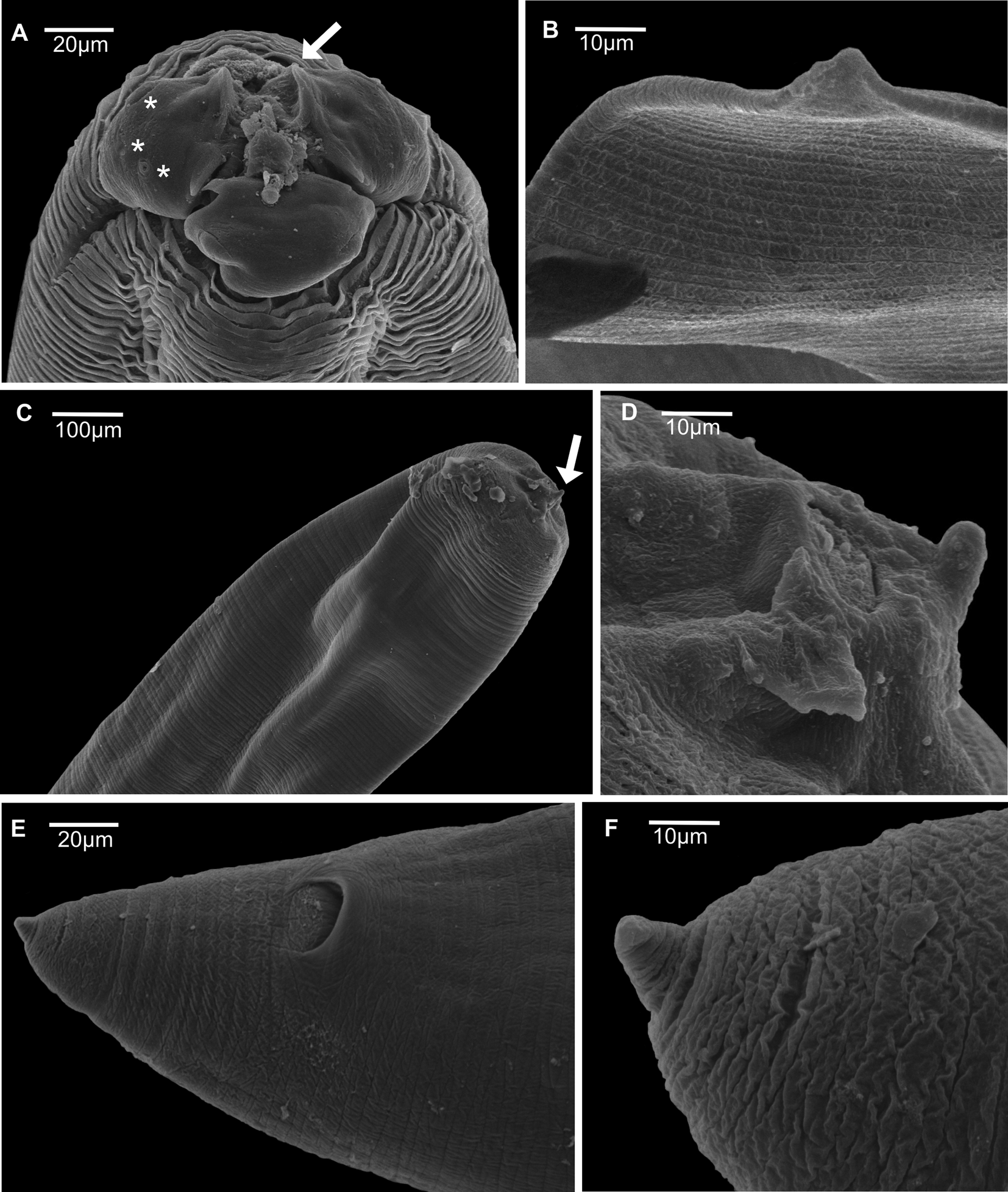
Fig. 4. Scanning electron microscopy of larval stages of Contracaecum jorgei n. sp. (A) Fourth-stage larvae, anterior end, lateral view showing cephalic extremity rounded with three well-developed lips (one dorsal and two ventrolateral), auricles (arrow) and cephalic papillae of ventrolateral lip. (B) Fourth-stage larvae, posterior end showing conical tail with pointed tip. (C) Third-stage larvae, anterior end, lateral view showing cephalic extremity rounded with one lip with a small larval tooth (arrow). (D) Third-stage larvae, detail showing larval tooth. (E) Third-stage larvae, posterior end showing cloacal opening. (F) Third-stage larvae, detail showing conical tail with pointed tip.
