Ion transport is critical to the properties of nanofluidic devices such as ionic diodes and transistors. Ions naturally move along a concentration gradient from high to low concentrations. A research team led by Wei Guo of the Chinese Academy of Sciences has now demonstrated a counter example, where a piece of illuminated graphene oxide membrane (GOM) was shown to pump monovalent and divalent cations against a concentration gradient. This discovery was reported in a recent issue of Nature Communications (doi:10.1038/s41467-019-09178-x).
“Using light to control ion transport in fully synthetic membranes is the dream of a materials scientist, like me,” says Guo. Previously, anti-gradient ion transport, also called active ion transport, has mostly only been observed in biological systems (e.g., cell membranes). Fully abiotic systems were unable to attain active ion transport as efficiently as their biotic counterparts. This limitation drove Guo and co-workers to develop strategies rendering the active-ion-transport capability of abiotic systems.
The researchers focused attention on a two-dimensional (2D) photoelectric semiconductor—graphene oxide (GO) nanosheet. They first assembled GO nanosheets into a piece of membrane through vacuum filtration, and bridged two ion reservoirs with the GOM. The inter-sheet space in GO functioned as ion-transport channels between the two reservoirs. When the GOM was illuminated using a beam of visible light (100 mW cm–2, 30 s duration) at an off-center position, ionic current flowed from non-illuminated positions to the illuminated spot, a direction against the ion concentration gradient. The active ion transport rate approached ∼1 mol h–1 m–2, approximately five orders of magnitude faster than that of conventional diffusion.
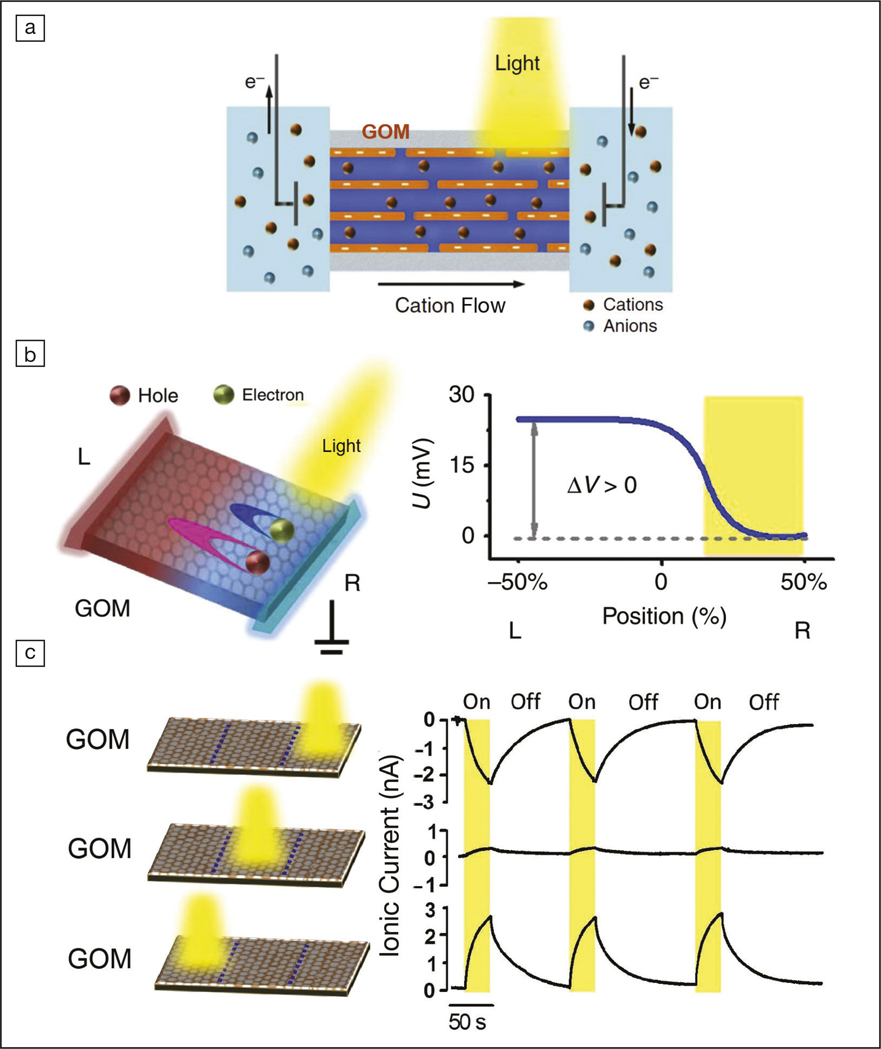
(a) The experimental setup. GOM: graphic oxide membrane. (b) The faster diffusion speed of holes than that of electrons in GO results in an electric potential difference (ΔV), which drives the active ion transport. (c) The illuminating positions on GOMs and the corresponding time traces of ionic current. Irradiating the GOMs at off-center positions leads to asymmetric charge distribution and triggers pronounced ionic current due to the active ion transport. Credit: Nature Communications.
The active-ion-transport process was associated with the photoelectronic properties of GO. Upon illumination, the GOM generated positively charged holes and negatively charged electrons at the irradiated spot. The photoexcited holes and electrons diffused to the non-illuminated parts in the membrane. Since holes moved faster than electrons in GO, the non-illuminated areas became relatively positive with respect to the illuminated area due to the accumulation of holes. This asymmetric charge distribution induced an electric potential difference. Once the driving force of the potential difference imposed on the cations became larger than the dragging force due to the concentration gradient, active ion transport could proceed.
Dan Li of The University of Melbourne, Australia, says, “This works demonstrates a novel strategy of coupling optical and electronic properties of GO to realize active ion transport in nanochannels, which opens up a new avenue for nanoionics and nanofluidics research.” Li was not involved in the research.
Guo’s team is now developing methods “to amplify the potential difference, as well as to scale up the membrane materials with, for example, printing techniques,” he says. Additionally, the researchers are expanding the materials toolbox for active ion transport by investigating 2D semiconductors beyond GO and combining them into heterostructures.