L3. Based on four paratypes. Medium-sized nematodes white in colour with cuticle striated transversely (fig. 4C). Body length 11.10–12.23 (11.56) and width at the caecum level 0.24–0.39 (0.29). Cephalic extremity rounded with three underdeveloped lips, one lip with a small larval tooth (figs 3D and 4C, D). Excretory pore situated below the ventral cephalic tooth 0.04–0.05 (0.05) in length (fig. 3D). Nerve ring located 0.18–0.28 (0.25) from the anterior end (fig. 3D). Oesophagus length 1.63–1.89 (1.76) and width 0.06–0.16 (0.10) (fig. 3D). Ventriculus small and oval, length 0.03–0.05 (0.04) and width 0.04–0.06 (0.05) (fig. 3D). Intestine distinct and dark. Ventricular appendix length 0.30–0.37 (0.34) and width 0.04–0.07 (0.06) (fig. 3D). Length of ventricular appendix representing 2.76–3.07% of body length. Intestinal caecum longer than ventricular appendix, length 0.98–1.30 (1.08) and width 0.10–0.12 (0.12). Length of intestinal caecum representing 8.86–11.57% of body length. Posterior extremity with two anal glands (fig. 3E). Conical tail with pointed tip with 0.11–0.13 (0.13) in length (figs 3E and 4E, F).
Taxonomic summary
Type host. Nannopterum brasilianus (Gmelin, 1789) (Suliformes: Phalacrocoracidae).
Site of infection. Intestine and proventriculus of N. brasilianus and intestine of H. argentinensis.
Type locality. Pampean shallow lakes, Unión Department, province of Córdoba, Argentina (33°25′S, 62°54′W).
Prevalence. 50% (one infected/two host specimens examined) in N. brasilianus; 100% (one infected/one host specimen examined) in H. argentinensis.
Intensity. 22 in N. brasilianus and four in H. argentinensis.
Specimens deposited. Holotype (adult male specimen from N. brasilianus, CHIOC number 38925a). Allotype (adult female specimen from N. brasilianus, CHIOC number 38925b). Paratypes:3 adult males and 2 adult females (CHIOC number 38925c); 3 L4 from N. brasilianus (CHIOC number 38925d); and 2 L3 from H. argentinensis (CHIOC number 38926).
This article was registered in the Official Register of Zoological Nomenclature (ZooBank) as: urn:lsid:zoobank.org:act:1D6178CA-533C-417C-8F27-53E4E72CC3E9.
Etymology. The new species is named in honour of Antônio Jorge de Andrade Sardella, father of the first author.
Molecular and phylogenetic analyses
Genetic sequences from Contracaecum jorgei n. sp. were generated (519 bp). Pairwise/Basic Local Alignment Search Tool (BLAST)/National Center for Biotechnology Information (NCBI) comparisons with the GenBank mt cox-2 gene data set confirmed the species as the genus Contracaecum, but not the species identification with any Contracaecum spp. available. Intraspecific genetic distance of C. jorgei n. sp. sequences ranged from 0.000 (between L3 and L4) to 0.004 (between adult and L3; between adult and L4). The interspecific distances between C. jorgei n. sp. and the species of the nearest clade were 0.105–0.107 (Contracaecum overstreeti) and 0.118 (Contracaecum gibsoni).
Tree topologies produced with different optimality criteria (ML and BI) were similar, with little variation in node support values (fig. 5). The sequences belonging to L3, L4 and adult of C. jorgei n. sp. were similar and grouped forming a well-supported clade (ML = 100%; Bayesian posterior probabilities (BPP) = 100%). Contracaecum jorgei n. sp. formed a sister group with C. gibsoni and C. overstreeti that was well supported (ML = 65%; BPP = 96%).
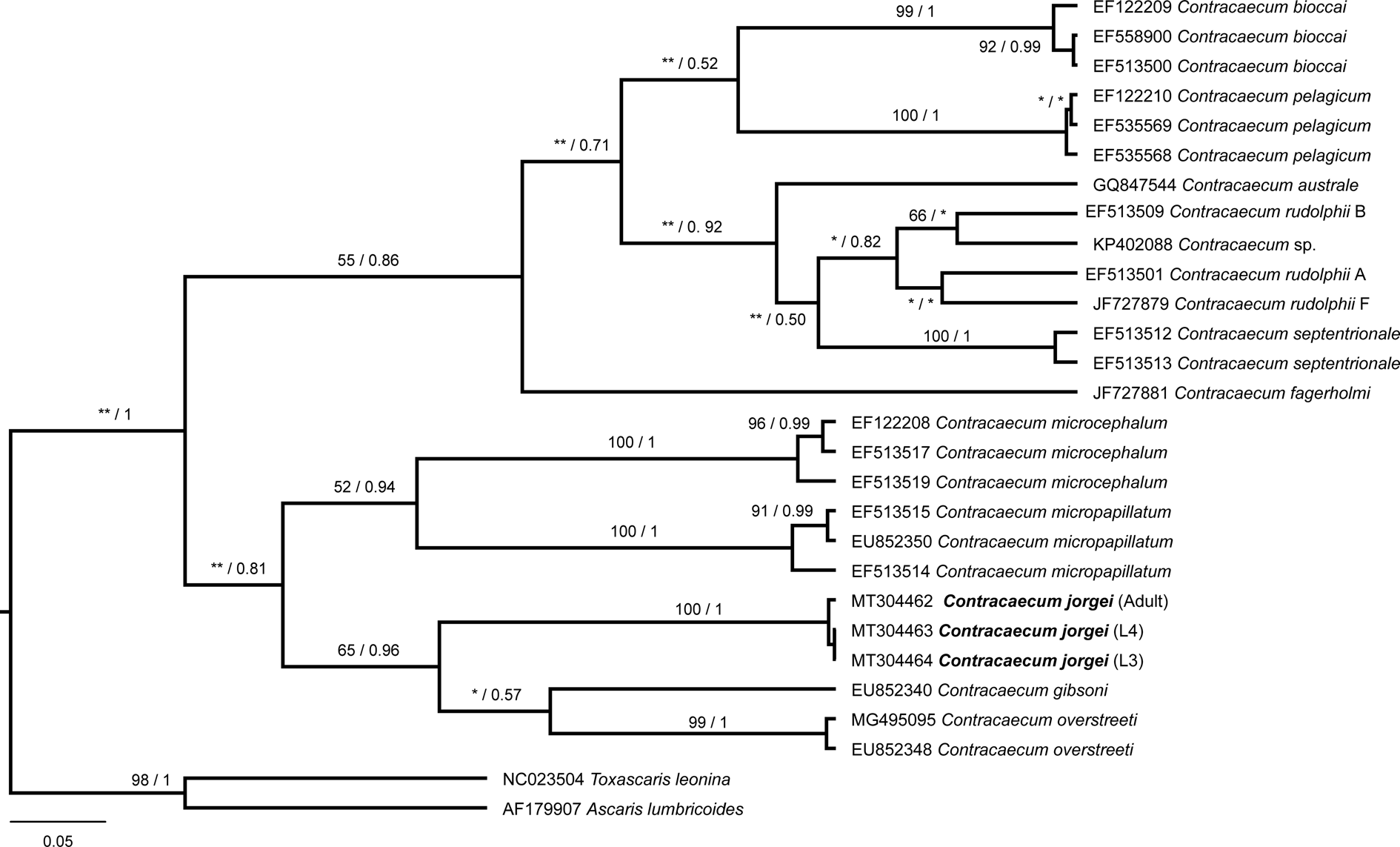
Fig. 5. Phylogenetic relationships within the Contracaecum genus based on the partial fragment of cytochrome oxidase II gene. The tree was inferred by using the maximum likelihood (ML) and Bayesian inference (BI) with the HKY + I + G model. The nodal support is described at the left by bootstrap replicates and at the right by posterior probability to each node represented. *Low nodal support in ML or BI; **incongruence between ML and BI. The scale bar represents the number of substitutions per site.
Discussion
The proposed new species belongs to the genus Contracaecum according to the following morphological characteristics: oesophagus provided with ventriculus, presence of posterior ventricular appendix, anterior caecum and excretory pore situated at the level of lip bases, and adults presenting interlabia (Moravec, Reference Moravec1998).
When comparing C. jorgei n. sp. with the other species described from N. brasilianus, the species C. rudolphii, C. australe and C. travassosi are the most similar regarding the morphometry of the oesophagus, intestinal caecum and number and distribution of caudal papillae; however, they can be distinguished by the size of spicules. The new species has subequal and shorter spicules than C. australe and C. travassosi, which have equal and long spicules (9.60–15.88 mm and 7.70–11.10 mm, respectively). Moreover, C. rudolphii can be distinguished from the new species by having unequal and larger spicules (right 4.50–7.50 mm and left 5.90–8.20 mm), whereas C. multipapillatum presents equal and smaller spicules (0.89–1.11 mm and 0.90–1.09 mm) than the new species (right 3.63–2.28 mm and left 3.18–2.03 mm) and a greater number of caudal papillae (32–93 in C. multipapillatum; 61 in C. caballeroi and 32 in C. jorgei n. sp.). In addition, C. rudolphii, C. australe and C. caballeroi can be separated from the new species by the shape of the interlabia and arrangement of cephalic papillae. Contracaecum rudolphii and C. australe present bifurcation in the distal end of the interlabia, two double papillae on the dorsal lip, one double papilla and one amphid in each ventrolateral lip, while C. caballeroi possesses two large papillae in the dorsal lip and two papillae in each ventrolateral lip (one large ventral and one smaller lateral). In contrast, C. jorgei n. sp. has an interlabia without bifurcation with the same length as the lips and one additional small papilla in each ventrolateral lip. Furthermore, C. multipapillatum and C. caballeroi are differentiated from C. jorgei n. sp. by having interlabia smaller than the lips, longer length (20.63–32.34 mm in C. multipapillatum; 24.29–26.97 mm in C. caballeroi; 14.31–20.98 mm in the new species) and longer ventricular appendix (0.47–0.69 mm; 0.51–0.61 mm vs. 0.29–0.57 mm in the new species). Additionally, C. multipapillatum has a longer oesophagus (2.60–4.62 mm vs. 2.01–3.88 mm in the new species) and ventriculus (0.12–0.23 mm vs. 0.03–0.09 mm in the new species), while C. caballeroi has a conical tail without pointed tip (Lent & Freitas, Reference Lent and Freitas1948; Amato et al., Reference Amato, Monteiro and Amato2006; Garbin et al., Reference Garbin, Mattiucci, Paoletti, González-Acuña and Nascetti2011; Violante-González et al., Reference Violante-González, Monks, Gil-Guerrero, Rojas-Herrera, Flores-Garza and Larumbe-Morán2011).
Other species of Contracaecum reported in the American continents have some similarities with the new species. Contracaecum margolisi Mattiucci, Cianchi, Nascetti, Paggi, Sardella, Timi, Webb, Bastida, Rodríguez & Bullini, 2003 found in Zalophus californianus from Canada; Contracaecum fagerholmi D'Amelio, Cavallero, Dronen, Barros and Paggi, 2012 and C. rudolphii D'Amelio Cavallero, Dronen, Barros and Paggi, 2012 found in Pelecanus occidentalis from Texas; Contracaecum bioccai Mattiucci, Paoletti, Olivero-Verbel, Baldiris, Arroyo-Salgado and Garbin, 2008 found in P. occidentalis from Colombia; and Contracaecum mirounga Nikolskiy, 1974 found in Spheniscus magellanicus from Argentina have the same number and arrangement of cephalic papillae as C. jorgei n. sp.. They also have distal tip of interlabia not bifurcated, except C. rudolphii F and C. bioccai. Contracaecum jorgei n. sp. most resembles C. mirounga due to body length (14.31–20.98 mm vs. 15.60–18.60 mm, respectively), distance from anterior end to nerve ring (0.29–0.46 mm vs. 0.35–0.43 mm) and size and shape of the tail (0.15–0.21 mm vs. 0.19–0.23 mm). However, C. jorgei n. sp. has much smaller spicules (right 2.28–3.63 mm; left 2.03–3.18 mm) than the other species: C. fagerholmi (spicules equal in length, 4.15–4.85 mm); C. rudolphii F (spicules equal in length 5.96–7.30 mm); C. bioccai (right 6.00–6.50 mm; left 5.80–6.20 mm); C. mirounga (right 8.12–10.71 mm; left 5.89–10.59 mm); and C. margolisi (right 6.10 mm; left 6.30 mm). The number of postcloacal papillae of C. jorgei n. sp. is greater than that of C. bioccai (six vs. four) and less than C. mirounga (six vs. 13 pairs), while C. fagerholmi and C. margolisi have the same number of postcloacal papillae and a single median papilla on the anterior cloaca rim (Mattiucci et al., Reference Mattiucci, Cianchi and Nascetti2003, Reference Mattiucci, Paoletti, Olivero-Verbel, Baldiris, Arroyo-Salgado, Garbin, Navone and Nascetti2008; D'Amelio et al., Reference D'Amelio, Cavallero, Dronen, Barros and Paggi2012; Garbin et al., Reference Garbin, Diaz and Navone2019).
The present species formed a well-supported clade with the sister group C. gibsoni Mattiucci, Paoletti, Solorzano and Nascetti, 2010 and C. overstreeti Mattiucci, Paoletti, Solorzano and Nascetti, 2010, but it is genetically and morphologically distinct. The sequences of C. gibsoni (EU852340) and C. overstreeti (EU852348) were obtained from adult worms recovered from Pelecanus crispus (L.) in Greece and C. overstreeti (MG495095) was obtained from larvae infecting Mugil cephalus in Turkey (Mattiucci et al., Reference Mattiucci, Paoletti, Solorzano and Nascetti2010; Pekmezci & Yardimci, Reference Pekmezci and Yardimci2019). The adults of C. gibsoni and C. overstreeti are larger than C. jorgei n. sp. (30.00–47.00 mm; 44.00–50.00 mm vs. 14.31–20.98), but they resemble C. jorgei n. sp. by the disposition and number of cephalic papillae and absence of bifurcated interlabia. These species differ from the new species by presenting smaller spicules (C. gibsoni: right 2.15–2.30 mm and left 2.10–2.30 mm; C. overstreeti: right 2.78–2.86 mm and left 2.90–3.15 mm vs. right 3.63–2.28 mm and left 3.18–2.03 mm) and the number and arrangement of caudal papillae. Contracaecum gibsoni has 65–68 postcloacal papillae, while C. overstreeti presents 61–65 postcloacal papillae. In addition, both species differ from the present species by having four pairs of proximal papillae posterior to the cloaca, a single pair of double paracloacal papillae, four pairs of distal papillae and a single pair of very small papilla-like phasmids.
The larvae of C. overstreeti differ from larvae of C. jorgei n. sp. by larger size (20.10–22.60 mm vs. 11.10–12.23 mm); greater length of the oesophagus (2.23–2.91 mm vs. 1.63–1.89 mm); ventricular appendix (0.78–0.82 mm vs. 0.30–0.37 mm); intestinal caecum (2.18–2.22 mm vs. 0.98–1.30 mm); and longer tail (0.22–0.23 mm vs. 0.11–0.13 mm). In addition, the tail of C. overstreeti larvae have sharply pointed ends with spines, distinguishing them from C. jorgei n. sp.
There are several records of L3 of Contracaecum in freshwater fish from the Atlantic region of South America, some of them including a description of the morphotypes (Moravec et al., Reference Moravec, Kohn and Fernandes1993; Martins et al., Reference Martins, Onaka and Fenerick2005; Luque et al., Reference Luque, Aguiar, Vieira, Gibson and Santos2011; Pinheiro et al., Reference Pinheiro, Furtado, Santos and Giese2019 in Brazil; and Mancini et al., Reference Mancini, Biolé, Salinas, Guagliardo, Tanzola and Morra2014 in Argentina). Moravec et al. (Reference Moravec, Kohn and Fernandes1993) described the larval type 1 of Contracaecum parasitizing Hoplias malabaricus in the Paraná River, Brazil, but this morphotype presents clear morphometric differences in relation to the new species (the total length, length of the oesophagus and length of the intestinal caecum are greater in the new species, while ventriculus length is shorter). Martins et al. (Reference Martins, Onaka and Fenerick2005) described larvae of Contracaecum parasitizing H. malabaricus from northern Brazil with morphometric characteristics similar to the larval type 2 described by Moravec et al. (Reference Moravec, Kohn and Fernandes1993). The L3 of the new species can be separated from the type 2 and the larvae described by Martins et al. (Reference Martins, Onaka and Fenerick2005) due to the shorter length of the body, oesophagus and intestinal caecum, while having a larger ventricle. The larvae of Contracaecum reported by Mancini et al. (Reference Mancini, Biolé, Salinas, Guagliardo, Tanzola and Morra2014) parasitizing nine freshwater fish species in Argentina, including H. malabaricus, were identified as type 2 (Moravec et al., Reference Moravec, Kohn and Fernandes1993), but the authors did not present morphometric information to allow comparison with C. jorgei n. sp. Unfortunately, there is no molecular information available about the larvae used in the morphological comparison, which could help to determine their specific identification.
The morphology of L4 of Contracaecum has not been described in detail until now. Torres et al. (Reference Torres, Valdivieso, Schlatter, Montefusco, Revenga, Marín, Lamilla and Ramallo2000) pointed to the difficulty of distinguishing L4 larvae from immature adults, but the latter could be recognized by the presence of caudal papillae, spicules in males and well-developed vulvae in females. In addition, the main feature to distinguish L4 from the adults is the absence of interlabia, as confirmed by morphological descriptions of L4 in other Contracaecum species (Kreis, Reference Kreis1955; McClelland & Ronald, Reference McClelland and Ronald1973; Semenova, Reference Semenova1974; Fagerholm et al., Reference Fagerholm, Overstreet and Humprey-Smith1996; Garbin et al., Reference Garbin, Navone, Diaz and Cremonte2007, Reference Garbin, Diaz, Cremonte and Navone2008; Kanarek & Bohdanowicz, Reference Kanarek and Bohdanowicz2009), in agreement with the characteristics observed in the L4 of the present study. According to Kanarek & Bohdanowicz (Reference Kanarek and Bohdanowicz2009), the lack of comprehensive analyses using molecular techniques to confirm the morphological criteria is a challenge to the identification of Contracaecum larvae. By integrating morphological and molecular studies of stages L3, L4 and adults isolated from a wide range of fish species (intermediate hosts) and birds and marine mammals (definitive hosts) will enable identifying specific larval stages of some species of Contracaecum.
There are only a few taxonomic descriptions of L3 and L4 of Contracaecum and studies using molecular techniques for larval identification, despite the high prevalence found in intermediate hosts in Argentina reported in recent years (Mancini et al., Reference Mancini, Biolé, Salinas, Guagliardo, Tanzola and Morra2014). Moreover, in the last 20 years, molecular genetic techniques have been important tools for species identification, mainly for larval stages of Anisakis, Pseudoterranova and Contracaecum, since morphological features are not enough to definitively distinguish species (Mattiucci & Nascetti, Reference Mattiucci and Nascetti2008; Mattiucci et al., Reference Mattiucci, Paoletti, Olivero-Verbel, Baldiris, Arroyo-Salgado, Garbin, Navone and Nascetti2008; Garbin et al., Reference Garbin, Mattiucci, Paoletti, Diaz, Nascetti and Navone2013).
In the life cycle of Contracaecum, eggs hatch and develop into second-stage larvae or L3 in water. These are ingested by aquatic arthropods and infect the body cavity of freshwater and marine fish when ingesting the infected aquatic arthropods (Dziekońska-Rynko & Rokicki, Reference Dziekońska-Rynko and Rokicki2007). It is likely that microcrustaceans and fish can act as intermediate hosts (Moravec, Reference Moravec2009); thus, the high availability of zooplankton and fish density recorded in shallow Pampean lakes in Argentina (Mancini & Grosman, Reference Mancini and Grosman2008) can increase the food supply for birds, enhancing the chance of infecting the definitive host. In the stomach of birds, L3 larvae develop to L4 and later become adults with sexual dimorphism (Køie & Fagerholm, Reference Køie and Fagerholm1995). Humans can accidentally be infected when consuming raw fish containing L3 of Contracaecum, as shown by Nagasawa (Reference Nagasawa2012) with the species Contracaecum osculatum. The larvae can penetrate the stomach and intestine walls, causing a life-threatening condition that causes stomach pains, fever, diarrhoea and vomiting (Dorny et al., Reference Dorny, Praet, Deckers and Gabriel2009). Moreover, it is known that nematodes, both larvae and adults, can negatively affect host health and cause serious losses to fisheries (Dick et al., Reference Dick, Papst and Paul1987). For that reason, an accurate species identification of Contracaecum infective larvae found in fish used for human consumption is very important (Kanarek & Bohdanowicz, Reference Kanarek and Bohdanowicz2009).
The use of molecular techniques is fundamental not only to define the taxonomic status of these species, but also for their recognition. Due to its higher rate of base change and the genetic variability observed in the mt cox-2 gene, this gene is larger than ribosomal genes (Ceballos-Mendiola et al., Reference Ceballos-Mendiola, Valero, Polo-Vico, Tejada, Abattouy, Karl, De Las Heras and Martín-Sánchez2010; Mattiucci et al., Reference Mattiucci, Cipriani, Webb, Paoletti, Marcer, Bellisario, Gibson and Nascetti2014) for species. The direct relationship between adults and larval stages of C. jorgei n. sp. and the interspecific variation found in this study show that mt cox-2 can be a valuable tool for species identification within the genus (Jex et al., Reference Jex, Waeschenbach, Littlewood, Hu and Gasser2008; Borges et al., Reference Borges, Santos, Brandão, Santos, Miranda, Balthazar, Luque and Santos2014). Therefore, due to the biodiversity of Anisakidae nematodes, the analysis of molecular genetic markers has been shown to be an efficient tool for specific diagnosis and unequivocal identification of anisakid nematodes with zoonotic potential (Kanarek & Bohdanowicz, Reference Kanarek and Bohdanowicz2009). This is an essential requirement for epidemiological surveys. Thus, the phylogenetic analyses indicate that C. jorgei n. sp. belongs to a lineage independent of the other species of Contracaecum, confirming it as a separate species.
Acknowledgments
Our thanks to Douglas McIntosh, Laboratory of Molecular Biology, Department of Animal Parasitology, UFRRJ, for technical support in sequencing, and to Jonathan Gonçalves de Oliveira for his help in discussing the molecular analysis data. We also thank the Rudolf Barth Electron Microscopy Platform (PDTIS/FIOCRUZ) for the support in scanning photographs, and Santiago Gimenez, Martín Garro and Fernando Amaya for their collaboration during collections.
Financial support
This study was supported partially by the Coordenação de Aperfeiçoamento de Pessoal de Nível Superior-Brasil (CAPES–Finance Code 001, grant number 88881.143550/2017-01) to CJS (‘Programa Centros Associados da Pós-Graduação Brasil-Argentina’). JLL was partially supported by Conselho Nacional de Desenvolvimento Científico e Tecnológico-Brasil (CNPq grant number 306952/2015-7).
Conflicts of interest
None.
Ethical standards
The authors declare that all applicable international, national and/or institutional guidelines for the care and use of animals were followed.